3D bioprinting techniques and hydrogels for osteochondral integration regeneration
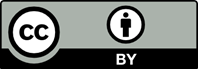
Despite considerable advancements in regenerative medicine, restoring the osteochondral interface and facilitating the integration of osteochondral regeneration remain significant clinical conundrums. This challenge is predominantly attributed to the scarcity of appropriate tissue engineering materials for replacing osteochondral defects and facilitating tissue regeneration. 3D bioprinting constitutes a promising approach for bone fabrication, as it not only allows for the design of precise personalized scaffolds but also encapsulates cells and growth factors, with the potential to replicate the functions of native tissues. Many critical properties of hydrogels, such as their mechanical properties, elasticity, and bioactivity, make them the most prevalently utilized bioinks in tissue engineering. In addition, their structure can be easily adjusted to meet the needs of different situations. Therefore, 3D-bioprinted hydrogel scaffolds may have promising prospects for integrated osteochondral repair and are receiving increasing attention. In this review, we describe the current problems encountered in the field of osteochondral integration repair and review the latest advances in current 3D printing technology and 3D bioprinting hydrogel scaffolds. We propose prospects for the development of novel 3D-bioprinted hydrogel scaffolds, providing cues for future research directions.

- Wu J, Kuang L, Chen C, et al. miR-100-5p-abundant exosomes derived from infrapatellar fat pad MSCs protect articular cartilage and ameliorate gait abnormalities via inhibition of mTOR in osteoarthritis. Biomaterials. 2019;206:87-100. doi: 10.1016/j.biomaterials.2019.03.022
- Lin W, Kluzek M, Iuster N, et al. Cartilage-inspired, lipid-based boundary-lubricated hydrogels. Science. 2020;370(6514):335-338. doi: 10.1126/science.aay8276
- Lesage C, Lafont M, Guihard P, Weiss P, Guicheux J, Delplace V. Material-assisted strategies for osteochondral defect repair. Adv Sci (Weinh). 2022;9(16):e2200050. doi: 10.1002/advs.202200050
- Zhou L, Gjvm VO, Malda J, et al. Innovative tissue-engineered strategies for osteochondral defect repair and regeneration: current progress and challenges. Adv Healthc Mater. 2020;9(23):e2001008. doi: 10.1002/adhm.202001008
- Deng C, Chang J, Wu C. Bioactive scaffolds for osteochondral regeneration. J Orthop Translat. 2019;17:15-25. doi: 10.1016/j.jot.2018.11.006
- Ansari S, Khorshidi S, Karkhaneh A. Engineering of gradient osteochondral tissue: from nature to lab. Acta Biomater. 2019;87:41-54. doi: 10.1016/j.actbio.2019.01.071
- Wang S, Zhao S, Yu J, Gu Z, Zhang Y. Advances in translational 3D printing for cartilage, bone, and osteochondral tissue engineering. Small. 2022;18(36):e2201869. doi: 10.1002/smll.202201869
- Wei W, Dai H. Articular cartilage and osteochondral tissue engineering techniques: recent advances and challenges. Bioact Mater. 2021;6(12):4830-4855. doi: 10.1016/j.bioactmat.2021.05.011
- Hu W, Chen Y, Dou C, Dong S. Microenvironment in subchondral bone: predominant regulator for the treatment of osteoarthritis. Ann Rheum Dis. 2021;80(4):413-422. doi: 10.1136/annrheumdis-2020-218089
- Zhang H, Wang L, Cui J, et al. Maintaining hypoxia environment of subchondral bone alleviates osteoarthritis progression. Sci Adv. 2023;9(14):eabo7868. doi: 10.1126/sciadv.abo7868
- Kang J, Li Y, Qin Y, et al. In situ deposition of drug and gene nanoparticles on a patterned supramolecular hydrogel to construct a directionally osteochondral plug. Nanomicro Lett. 2023;16(1):18. doi: 10.1007/s40820-023-01228-w
- Niu X, Li N, Du Z, Li X. Integrated gradient tissue-engineered osteochondral scaffolds: challenges, current efforts and future perspectives. Bioact Mater. 2023;20:574-597. doi: 10.1016/j.bioactmat.2022.06.011
- Li H, He Z, Li W, et al. Exploring the mechanism of microfracture in the treatment of porcine full-thickness cartilage defect. Am J Sports Med. 2023;51(4): 1033-1046. doi: 10.1177/03635465231153630
- Ghouri A, Muzumdar S, Barr AJ, et al. The relationship between meniscal pathologies, cartilage loss, joint replacement and pain in knee osteoarthritis: a systematic review. Osteoarthritis Cartilage. 2022;30(10):1287-1327. doi: 10.1016/j.joca.2022.08.002
