Development of multi-layered 3D-printed scaffold for sequential delivery of biomolecules
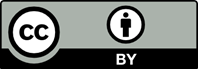
Spatiotemporal control of exogenous growth factors plays a crucial role in the sequential repair of damaged tissues. However, traditional therapeutic trials have mostly relied on the delivery of a single molecule due to limitations in rapid diffusion and the instability of biomolecules. In this study, we developed a novel strategy using a composite of gelatin and silica as an alternative for the stable and sequential release of biomolecules. Two biomolecules, basic fibroblast growth factor, and bone morphogenetic protein-2, were incorporated into two separate composites and sequentially layered onto the activated polymer surface. Each molecule was sequentially released from each layer of the scaffold, and the silica composite prevented rapid diffusion due to its nanoporous structure. The adhesion, proliferation, and osteogenic differentiation of rat bone marrow-derived mesenchymal stem cells were significantly enhanced in the double-layered group containing separately delivered dual molecules compared with the control or single groups. Our developed gelatin-silica composite was successfully incorporated in double layers on polymer scaffolds, and cellular responses were promoted in this manner. These results demonstrated that our scaffolding system has potential as a therapeutic strategy for the delivery of dual or multiple biomolecules in regenerative medicine.

- Hussey GS, Dziki JL, Badylak SF. Extracellular matrix-based materials for regenerative medicine. Nat Rev Mater. 2018;3(7):159-173. doi: 10.1038/s41578-018-0023-x
- Won JE, Lee YS, Park JH, et al. Hierarchical microchanneled scaffolds modulate multiple tissue-regenerative processes of immune-responses, angiogenesis, and stem cell homing. Biomaterials. 2020;227:119548. doi: 10.1016/j.biomaterials.2019.119548
- Babensee JE, McIntire LV, Mikos AG. Growth factor delivery for tissue engineering. Pharm Res. 2000;17(5):497-504. doi: 10.1023/a:1007502828372
- Wang ZM, Wang ZF, Lu WW, Zhen WX, Yang DZ, Peng SL. Novel biomaterial strategies for controlled growth factor delivery for biomedical applications. NPG Asia Mater. 2017;9:e435-451. doi: 10.1038/am.2017.171
- Yun YR, Jang JH, Jeon E, et al. Administration of growth factors for bone regeneration. Regen Med. 2012;7(3): 369-385. doi: 10.2217/rme.12.1
- Kang J, Park HM, Kim YW, et al. Control of mesenchymal stem cell phenotype and differentiation depending on cell adhesion mechanism. Eur Cell Mater. 2014;28:387-403. doi: 10.22203/ecm.v028a27
- Shah NJ, Hyder MN, Quadir MA, et al. Adaptive growth factor delivery from a polyelectrolyte coating promotes synergistic bone tissue repair and reconstruction. Proc Natl Acad Sci USA. 2014;111(35):12847-12852. doi: 10.1073/pnas.1408035111
- Kuttappan S, Mathew D, Jo JI, et al. Dual release of growth factor from nanocomposite fibrous scaffold promotes vascularisation and bone regeneration in rat critical sized calvarial defect. Acta Biomater. 2018;78:36-47. doi: 10.1016/j.actbio.2018.07.050
- Barnes GL, Kostenuik PJ, Gerstenfeld LC, Einhorn TA. Growth factor regulation of fracture repair. J Bone Miner Res. 1999;14(11):1805-1815. doi: 10.1359/jbmr.1999.14.11.1805
- Termaat MF, Den Boer FC, Bakker FC, Patka P, Haarman HJ. Bone morphogenetic proteins. Development and clinical efficacy in the treatment of fractures and bone defects. J Bone Joint Surg Am. 2005;87(6):1367-1378. doi: 10.2106/JBJS.D.02585
- Cochran DL, Jones AA, Lilly LC, Fiorellini JP, Howell H. Evaluation of recombinant human bone morphogenetic protein-2 in oral applications including the use of endosseous implants: 3-year results of a pilot study in humans. J Periodontol. 2000;71(8):1241-1257. doi: 10.1902/jop.2000.71.8.1241
- Epstein SE, Fuchs S, Zhou YF, Baffour R, Kornowski R. Therapeutic interventions for enhancing collateral development by administration of growth factors: basic principles, early results and potential hazards. Cardiovasc Res. 2001;49(3):532-542. doi: 10.1016/s0008-6363(00)00217-0
