3D-printed polycaprolactone-magnetic nanoparticles composite multifunctional scaffolds for bone tissue regeneration and hyperthermia treatment
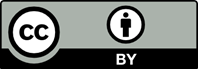
Cancer management after tumor resection is characterized by two critical needs: (i) rehabilitation of the resected tissue and (ii) preventing post-surgical tumor recurrence by destroying any residual tumors. The state-of-the-art in literature is limited because it tackles these two major priorities individually: achieving tissue regeneration via autologous grafting and preventing tumor recurrence through chemotherapy. In this paper, extrusion 3D printing has been employed to manufacture composite multi-functional scaffolds with polycaprolactone (PCL) as a polymeric matrix and magnetic nanoparticles (MNPs) in a 0–50 wt% ratio, which would aid in bone regeneration and cancer management via magnetic hyperthermia treatment. The fabricated scaffolds were evaluated for their mechanical, magnetic, and thermal characteristics. The Young’s modulus of the scaffolds increased multi-fold with increasing concentration of MNPs added to PCL (i.e., 26.88 ± 9.02 MPa for pure PCL to 229.06 ± 37.05 MPa for PCL/50 wt% MNP scaffolds). PCL/MNP scaffolds displayed a directly proportional correlation between MNP concentration and saturation magnetization. While the in vitro tests demonstrated a statistically significant cell growth of human mesenchymal stem cells (hMSCs) over 7 days for all MNP concentrations, only the 50% MNP scaffold exhibited an increase in temperature over 41oC when subjected to an alternating magnetic field, making it suitable for the hyperthermia treatment. On application of alternating magnetic fields to PCL and PCL/50 wt% MNP scaffolds, there was an increase in hMSCs proliferation and a decrease in bone cancer cell proliferation, thus validating the potential of the multi-functional scaffold for post-surgical bone cancer management.
- Kanwar S, Al-Ketan O, Vijayavenkataraman S. A novel method to design biomimetic, 3D printable stochastic scaffolds with controlled porosity for bone tissue engineering. Mater Design. 2022;220:110857. doi: 10.1016/j.matdes.2022.110857
- Liu JMH, Zhang J, Zhang X, et al. Transforming growth factor-beta 1 delivery from microporous scaffolds decreases inflammation post-implant and enhances function of transplanted islets. Biomaterials. 2016;80:11-19. doi: 10.1016/j.biomaterials.2015.11.065
- Llopis-Hernández V, Cantini M, González-García C, et al. Material-driven fibronectin assembly for high-efficiency presentation of growth factors. Sci Adv. 2016;2(8):e1600188. doi: 10.1126/sciadv.1600188
- Wan T, Stylios GK, Giannoudi M, Giannoudis PV. Investigating a new drug delivery nano composite membrane system based on PVA/PCL and PVA/HA(PEG) for the controlled release of biopharmaceuticals for bone infections. Injury. 2015;46:S39-S43. doi: 10.1016/s0020-1383(15)30053-x
- Chocholata P, Kulda V, Babuska V. Fabrication of scaffolds for bone-tissue regeneration. Materials. 2019;12(4):568. doi: 10.3390/jfb14070343
- Dong S, Chen Y, Yu L, Lin K, Wang X. Magnetic hyperthermia–synergistic H2O2 self-sufficient catalytic suppression of osteosarcoma with enhanced bone-regeneration bioactivity by 3D-printing composite scaffolds. Adv Funct Mater. 2020;30(4):1907071. doi: 10.1002/adfm.201907071