- Kubosch EJ, Lang G, Furst D, et al. The potential for synovium-derived stem cells in cartilage repair. Curr Stem Cell Res Ther. 2018;13(3):174-184. doi: 10.2174/1574888x12666171002111026
- Schuette HB, Kraeutler MJ, Schrock JB, McCarty EC. Primary autologous chondrocyte implantation of the knee versus autologous chondrocyte implantation after failed marrow stimulation: a systematic review. Am J Sports Med. 2021;49(9):2536-2541. doi: 10.1177/0363546520968284
- Gilat R, Haunschild ED, Huddleston H, et al. Osteochondral allograft transplantation of the knee in adolescent patients and the effect of physeal closure. Arthroscopy. 2021;37(5):1588-1596. doi: 10.1016/j.arthro.2020.12.204
- Gao L, Orth P, Cucchiarini M, Madry H. Autologous matrix-induced chondrogenesis: a systematic review of the clinical evidence. Am J Sports Med. 2019;47(1):222-231. doi: 10.1177/0363546517740575
- Welch T, Mandelbaum B, Tom M. Autologous chondrocyte implantation: past, present, and future. Sports Med Arthrosc Rev. 2016;24(2):85-91. doi: 10.1097/jsa.0000000000000115
- Tuan RS. A second-generation autologous chondrocyte implantation approach to the treatment of focal articular cartilage defects. Arthritis Res Ther. 2007;9(5):109. doi: 10.1186/ar2310
- Niemeyer P, Köstler W, Salzmann GM, Lenz P, Kreuz PC, Südkamp NP. Autologous chondrocyte implantation for treatment of focal cartilage defects in patients age 40 years and older: a matched-pair analysis with 2-year follow-up. Am J Sports Med. 2010;38(12):2410-2416. doi: 10.1177/0363546510376742
- Saris DB, Vanlauwe J, Victor J, et al. Treatment of symptomatic cartilage defects of the knee: characterized chondrocyte implantation results in better clinical outcome at 36 months in a randomized trial compared to microfracture. Am J Sports Med. 2009;37(Suppl 1):10s-19s. doi: 10.1177/0363546509350694
- Jones KJ, Cash BM. Matrix-induced autologous chondrocyte implantation with autologous bone grafting for osteochondral lesions of the femoral trochlea. Arthrosc Tech. 2019;8(3):e259-e266. doi: 10.1016/j.eats.2018.10.022
- Nixon AJ, Sparks HD, Begum L, et al. Matrix-induced autologous chondrocyte implantation (MACI) using a cell-seeded collagen membrane improves cartilage healing in the equine model. J Bone Joint Surg Am. 2017;99(23):1987-1998. doi: 10.2106/jbjs.16.00603
- Richter DL, Tanksley JA, Miller MD. Osteochondral autograft transplantation: a review of the surgical technique and outcomes. Sports Med Arthrosc Rev. 2016;24(2):74-78. doi: 10.1097/jsa.0000000000000099
- Zouzias IC, Bugbee WD. Osteochondral allograft transplantation in the knee. Sports Med Arthrosc Rev. 2016;24(2):79-84. doi: 10.1097/jsa.0000000000000109
- Borodi PG, Russu OM, Feier AM, Georgeanu VA, Zuh SG, Pop TS. Is microfracture alone enough? Appl. Sci. 2021;11(16):7309. doi: 10.3390/app11167309
- Lee SS, Du X, Kim I, Ferguson SJ. Scaffolds for bone-tissue engineering. Matter. 2022;5(9):2722-2759. doi: 10.1016/j.matt.2022.06.003
- Bian Y, Hu T, Lv Z, et al. Bone tissue engineering for treating osteonecrosis of the femoral head. Exploration (Beijing). 2023;3(2):20210105. doi: 10.1002/exp.20210105
- Qin L, Yang S, Zhao C, et al. Prospects and challenges for the application of tissue engineering technologies in the treatment of bone infections. Bone Res. 2024;12(1):28. doi: 10.1038/s41413-024-00332-w
- Kwon H, Brown WE, Lee CA, et al. Surgical and tissue engineering strategies for articular cartilage and meniscus repair. Nat Rev Rheumatol. 2019;15(9):550-570. doi: 10.1038/s41584-019-0255-1
- Yang J, Zhang YS, Yue K, Khademhosseini A. Cell-laden hydrogels for osteochondral and cartilage tissue engineering. Acta Biomater. 2017;57:1-25. doi: 10.1016/j.actbio.2017.01.036
- Huang BJ, Hu JC, Athanasiou KA. Cell-based tissue engineering strategies used in the clinical repair of articular cartilage. Biomaterials. 2016;98:1-22. doi: 10.1016/j.biomaterials.2016.04.018
- Yang Z, Yi P, Liu Z, et al. Stem cell-laden hydrogel-based 3D bioprinting for bone and cartilage tissue engineering. Front Bioeng Biotechnol. 2022;10:865770. doi: 10.3389/fbioe.2022.865770
- Daly AC, Freeman FE, Gonzalez-Fernandez T, Critchley SE, Nulty J, Kelly DJ. 3D bioprinting for cartilage and osteochondral tissue engineering. Adv Healthc Mater. 2017;6(22):1700298. doi: 10.1002/adhm.201700298
- Zhang H, Wang M, Wu R, et al. From materials to clinical use: advances in 3D-printed scaffolds for cartilage tissue engineering. Phys Chem Chem Phys. 2023;25(36):24244-24263. doi: 10.1039/d3cp00921a
