Toward robust and reproducible pluripotent stem cell expansion in bioprinted GelMA constructs
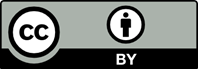
Combining the technologies of 3D bioprinting and human induced pluripotent stem cells (hiPSCs) has allowed for the creation of tissues with organ-level function in the lab, a promising technique for disease modeling and regenerative medicine. Expanding the stem cells in bioprinted tissues prior to differentiation allows for high cell density which is important for the formation of cell-cell junctions necessary for macroscale function upon differentiation. Yet, stem cell expansion, critical to successful in situ differentiation, depends heavily on the composition of the bioprinted scaffold. Here, we demonstrate how a common bioink component, gelatin methacryloyl (GelMA), varies depending on the vendor and degree of functionalization. We found that the vendor/GelMA production technique played a greater role in dictating the mechanical properties of the bioprinted constructs than the degree of functionalization, emphasizing the importance of reporting detailed characterization of GelMA scaffolds. Furthermore, the ability of singularized hiPSCs to survive and expand in GelMA scaffolds greatly varied across batches from different vendors and degrees of functionalization, where expansion correlated with the mechanical properties of the scaffold. Yet, we found that using a commercial cloning supplement could restore the ability of single hiPSCs to survive and expand across GelMA types, thus compensating for the varied mechanical properties of the scaffolds. These findings provide a practical guide for the expansion of hiPSCs in GelMA constructs with various mechanical properties as required for successful in situ differentiation.