- Gu J, Liu X, Cui P, Yi X. Multifunctional bioactive glasses with spontaneous degradation for simultaneous osteosarcoma therapy and bone regeneration. Biomater Adv. 2023;154:213626. doi: 10.1016/j.bioadv.2023.213626
- Kiumarsi N, Najmoddin N. Systematically engineered GO with magnetic CuFe2O4 to enhance bone regeneration on 3D printed PCL scaffold. Surf Interf. 2023;39:102973. doi: 10.1016/j.surfin.2023.102973
- Giustini AJ, Petryk AA, Cassim SM, Tate JA, Baker I, Hoopes PJ. Magnetic nanoparticle hyperthermia in cancer treatment. Nano Life. 2010;1(1n02):10.1142/S1793984410000067. doi: 10.1142/S1793984410000067
- Sadeghzadeh H, Dianat-Moghadam H, Del Bakhshayesh AR, Mohammadnejad D, Mehdipour A. A review on the effect of nanocomposite scaffolds reinforced with magnetic nanoparticles in osteogenesis and healing of bone injuries. Stem Cell Res Ther. 2023;14(1):194. doi: 10.1186/s13287-023-03426-0
- Díaz E, Valle MB, Barandiarán JM. Magnetic composite scaffolds of polycaprolactone/nFeHA, for bone-tissue engineering. Int J Polym Mater Polym Biomater. 2016;65(12):593-600. doi: 10.1080/00914037.2016.1149848
- Cojocaru FD, Balan V, Popa MI, et al. Biopolymers - calcium phosphates composites with inclusions of magnetic nanoparticles for bone tissue engineering. Int J Biol Macromol. 2019;125:612-620. doi: 10.1016/j.ijbiomac.2018.12.083
- Li Y, Huang L, Tai G, et al. Graphene oxide-loaded magnetic nanoparticles within 3D hydrogel form high-performance scaffolds for bone regeneration and tumour treatment. Compos A: Appl Sci Manuf. 2022;152:106672. doi: 10.1016/j.compositesa.2021.106672
- Zhang Y, Zhang Y, Yang Z, et al. Cytotoxicity effect of iron oxide (Fe3O4)/graphene oxide (GO) nanosheets in cultured HBE cells. Front Chem. 2022;10:888033. doi: 10.3389/fchem.2022.888033
- Ali A, Shah T, Ullah R, et al. Review on recent progress in magnetic nanoparticles: synthesis, characterization, and diverse applications. Front Chem. 2021;9:629054. doi: 10.3389/fchem.2021.629054
- Obisesan OS, Ajiboye TO, Mhlanga SD, Mufhandu HT. Biomedical applications of biodegradable polycaprolactone-functionalized magnetic iron oxides nanoparticles and their polymer nanocomposites. Colloids Surf B Biointerfaces. 2023;227:113342. doi: 10.1016/j.colsurfb.2023.113342
- Shuai C, Yang W, He C, et al. A magnetic micro-environment in scaffolds for stimulating bone regeneration. Mater Design. 2020;185:108275. doi: 10.1016/j.matdes.2019.108275
- Ortolani A, Bianchi M, Mosca M, et al. The prospective opportunities offered by magnetic scaffolds for bone tissue engineering: a review. Joints. 2017;4(4):228-235. doi: 10.11138/jts/2016.4.4.228
- Gujjalapudi M, Anam C, Mamidi P, Chiluka R, Kumar AG, Bibinagar R. Effect of magnetic field on bone healing around endosseous implants - an in-vivo study. J Clin Diagn Res. 2016;10(10):ZF01-ZF4. doi: 10.7860/JCDR/2016/21509.8666
- Zhu Y, Yang Q, Yang M, et al. Protein corona of magnetic hydroxyapatite scaffold improves cell proliferation via activation of mitogen-activated protein kinase signaling pathway. ACS Nano. 2017;11(4):3690-3704. doi: 10.1021/acsnano.6b08193
- Wójcik-Piotrowicz K, Kaszuba-Zwoińska J, Rokita E, Thor P. Cell viability modulation through changes of Ca(2+)- dependent signalling pathways. Prog Biophys Mol Biol. 2016;121(1):45-53. doi: 10.1016/j.pbiomolbio.2016.01.004
- Wang Q, Chen B, Cao M, et al. Response of MAPK pathway to iron oxide nanoparticles in vitro treatment promotes osteogenic differentiation of hBMSCs. Biomaterials. 2016;86:11-20. doi: 10.1016/j.biomaterials.2016.02.004