- Huey DJ, Hu JC, Athanasiou KA. Unlike bone, cartilage regeneration remains elusive. Science. 2012;338(6109):917-921. doi: 10.1126/science.1222454
- Mouser VHM, Levato R, Bonassar LJ, et al. Three-dimensional bioprinting and its potential in the field of articular cartilage regeneration. Cartilage. 2017;8(4):327-340. doi: 10.1177/1947603516665445
- Schon BS, Hooper GJ, Woodfield TB. Modular tissue assembly strategies for biofabrication of engineered cartilage. Ann Biomed Eng. 2017;45(1):100-114. doi: 10.1007/s10439-016-1609-3
- Jiang G, Li S, Yu K, et al. A 3D-printed PRP-GelMA hydrogel promotes osteochondral regeneration through M2 macrophage polarization in a rabbit model. Acta Biomater. 2021;128:150-162. doi: 10.1016/j.actbio.2021.04.010
- Ege D, Hasirci V. Is 3D printing promising for osteochondral tissue regeneration? ACS Appl Bio Mater. 2023;6(4):1431-1444. doi: 10.1021/acsabm.3c00093
- Visser J, Melchels FP, Jeon JE, et al. Reinforcement of hydrogels using three-dimensionally printed microfibres. Nat Commun. 2015;6:6933. doi: 10.1038/ncomms7933
- Baur E, Hirsch M, Amstad E. Porous 3D printable hydrogels. Adv Mater Technol. 2023;8(9):2201763. doi: 10.1002/admt.202201763
- Yue K, Trujillo-de Santiago G, Alvarez MM, Tamayol A, Annabi N, Khademhosseini A. Synthesis, properties, and biomedical applications of gelatin methacryloyl (GelMA) hydrogels. Biomaterials. 2015;73:254-271. doi: 10.1016/j.biomaterials.2015.08.045
- Geckil H, Xu F, Zhang X, Moon S, Demirci U. Engineering hydrogels as extracellular matrix mimics. Nanomedicine (Lond). 2010;5(3):469-484. doi: 10.2217/nnm.10.12
- Castilho M, Mouser V, Chen M, Malda J, Ito K. Bi-layered micro-fibre reinforced hydrogels for articular cartilage regeneration. Acta Biomater. 2019;95:297-306. doi: 10.1016/j.actbio.2019.06.030
- Liang Y, He J, Guo B. Functional hydrogels as wound dressing to enhance wound healing. ACS Nano. 2021;15(8):12687-12722. doi: 10.1021/acsnano.1c04206
- Olov N, Bagheri-Khoulenjani S, Mirzadeh H. Injectable hydrogels for bone and cartilage tissue engineering: a review. Prog Biomater. 2022;11(2):113-135. doi: 10.1007/s40204-022-00185-8
- Askari M, Afzali Naniz M, Kouhi M, Saberi A, Zolfagharian A, Bodaghi M. Recent progress in extrusion 3D bioprinting of hydrogel biomaterials for tissue regeneration: a comprehensive review with focus on advanced fabrication techniques. Biomater Sci. 2021;9(3):535-573. doi: 10.1039/d0bm00973c
- Elkhoury K, Morsink M, Sanchez-Gonzalez L, Kahn C, Tamayol A, Arab-Tehrany E. Biofabrication of natural hydrogels for cardiac, neural, and bone tissue engineering applications. Bioact Mater. 2021;6(11):3904-3923. doi: 10.1016/j.bioactmat.2021.03.040
- Singh YP, Moses JC, Bhardwaj N, Mandal BB. Injectable hydrogels: a new paradigm for osteochondral tissue engineering. J Mater Chem B. 2018;6(35):5499-5529. doi: 10.1039/c8tb01430b
- Lin X, Zhang L, Duan B. Polyphenol-mediated chitin self-assembly for constructing a fully naturally resourced hydrogel with high strength and toughness. Mater Horiz. 2021;8(9):2503-2512. doi: 10.1039/d1mh00878a
- Qin XH, Wang X, Rottmar M, Nelson BJ, Maniura-Weber K. Near-infrared light-sensitive polyvinyl alcohol hydrogel photoresist for spatiotemporal control of cell-instructive 3D microenvironments. Adv Mater. 2018;30(10):1705564. doi: 10.1002/adma.201705564
- Huang Q, Zou Y, Arno MC, et al. Hydrogel scaffolds for differentiation of adipose-derived stem cells. Chem Soc Rev. 2017;46(20):6255-6275. doi: 10.1039/c6cs00052e
- Beninatto R, Barbera C, De Lucchi O, et al. Photocrosslinked hydrogels from coumarin derivatives of hyaluronic acid for tissue engineering applications. Mater Sci Eng C Mater Biol Appl. 2019;96:625-634. doi: 10.1016/j.msec.2018.11.052
- Rosenquist J, Folkesson M, Höglund L, Pupkaite J, Hilborn J, Samanta A. An injectable, shape-retaining collagen hydrogel cross-linked using thiol-maleimide click chemistry for sealing corneal perforations. ACS Appl Mater Interfaces. 2023;15(29):34407-34418. doi: 10.1021/acsami.3c03963
- Wang Y, Wang J, Ji Z, et al. Application of bioprinting in ophthalmology. Int J Bioprint. 2022;8(2):552. doi: 10.18063/ijb.v8i2.552
- Kelly BE, Bhattacharya I, Heidari H, Shusteff M, Spadaccini CM, Taylor HK. Volumetric additive manufacturing via tomographic reconstruction. Science. 2019;363(6431):1075-1079. doi: 10.1126/science.aau7114