- Freeman FE, Pitacco P, van Dommelen LHA, et al. 3D bioprinting spatiotemporally defined patterns of growth factors to tightly control tissue regeneration. Sci Adv. 2020;6(33):eabb5093. doi: 10.1126/sciadv.abb5093
- Oliveira ER, Nie L, Podstawczyk D, et al. Advances in growth factor delivery for bone tissue engineering. Int J Mol Sci. 2021;22(2):903-935. doi: 10.3390/ijms22020903
- Nyberg E, Holmes C, Witham T, Grayson WL. Growth factor-eluting technologies for bone tissue engineering. Drug Deliv Transl Res. 2016;6(2):184-194. doi: 10.1007/s13346-015-0233-3
- Keceli HG, Bayram C, Celik E, Ercan N, Demirbilek M, Nohutcu RM. Dual delivery of platelet-derived growth factor and bone morphogenetic factor-6 on titanium surface to enhance the early period of implant osseointegration. J Periodontal Res. 2020;55(5):694-704. doi: 10.1111/jre.12756
- Won JE, Yun YR, Jang JH, et al. Multifunctional and stable bone mimic proteinaceous matrix for bone tissue engineering. Biomaterials. 2015;56:46-57. doi: 10.1016/j.biomaterials.2015.03.022
- Lee SJ, Won JE, Han C, et al. Development of a three-dimensionally printed scaffold grafted with bone forming peptide-1 for enhanced bone regeneration with in vitro and in vivo evaluations. J Colloid Interface Sci. 2019;539:468-480. doi: 10.1016/j.jcis.2018.12.097
- Heller M, Bauer HK, Goetze E, et al. Applications of patient-specific 3D printing in medicine. Int J Comput Dent. 2016;19(4):323-339.
- Laubach M, Suresh S, Herath B, et al. Clinical translation of a patient-specific scaffold-guided bone regeneration concept in four cases with large long bone defects. J Orthop Translat. 2022;34:73-84. doi: 10.1016/j.jot.2022.04.004
- Han HH, Shim JH, Lee H, et al. Reconstruction of complex maxillary defects using patient-specific 3D-printed biodegradable scaffolds. Plast Reconstr Surg Glob Open. 2018;6(11):e1975. doi: 10.1097/GOX.0000000000001975
- Hernandez I, Kumar A, Joddar B. A bioactive hydrogel and 3D printed polycaprolactone system for bone tissue engineering. Gels. 2017;3(3):26-38. doi: 10.3390/gels3030026
- Yu HS, Park J, Lee HS, Park SA, Lee DW, Park K. Feasibility of polycaprolactone scaffolds fabricated by three-dimensional printing for tissue engineering of tunica albuginea. World J Mens Health. 2018;36(1):66-72. doi: 10.5534/wjmh.17025
- Khandaker M, Riahinezhad S, Sultana F, Morris T, Wolf R, Vaughan M. Effect of collagen-polycaprolactone nanofibers matrix coating on the in vitro cytocompatibility and in vivo bone responses of titanium. J Med Biol Eng. 2018;38(2):197-210. doi: 10.1007/s40846-017-0312-7
- Burton TP, Corcoran A, Callanan A. The effect of electrospun polycaprolactone scaffold morphology on human kidney epithelial cells. Biomed Mater. 2017;13(1):015006. doi: 10.1088/1748-605X/aa8dde
- Won JE, Mateos-Timoneda MA, Castano O, et al. Fibronectin immobilization on to robotic-dispensed nanobioactive glass/polycaprolactone scaffolds for bone tissue engineering. Biotechnol Lett. 2015;37(4):935-942. doi: 10.1007/s10529-014-1745-5
- Dorj B, Won JE, Purevdorj O, et al. A novel therapeutic design of microporous-structured biopolymer scaffolds for drug loading and delivery. Acta Biomater. 2014;10(3):1238-1250. doi: 10.1016/j.actbio.2013.11.002
- Kirby GTS, White LJ, Rahman CV, et al. PLGA-based microparticles for the sustained release of BMP-2. Polymers- Basel. 2011;3(1):571-586. doi: 10.3390/polym3010571
- Bryans TR, Brawner VL, Quitevis EL. Microstructure and porosity of silica xerogel monoliths prepared by the fast sol-gel method. J Sol-Gel Sci Techn. 2000;17(3):211-217. doi: 10.1023/A:1008711921746
- Czarnobaj K. Preparation and characterization of silica xerogels as carriers for drugs. Drug Deliv. 2008;15(8):485-492. doi: 10.1080/10717540802321495
- Kortesuo P, Ahola M, Karlsson S, Kangasniemi I, Yli-Urpo A, Kiesvaara J. Silica xerogel as an implantable carrier for controlled drug delivery – evaluation of drug distribution and tissue effects after implantation. Biomaterials. 2000;21(2):193-198. doi: 10.1016/S0142-9612(99)00148-9