- Hu S, Zhou Y, Zhao Y, et al. Enhanced bone regeneration and visual monitoring via superparamagnetic iron oxide nanoparticle scaffold in rats. J Tissue Eng Regen Med. 2018;12(4):e2085-e98. doi: 10.1002/term.2641
- Duarte A, Paola S-A, João CS, Frederico CF. 3D (bio)printing of magnetic hydrogels: formulation and applications in tissue engineering. Int J Bioprinting. 2024;10(1):0965. doi: 10.36922/ijb.0965
- Paun IA, Popescu RC, Calin BS, Mustaciosu CC, Dinescu M, Luculescu CR. 3D biomimetic magnetic structures for static magnetic field stimulation of osteogenesis. Int J Mol Sci. 2018;19(2):495. doi: 10.3390/ijms19020495
- Jia Y, Zhang P, Sun Y, et al. Regeneration of large bone defects using mesoporous silica coated magnetic nanoparticles during distraction osteogenesis. Nanomedicine. 2019;21:102040. doi: 10.1016/j.nano.2019.102040
- Wu D, Chang X, Tian J, et al. Bone mesenchymal stem cells stimulation by magnetic nanoparticles and a static magnetic field: release of exosomal miR-1260a improves osteogenesis and angiogenesis. J Nanobiotechnol. 2021;19(1):209. doi: 10.1186/s12951-021-00958-6
- Jasemi A, Kamyab Moghadas B, Khandan A, Saber- Samandari S. A porous calcium-zirconia scaffolds composed of magnetic nanoparticles for bone cancer treatment: fabrication, characterization and FEM analysis. Ceram Int. 2022;48(1):1314-1325. doi: 10.1016/j.ceramint.2021.09.216
- Zhao Y, Fan T, Chen J, et al. Magnetic bioinspired micro/ nanostructured composite scaffold for bone regeneration. Colloids Surf B Biointerfaces. 2019;174:70-79. doi: 10.1016/j.colsurfb.2018.11.003
- Zhang J, Zhao S, Zhu M, et al. 3D-printed magnetic Fe3O4/ MBG/PCL composite scaffolds with multifunctionality of bone regeneration, local anticancer drug delivery and hyperthermia. J Mater Chem B. 2014;2(43):7583-7595. doi: 10.1039/C4TB01063A
- Daňková J, Buzgo M, Vejpravová J, et al. Highly efficient mesenchymal stem cell proliferation on poly-ε-caprolactone nanofibers with embedded magnetic nanoparticles. Int J Nanomed. 2015;7(10):7307-7317. doi: 10.2147/ijn.s93670
- De Santis R, Russo A, Gloria A, et al. Towards the design of 3D fiber-deposited poly( -caprolactone)/iron-doped hydroxyapatite nanocomposite magnetic scaffolds for bone regeneration. J Biomed Nanotechnol. 2015;11(7):1236-1246. doi: 10.1166/jbn.2015.2065
- Rezaei V, Mirzaei E, Taghizadeh S-M, Berenjian A, Ebrahiminezhad A. Nano iron oxide-PCL composite as an improved soft tissue scaffold. Processes. 2021;9(9):1559. doi: 10.3390/pr9091559
- Cheah C, Chua C, Leong K, et al. Development of a tissue engineering scaffold structure library for rapid prototyping. Part 1: investigation and classification. Int J Adv Manuf Technol. 2003;21:291-301. doi: 10.1007/s001700300034
- Sato K, Watanabe Y, Horiuchi A, et al. Feasibility of new heating method of hepatic parenchyma using a sintered MgFe2O4 needle under an alternating magnetic field. J Surg Res. 2008;146(1):110-116. doi: 10.1016/j.jss.2007.05.022
- Newman D, Laredo E, Bello A, Grillo A, Feijoo JL, Müller AJ. Molecular mobilities in biodegradable poly(dl-lactide)/poly(ε-caprolactone) blends. Macromolecules. 2009;42(14):5219-5525. doi: 10.1021/ma9007303
- Galarreta-Rodriguez I, Lopez-Ortega A, Garayo E, et al. Magnetically activated 3D printable polylactic acid/ polycaprolactone/magnetite composites for magnetic induction heating generation. Adv Compos Hybrid Mater. 2023;6:102. doi: 10.1007/s42114-023-00687-4