- He W, Deng J, Ma B, et al. Recent advancements of bioinks for 3D bioprinting of human tissues and organs. ACS Appl Bio Mater. 2024;7(1):17-43. doi: 10.1021/acsabm.3c00806
- Li X, Liu B, Pei B, et al. Inkjet bioprinting of biomaterials. Chem Rev. 2020;120(19):10793-10833. doi: 10.1021/acs.chemrev.0c00008
- Heinrich MA, Liu W, Jimenez A, et al. 3D bioprinting: from benches to translational applications. Small. 2019;15(23):e1805510. doi: 10.1002/smll.201805510
- Gudapati H, Dey M, Ozbolat I. A comprehensive review on droplet-based bioprinting: past, present and future. Biomaterials. 2016;102:20-42. doi: 10.1016/j.biomaterials.2016.06.012
- Matai I, Kaur G, Seyedsalehi A, McClinton A, Laurencin CT. Progress in 3D bioprinting technology for tissue/organ regenerative engineering. Biomaterials. 2020;226:119536. doi: 10.1016/j.biomaterials.2019.119536
- Saunders RE, Gough JE, Derby B. Delivery of human fibroblast cells by piezoelectric drop-on-demand inkjet printing. Biomaterials. 2008;29(2):193-203. doi: 10.1016/j.biomaterials.2007.09.032
- Zandrini T, Florczak S, Levato R, Ovsianikov A. Breaking the resolution limits of 3D bioprinting: future opportunities and present challenges. Trends Biotechnol. 2023;41(5):604-614. doi: 10.1016/j.tibtech.2022.10.009
- Mandrycky C, Wang Z, Kim K, Kim DH. 3D bioprinting for engineering complex tissues. Biotechnol Adv. 2016;34(4):422-434. doi: 10.1016/j.biotechadv.2015.12.011
- Lee JM, Sing SL, Zhou M, Yeong WY. 3D bioprinting processes: a perspective on classification and terminology. Int J Bioprint. 2018;4(2):151. doi: 10.18063/IJB.v4i2.151
- Lawlor KT, Vanslambrouck JM, Higgins JW, et al. Cellular extrusion bioprinting improves kidney organoid reproducibility and conformation. Nat Mater. 2021;20(2):260-271. doi: 10.1038/s41563-020-00853-9
- Ozbolat IT, Hospodiuk M. Current advances and future perspectives in extrusion-based bioprinting. Biomaterials. 2016;76:321-343. doi: 10.1016/j.biomaterials.2015.10.076
- Pati F, Jang J, Ha DH, et al. Printing three-dimensional tissue analogues with decellularized extracellular matrix bioink. Nat Commun. 2014;5:3935. doi: 10.1038/ncomms4935
- Mironov V, Trusk T, Kasyanov V, Little S, Swaja R, Markwald R. Biofabrication: a 21st century manufacturing paradigm. Biofabrication. 2009;1(2):022001. doi: 10.1088/1758-5082/1/2/022001
- Liu W, Heinrich MA, Zhou Y, et al. Extrusion bioprinting of shear-thinning gelatin methacryloyl bioinks. Adv Healthc Mater. 2017;6(12):1601451. doi: 10.1002/adhm.201601451
- Zandi N, Sani ES, Mostafavi E, et al. Nanoengineered shear-thinning and bioprintable hydrogel as a versatile platform for biomedical applications. Biomaterials. 2021; 267:120476. doi: 10.1016/j.biomaterials.2020.120476
- Loebel C, Rodell CB, Chen MH, Burdick JA. Shear-thinning and self-healing hydrogels as injectable therapeutics and for 3D-printing. Nat Protoc. 2017;12(8):1521-1541. doi: 10.1038/nprot.2017.053
- Xin S, Deo KA, Dai J, et al. Generalizing hydrogel microparticles into a new class of bioinks for extrusion bioprinting. Sci Adv. 2021;7(42):eabk3087. doi: 10.1126/sciadv.abk3087
- Liu W, Zhang YS, Heinrich MA, et al. Rapid continuous multimaterial extrusion bioprinting. Adv Mater. 2017;29(3):. doi: 10.1002/adma.201604630
- Kyle S, Jessop ZM, Al-Sabah A, Whitaker IS. ‘Printability’ of candidate biomaterials for extrusion based 3D printing: state-of-the-art. Adv Healthc Mater. 2017;6(16): 1604630. doi: 10.1002/adhm.201700264
- Kosorn W, Sakulsumbat M, Uppanan P, et al. PCL/PHBV blended three dimensional scaffolds fabricated by fused deposition modeling and responses of chondrocytes to the scaffolds. J Biomed Mater Res B Appl Biomater. 2017;105(5):1141-1150. doi: 10.1002/jbm.b.33658
- Winarso R, Anggoro PW, Ismail R, Jamari J, Bayuseno AP. Application of fused deposition modeling (FDM) on bone scaffold manufacturing process: a review. Heliyon. 2022;8(11):e11701. doi: 10.1016/j.heliyon.2022.e11701
- Turnbull G, Clarke J, Picard F, et al. 3D bioactive composite scaffolds for bone tissue engineering. Bioact Mater. 2018;3(3):278-314. doi: 10.1016/j.bioactmat.2017.10.001
- Park MJ, Bae J, Ju YK. Structural behavior of a composite curtain wall fabricated by the fused deposition modeling 3D printing method. Polymers (Basel). 2022;14(7):1431. doi: 10.3390/polym14071431
- Junqueira LA, Tabriz AG, Raposo FJ, et al. Coupling of fused deposition modeling and inkjet printing to produce drug loaded 3D printed tablets. Pharmaceutics. 2022;14(1). doi: 10.3390/pharmaceutics14010159