- Ahola M, Kortesuo P, Kangasniemi I, Kiesvaara J, Yli- Urpo A. Silica xerogel carrier material for controlled release of toremifene citrate. Int J Pharm. 2000;195(1-2): 219-227. doi: 10.1016/s0378-5173(99)00403-2
- Munusamy P, Seleem MN, Alqublan H, Tyler R, Sriranganathan N, Pickrell G. Targeted drug delivery using silica xerogel systems to treat diseases due to intracellular pathogens. Mat Sci Eng C-Mater. 2009;29(8):2313-2318. doi: 10.1016/j.msec.2009.05.020
- Simovic S, Ghouchi-Eskandar N, Sinn AM, Losic D, Prestidge CA. Silica materials in drug delivery applications. Curr Drug Discov Technol. 2011;8(3):269-276. doi: 10.2174/157016311796799026
- Xue JM, Tan CH, Lukito D. Biodegradable polymer-silica xerogel composite microspheres for controlled release of gentamicin. J Biomed Mater Res B Appl Biomater. 2006;78(2):417-422. doi: 10.1002/jbm.b.30503
- Lee EJ, Shin DS, Kim HE, Kim HW, Koh YH, Jang JH. Membrane of hybrid chitosan-silica xerogel for guided bone regeneration. Biomaterials. 2009;30(5):743-750. doi: 10.1016/j.biomaterials.2008.10.025
- Mahony O, Tsigkou O, Ionescu C, et al. Silica-gelatin hybrids with tailorable degradation and mechanical properties for tissue regeneration. Adv Funct Mater. 2010;20(22):3835-3845. doi: 10.1002/adfm.201000838
- Kim HW, Knowles JC, Kim HE. Hydroxyapatite and gelatin composite foams processed via novel freeze-drying and crosslinking for use as temporary hard tissue scaffolds. J Biomed Mater Res A. 2005;72(2):136-145. doi: 10.1002/jbm.a.30168
- Carpena NT, Min YK, Lee BT. Improved in vitro biocompatibility of surface-modified hydroxyapatite sponge scaffold with gelatin and BMP-2 in comparison against a commercial bone allograft. ASAIO J. 2015;61(1):78-86. doi: 10.1097/MAT.0000000000000155
- Fu A, Tang R, Hardie J, Farkas ME, Rotello VM. Promises and pitfalls of intracellular delivery of proteins. Bioconjug Chem. 2014;25(9):1602-1608. doi: 10.1021/bc500320j
- Zhou X, Weng W, Chen B, et al. Mesoporous silica nanoparticles/gelatin porous composite scaffolds with localized and sustained release of vancomycin for treatment of infected bone defects. J Mater Chem B. 2018;6(5):740-752. doi: 10.1039/c7tb01246b
- Uskokovic V, Ghosh S, Wu VM. Antimicrobial hydroxyapatite-gelatin-silica composite pastes with tunable setting properties. J Mater Chem B. 2017;5(30):6065-6080. doi: 10.1039/C7TB01794D
- Song JH, Kim HE, Kim HW. Collagen-apatite nanocomposite membranes for guided bone regeneration. J Biomed Mater Res B Appl Biomater. 2007;83(1):248-257. doi: 10.1002/jbm.b.30790
- Liu C, Li X, Zhao Q, et al. Nanofibrous bicomponent scaffolds for the dual delivery of NGF and GDNF: controlled release of growth factors and their biological effects. J Mater Sci Mater Med. 2021;32(1):9. doi: 10.1007/s10856-020-06479-2
- Gaydhane MK, Sharma CS, Majumdar S. Electrospun nanofibres in drug delivery: advances in controlled release strategies. RSC Adv. 2023;13(11):7312-7328. doi: 10.1039/d2ra06023j
- Houaoui A, Szczodra A, Lallukka M, et al. New generation of hybrid materials based on gelatin and bioactive glass particles for bone tissue regeneration. Biomolecules. 2021;11(3):444. doi: 10.3390/biom11030444
- Fujimoto Y, Yokozeki T, Yokoyama A, Tabata Y. Basic fibroblast growth factor enhances proliferation and hepatocyte growth factor expression of feline mesenchymal stem cells. Regen Ther. 2020;15:10-17. doi: 10.1016/j.reth.2020.03.013
- Visser R, Arrabal PM, Santos-Ruiz L, Becerra J, Cifuentes M. Basic fibroblast growth factor enhances the osteogenic differentiation induced by bone morphogenetic protein-6 in vitro and in vivo. Cytokine. 2012;58(1):27-33. doi: 10.1016/j.cyto.2011.12.020
- Lee H, Won DS, Park S, et al. 3D-printed versatile biliary stents with nanoengineered surface for anti-hyperplasia and antibiofilm formation. Bioact Mater. 2024;37:172-190. doi: 10.1016/j.bioactmat.2024.03.018