- Atanasova N, Paunova-Krasteva T, Stoitsova S, et al. Degradation of poly(ε-caprolactone) by a thermophilic community and Brevibacillus thermoruber Strain 7 isolated from Bulgarian hot spring. Biomolecules. 2021;11(10):1488. doi: 10.3390/biom11101488
- Bin S, Wang A, Guo W, Yu L, Feng P. Micro magnetic field produced by Fe3O4 nanoparticles in bone scaffold for enhancing cellular activity. Polymers (Basel). 2020;12(9):2045. doi: 10.3390/polym12092045
- Mirkhalaf M, Men Y, Wang R, No Y, Zreiqat H. Personalized 3D printed bone scaffolds: a review. Acta Biomater. 2023;156:110-124. doi: 10.1016/j.actbio.2022.04.014
- Kanwar S, Vijayavenkataraman S. 3D printable bone-mimicking functionally gradient stochastic scaffolds for tissue engineering and bone implant applications. Mater Design. 2022;223:111199. doi: 10.1016/j.matdes.2022.111199
- Meenarathi B, Siva P, Palanikumar S, Kannammal L, Anbarasan R. Synthesis, characterization and drug release activity of poly(ε-caprolactone)/Fe3O4–alizarinred nanocomposites. Nanocomposites. 2016;2(2):98-107. doi: 10.1080/20550324.2016.1207009
- Saeed M, Beigi-Boroujeni S, Rajabi S, Ashteiani GR, Dolatfarahi M, Özcan M. A simple, green chemistry technology for fabrication of tissue-engineered scaffolds based on mussel-inspired 3D centrifugal spun. Mater Sci Eng C. 2021;121:111849. doi: 10.1016/j.msec.2020.111849
- Zeng X, Meng Z, Qiu Z, He J, Fan J, Li D. Melt-based embedded printing for freeform fabrication of overhanging and flexible polycaprolactone scaffolds. Virtual Phys Prototype. 2023;18(1):e2209778. doi: 10.1080/17452759.2023.2209778
- Haag H, Dalton PD, Bloemen V. The synergy of biomimetic design strategies for tissue constructs. Adv Funct Mater. 2022;32:2201414. doi: 10.1002/adfm.202201414
- Bakhtiary N, Pezeshki-Modaress M, Najmoddin N. Wet-electrospinning of nanofibrous magnetic composite 3-D scaffolds for enhanced stem cells neural differentiation. Chem Eng Sci. 2022;264:118144. doi: 10.1016/j.ces.2022.118144
- Lam T, Moy A, Lee HR, Shao Q, Bischof JC, Azarin SM. Iron oxide-loaded polymer scaffolds for non-invasive hyperthermic treatment of infiltrated cells. AIChE J. 2020;66(12):e17001. doi: 10.1002/aic.17001
- Shah RR, Davis TP, Glover AL, Nikles DE, Brazel CS. Impact of magnetic field parameters and iron oxide nanoparticle properties on heat generation for use in magnetic hyperthermia. J Magn Magn Mater. 2015;387:96-106. doi: 10.1016/j.jmmm.2015.03.085
- Xie W, Guo Z, Gao F, et al. Shape-, size- and structure-controlled synthesis and biocompatibility of iron oxide nanoparticles for magnetic theranostics. Theranostics. 2018;8(12):3284-3307. doi: 10.7150/thno.25220
- Urano M, Kuroda M, Nishimura Y. For the clinical application of thermochemotherapy given at mild temperatures. Int J Hyperthermia. 1999;15(2):79-107. doi: 10.1080/026567399285765
- Hildebrandt B, Wust P, Ahlers O, et al. The cellular and molecular basis of hyperthermia. Crit Rev Oncol Hematol. 2002;43(1):33-56. doi: 10.1016/S1040-8428(01)00179-2
- Freeman C, Halperin EC, Brady LW, David EW. Perez and Brady’s Principles and Practice of Radiation Oncology. Philadelphia: Wolters Kluwer Health/Lippincott Williams & Wilkins; 2008: 637-644. ISBN 978-0-7817-6369-1.
- Chew SA, Danti S. Biomaterial-based implantable devices for cancer therapy. Adv Healthc Mater. 2017;6(2):1600766. doi: 10.1002/adhm.201600766