- Guillotin B, Souquet A, Catros S, et al. Laser assisted bioprinting of engineered tissue with high cell density and microscale organization. Biomaterials. 2010;31(28):7250-7256. doi: 10.1016/j.biomaterials.2010.05.055
- Mézel C, Souquet A, Hallo L, Guillemot F. Bioprinting by laser-induced forward transfer for tissue engineering applications: jet formation modeling. Biofabrication. 2010;2(1):014103. doi: 10.1088/1758-5082/2/1/014103
- Derakhshanfar S, Mbeleck R, Xu K, Zhang X, Zhong W, Xing M. 3D bioprinting for biomedical devices and tissue engineering: a review of recent trends and advances. Bioact Mater. 2018;3(2):144-156. doi: 10.1016/j.bioactmat.2017.11.008
- Dou C, Perez V, Qu J, Tsin A, Xu B, Li J. A state-of-the-art review of laser-assisted bioprinting and its future research trends. Chem Bio Eng Rev. 2021;8(5):517-534. doi: 10.1002/cben.202000037
- Cheptsov VS, Tsypina SI, Minaev NV, Yusupov VI, Chichkov BN. New microorganism isolation techniques with emphasis on laser printing. Int J Bioprint. 2019;5(1):165. doi: 10.18063/ijb.v5i1.165
- Bedell ML, Navara AM, Du Y, Zhang S, Mikos AG. Polymeric systems for bioprinting. Chem Rev. 2020;120(19):10744-10792. doi: 10.1021/acs.chemrev.9b00834
- Douillet C, Nicodeme M, Hermant L, et al. From local to global matrix organization by fibroblasts: a 4D laser-assisted bioprinting approach. Biofabrication. 2022;14(2):025006. doi: 10.1088/1758-5090/ac40ed
- Li J, Chen M, Fan X, Zhou H. Recent advances in bioprinting techniques: approaches, applications and future prospects. J Transl Med. 2016;14:271. doi: 10.1186/s12967-016-1028-0
- Murphy SV, Atala A. 3D bioprinting of tissues and organs. Nat Biotechnol. 2014;32(8):773-785. doi: 10.1038/nbt.2958
- Loterie D, Delrot P, Moser C. High-resolution tomographic volumetric additive manufacturing. Nat Commun. 2020;11(1):852. doi: 10.1038/s41467-020-14630-4
- Hochleitner G, Jüngst T, Brown TD, et al. Additive manufacturing of scaffolds with sub-micron filaments via melt electrospinning writing. Biofabrication. 2015;7(3):035002. doi: 10.1088/1758-5090/7/3/035002
- Li K, Wang C, Sun L, et al. Laser-assisted electrohydrodynamic jet printing of hierarchical nanostructure. Appl Therm Eng. 2024;253:123659. doi: 10.1016/j.applthermaleng.2024.123659
- Huang J, Qin Q, Wang J. A review of stereolithography: processes and systems. Processes. 2020;8(9):1138. doi: 10.3390/pr8091138
- Jana S, Lerman A. Bioprinting a cardiac valve. Biotechnol Adv. 2015;33(8):1503-1521. doi: 10.1016/j.biotechadv.2015.07.006
- Hinczewski C, Corbel S, Chartier T. Ceramic suspensions suitable for stereolithography. J Eur Ceram Soc. 1998;18(6):583-590. doi: 10.1016/S0955-2219(97)00186-6
- Gruene M, Deiwick A, Koch L, et al. Laser printing of stem cells for biofabrication of scaffold-free autologous grafts. Tissue Eng Part C Methods. 2011;17(1):79-87. doi: 10.1089/ten.TEC.2010.0359
- Ceballos-González CF, Bolívar-Monsalve EJ, Quevedo- Moreno DA, et al. High-throughput and continuous chaotic bioprinting of spatially controlled bacterial microcosms. ACS Biomater Sci Eng. 2021;7(6):2408-2419. doi: 10.1021/acsbiomaterials.0c01646
- Han Y, Jia B, Lian M, et al. High-precision, gelatin-based, hybrid, bilayer scaffolds using melt electro-writing to repair cartilage injury. Bioact Mater. 2021;6(7): 2173-2186. doi: 10.1016/j.bioactmat.2020.12.018
- Ng WL, Lee JM, Zhou M, et al. Vat polymerization-based bioprinting-process, materials, applications and regulatory challenges. Biofabrication. 2020;12(2):022001. doi: 10.1016/j.addma.2024.104189
- Chekkaramkodi D, Jacob L, C MS, Umer R, Butt H. Review of vat photopolymerization 3D printing of photonic devices. Addit Mfg. 2024;86:104189 doi: 10.1016/j.addma.2024.104189
- Daly AC, Prendergast ME, Hughes AJ, Burdick JA. Bioprinting for the biologist. Cell. 2021;184(1):18-32. doi: 10.1016/j.cell.2020.12.002
- Miri AK, Nieto D, Iglesias L, et al. Microfluidics-enabled multimaterial maskless stereolithographic bioprinting. Adv Mater. 2018;30(27):e1800242. doi: 10.1002/adma.201800242
- Creff J, Courson R, Mangeat T, et al. Fabrication of 3D scaffolds reproducing intestinal epithelium topography by high-resolution 3D stereolithography. Biomaterials. 2019;221:119404. doi: 10.1016/j.biomaterials.2019.119404
- Wang Z, Abdulla R, Parker B, Samanipour R, Ghosh S, Kim K. A simple and high-resolution stereolithography-based 3D bioprinting system using visible light crosslinkable bioinks. Biofabrication. 2015;7(4):045009. doi: 10.1088/1758-5090/7/4/045009
- Anandakrishnan N, Ye H, Guo Z, et al. Fast stereolithography printing of large-scale biocompatible hydrogel models. Adv Healthc Mater. 2021;10(10):e2002103. doi: 10.1002/adhm.202002103
- Walker DA, Hedrick JL, Mirkin CA. Rapid, large-volume, thermally controlled 3D printing using a mobile liquid interface. Science. 2019;366(6463):360-364. doi: 10.1126/science.aax1562
- Zhang AP, Qu X, Soman P, et al. Rapid fabrication of complex 3D extracellular microenvironments by dynamic optical projection stereolithography. Adv Mater. 2012;24(31):4266-4270. doi: 10.1002/adma.201202024
- Zhang Z, Yao S, Hu X, et al. Sacrificial synthesis of supported ru single atoms and clusters on N-doped carbon derived from covalent triazine frameworks: a charge modulation approach. Adv Sci (Weinh). 2021;8(3):2001493. doi: 10.1002/advs.202001493
- Li W, Wang M, Mille LS, et al. A smartphone-enabled portable digital light processing 3D printer. Adv Mater. 2021;33(35):e2102153. doi: 10.1002/adma.202102153
- Tumbleston JR, Shirvanyants D, Ermoshkin N, et al. Additive manufacturing. Continuous liquid interface production of 3D objects. Science. 2015;347(6228):1349-1352. doi: 10.1126/science.aaa2397
- Zhu W, Ma X, Gou M, Mei D, Zhang K, Chen S. 3D printing of functional biomaterials for tissue engineering. Curr Opin Biotechnol. 2016;40:103-112. doi: 10.1016/j.copbio.2016.03.014
- Wang M, Li W, Mille LS, et al. Digital light processing based bioprinting with composable gradients. Adv Mater. 2022;34(1):e2107038. doi: 10.1002/adma.202107038
- Xing JF, Zheng ML, Duan XM. Two-photon polymerization microfabrication of hydrogels: an advanced 3D printing technology for tissue engineering and drug delivery. Chem Soc Rev. 2015;44(15):5031-5039. doi: 10.1039/c5cs00278h
- Wang B, Engay E, Stubbe PR, et al. Stiffness control in dual color tomographic volumetric 3D printing. Nat Commun. 2022;13(1):367. doi: 10.1038/s41467-022-28013-4
- Bernal PN, Delrot P, Loterie D, et al. Volumetric bioprinting of complex living-tissue constructs within seconds. Adv Mater. 2019;31(42):e1904209. doi: 10.1002/adma.201904209
- Li H, Wang J, Yang G, Pei X, Zhang X. Advances of mussel-inspired hydrogels for bone/cartilage regeneration. Chem Eng J. 2024;487:150560. doi: 10.1016/j.cej.2024.150560
- Lee KY, Mooney DJ. Hydrogels for tissue engineering. Chem Rev. 2001;101(7):1869-1879. doi: 10.1021/cr000108x
- Trica B, Delattre C, Gros F, et al. Extraction and Characterization of alginate from an edible brown seaweed (cystoseira barbata) harvested in the Romanian black sea. Mar Drugs. 2019;17(7):405. doi: 10.3390/md17070405
- Ahmad Raus R, Wan Nawawi WMF, Nasaruddin RR. Alginate and alginate composites for biomedical applications. Asian J Pharm Sci. 2021;16(3):280-306. doi: 10.1016/j.ajps.2020.10.001
- Rastogi P, Kandasubramanian B. Review of alginate-based hydrogel bioprinting for application in tissue engineering. Biofabrication. 2019;11(4):042001. doi: 10.1088/1758-5090/ab331e
- Chawla D, Kaur T, Joshi A, Singh N. 3D bioprinted alginate-gelatin based scaffolds for soft tissue engineering. Int J Biol Macromol. 2020;144:560-567. doi: 10.1016/j.ijbiomac.2019.12.127
- Kulanthaivel S, Rathnam VSS, Agarwal T, et al. Gum tragacanth-alginate beads as proangiogenic-osteogenic cell encapsulation systems for bone tissue engineering. J Mater Chem B. 2017;5(22):4177-4189. doi: 10.1039/c7tb00390k
- Tiwari S, Patil R, Bahadur P. Polysaccharide based scaffolds for soft tissue engineering applications. Polymers (Basel). 2018;11(1). doi: 10.3390/polym11010001
- Sarker B, Rompf J, Silva R, et al. Alginate-based hydrogels with improved adhesive properties for cell encapsulation. Int J Biol Macromol. 2015;78:72-78. doi: 10.1016/j.ijbiomac.2015.03.061
- Diniz IM, Chen C, Ansari S, et al. Gingival mesenchymal stem cell (GMSC) delivery system based on RGD-coupled alginate hydrogel with antimicrobial properties: a novel treatment modality for peri-implantitis. J Prosthodont. 2016;25(2):105-115. doi: 10.1111/jopr.12316
- Endo K, Anada T, Yamada M, Seki M, Sasaki K, Suzuki O. Enhancement of osteoblastic differentiation in alginate gel beads with bioactive octacalcium phosphate particles. Biomed Mater. 2015;10(6):065019. doi: 10.1088/1748-6041/10/6/065019
- Popa EG, Gomes ME, Reis RL. Cell delivery systems using alginate–carrageenan hydrogel beads and fibers for regenerative medicine applications. Biomacromolecules. 2011;12(11):3952-3961. doi: 10.1021/bm200965x
- Zeng H, Chen Z, Wei P, Huang H, Liu B, Fan Z. Rapid customization of biomimetic cartilage scaffold with stem cell capturing and homing capabilities for in situ inductive regeneration of osteochondral defects. Adv. Funct. Mater. 2024;34(28):2400608. doi: 10.1002/adfm.202400608
- Klotz BJ, Gawlitta D, Rosenberg A, Malda J, Melchels FPW. Gelatin-methacryloyl hydrogels: towards biofabrication-based tissue repair. Trends Biotechnol. 2016;34(5):394-407. doi: 10.1016/j.tibtech.2016.01.002
- Bellis SL. Advantages of RGD peptides for directing cell association with biomaterials. Biomaterials. 2011;32(18):4205-4210. doi: 10.1016/j.biomaterials.2011.02.029
- Samorezov JE, Headley EB, Everett CR, Alsberg E. Sustained presentation of BMP-2 enhances osteogenic differentiation of human adipose-derived stem cells in gelatin hydrogels. J Biomed Mater Res A. 2016;104(6):1387-1397. doi: 10.1002/jbm.a.35668
- Liu J, Li L, Suo H, Yan M, Yin J, Fu J. 3D printing of biomimetic multi-layered GelMA/nHA scaffold for osteochondral defect repair. Mater Des. 2019;171:107708. doi: 10.1016/j.matdes.2019.107708
- Dong Y, Zhang M, Han D, et al. A high-performance GelMA-GelMA homogeneous double-network hydrogel assisted by 3D printing. J Mater Chem B. 2022;10(20):3906-3915. doi: 10.1039/d2tb00330a
- Rajabi M, McConnell M, Cabral J, Ali MA. Chitosan hydrogels in 3D printing for biomedical applications. Carbohydr Polym. 2021;260:117768. doi: 10.1016/j.carbpol.2021.117768
- Choi B, Kim S, Lin B, Wu BM, Lee M. Cartilaginous extracellular matrix-modified chitosan hydrogels for cartilage tissue engineering. ACS Appl Mater Interfaces. 2014;6(22):20110-20121. doi: 10.1021/am505723k
- Sheehy EJ, Mesallati T, Vinardell T, Kelly DJ. Engineering cartilage or endochondral bone: a comparison of different naturally derived hydrogels. Acta Biomater. 2015;13:245-253. doi: 10.1016/j.actbio.2014.11.031
- Fu J, Yang F, Guo Z. The chitosan hydrogels: from structure to function. New J Chem. 2018;42(21):17162-17180. doi: 10.1039/C8NJ03482F
- Mourya VK, Inamdar NN. Trimethyl chitosan and its applications in drug delivery. J Mater Sci Mater Med. 2009;20(5):1057-1079. doi: 10.1007/s10856-008-3659-z
- Waibel KH, Haney B, Moore M, Whisman B, Gomez R. Safety of chitosan bandages in shellfish allergic patients. Mil Med. 2011;176(10):1153-1156. doi: 10.7205/milmed-d-11-00150
- Maloney FP, Kuklewicz J, Corey RA, et al. Structure, substrate recognition and initiation of hyaluronan synthase. Nature. 2022;604(7904):195-201. doi: 10.1038/s41586-022-04534-2
- Zamboni F, Wong CK, Collins MN. Hyaluronic acid association with bacterial, fungal and viral infections: can hyaluronic acid be used as an antimicrobial polymer for biomedical and pharmaceutical applications? Bioact Mater. 2023;19:458-473. doi: 10.1016/j.bioactmat.2022.04.023
- Ding YW, Wang ZY, Ren ZW, Zhang XW, Wei DX. Advances in modified hyaluronic acid-based hydrogels for skin wound healing. Biomater Sci. 2022;10(13):3393-3409. doi: 10.1039/d2bm00397j
- Yang X, Wang B, Peng D, et al. Hyaluronic acid-based injectable hydrogels for wound dressing and localized tumor therapy: a review. Adv Nanobiomed Res. 2022;2(12):2200124. doi: 10.1002/anbr.202200124
- Shebl RI, Amer ME, Abuamara TMM, et al. Staphylococcus aureus derived hyaluronic acid and bacillus Calmette- Guérin purified proteins as immune enhancers to rabies vaccine and related immuno-histopathological alterations. Clin Exp Vaccine Res. 2021;10(3):229-239. doi: 10.7774/cevr.2021.10.3.229
- Trombino S, Servidio C, Curcio F, Cassano R. Strategies for hyaluronic acid-based hydrogel design in drug delivery. Pharmaceutics. 2019;11(8):407. doi: 10.3390/pharmaceutics11080407
- Kotla NG, Mohd Isa IL, Larrañaga A, et al. Hyaluronic acid-based bioconjugate systems, scaffolds, and their therapeutic potential. Adv Healthc Mater. 2023;12(20):e2203104. doi: 10.1002/adhm.202203104
- Schuurmans CCL, Mihajlovic M, Hiemstra C, Ito K, Hennink WE, Vermonden T. Hyaluronic acid and chondroitin sulfate (meth)acrylate-based hydrogels for tissue engineering: synthesis, characteristics and pre-clinical evaluation. Biomaterials. 2021;268:120602. doi: 10.1016/j.biomaterials.2020.120602
- An C, Li H, Zhao Y, et al. Hyaluronic acid-based multifunctional carriers for applications in regenerative medicine: a review. Int J Biol Macromol. 2023;231:123307. doi: 10.1016/j.ijbiomac.2023.123307
- Pandit AH, Mazumdar N, Ahmad S. Periodate oxidized hyaluronic acid-based hydrogel scaffolds for tissue engineering applications. Int J Biol Macromol. 2019;137:853-869. doi: 10.1016/j.ijbiomac.2019.07.014
- Zhou D, Li S, Pei M, et al. Dopamine-modified hyaluronic acid hydrogel adhesives with fast-forming and high tissue adhesion. ACS Appl Mater Interfaces. 2020;12(16):18225-18234. doi: 10.1021/acsami.9b22120
- Zhou M, Wei W, Chen X, Xu X, Zhang X, Zhang X. pH and redox dual responsive carrier-free anticancer drug nanoparticles for targeted delivery and synergistic therapy. Nanomedicine. 2019;20:102008. doi: 10.1016/j.nano.2019.04.011
- Palmese LL, Thapa RK, Sullivan MO, Kiick KL. Hybrid hydrogels for biomedical applications. Curr Opin Chem Eng. 2019;24:143-157. doi: 10.1016/j.coche.2019.02.010
- Wang H, Xu Y, Wang P, et al. Cell-mediated injectable blend hydrogel-BCP ceramic scaffold for in situ condylar osteochondral repair. Acta Biomater. 2021;123:364-378. doi: 10.1016/j.actbio.2020.12.056
- Seol YJ, Park JY, Jeong W, Kim TH, Kim SY, Cho DW. Development of hybrid scaffolds using ceramic and hydrogel for articular cartilage tissue regeneration. J Biomed Mater Res A. 2015;103(4):1404-1413. doi: 10.1002/jbm.a.35276
- Li J, Zhi W, Xu T, et al. Ectopic osteogenesis and angiogenesis regulated by porous architecture of hydroxyapatite scaffolds with similar interconnecting structure in vivo. Regen Biomater. 2016;3(5):285-297. doi: 10.1093/rb/rbw031
- You F, Chen X, Cooper DML, Chang T, Eames BF. Homogeneous hydroxyapatite/alginate composite hydrogel promotes calcified cartilage matrix deposition with potential for three-dimensional bioprinting. Biofabrication. 2018;11(1):015015. doi: 10.1088/1758-5090/aaf44a
- Wang Y, Wu S, Kuss MA, Streubel PN, Duan B. Effects of hydroxyapatite and hypoxia on chondrogenesis and hypertrophy in 3D bioprinted ADMSC laden constructs. ACS Biomater Sci Eng. 2017;3(5):826-835. doi: 10.1021/acsbiomaterials.7b00101
- Kosik-Kozioł A, Costantini M, Mróz A, et al. 3D bioprinted hydrogel model incorporating β-tricalcium phosphate for calcified cartilage tissue engineering. Biofabrication. 2019;11(3):035016. doi: 10.1088/1758-5090/ab15cb
- Wang W, Shen J, Meng Y, et al. Magnesium cationic cue enriched interfacial tissue microenvironment nurtures the osseointegration of gamma-irradiated allograft bone. Bioact Mater. 2022;10:32-47. doi: 10.1016/j.bioactmat.2021.08.027
- Chen Y, Chen Y, Xiong X, et al. Hybridizing gellan/ alginate and thixotropic magnesium phosphate-based hydrogel scaffolds for enhanced osteochondral repair. Mater Today Bio. 2022;14:100261. doi: 10.1016/j.mtbio.2022.100261
- Gao F, Xu Z, Liang Q, et al. Osteochondral regeneration with 3D-printed biodegradable high-strength supramolecular polymer reinforced-gelatin hydrogel scaffolds. Adv Sci (Weinh). 2019;6(15):1900867. doi: 10.1002/advs.201900867
- Dong L, Han Z, Li X. Tannic acid-mediated multifunctional 3D printed composite hydrogel for osteochondral regeneration. Int J Bioprint. 2022;8(3):587. doi: 10.18063/ijb.v8i3.587
- Antich C, de Vicente J, Jiménez G, et al. Bio-inspired hydrogel composed of hyaluronic acid and alginate as a potential bioink for 3D bioprinting of articular cartilage engineering constructs. Acta Biomater. 2020;106: 114-123. doi: 10.1016/j.actbio.2020.01.046
- Agostinacchio F, Mu X, Dirè S, Motta A, Kaplan DL. In situ 3D printing: opportunities with silk inks. Trends Biotechnol. 2021;39(7):719-730. doi: 10.1016/j.tibtech.2020.11.003
- Ma K, Zhao T, Yang L, et al. Application of robotic-assisted in situ 3D printing in cartilage regeneration with HAMA hydrogel: an in vivo study. J Adv Res. 2020;23:123-132. doi: 10.1016/j.jare.2020.01.010
- Motloung MP, Mofokeng TG, Ray SS. Viscoelastic, thermal, and mechanical properties of melt-processed poly (ε-Caprolactone) (PCL)/hydroxyapatite (HAP) composites. Materials (Basel). 2021;15(1). doi: 10.3390/ma15010104
- Rahmani S, Maroufkhani M, Mohammadzadeh-Komuleh S, Khoubi-Arani Z. Chapter 7 – polymer nanocomposites for biomedical applications. In: Barhoum A, Jeevanandam J, Danquah MK, eds. Fundamentals of Bionanomaterials. Elsevier; 2022:175-215. doi: 10.1016/B978-0-12-824147-9.00007-8
- Chen H, Gonnella G, Huang J, Di-Silvio L. Fabrication of 3D bioprinted bi-phasic scaffold for bone-cartilage interface regeneration. Biomimetics (Basel). 2023;8(1):87. doi: 10.3390/biomimetics8010087
- Wang S, Gu R, Wang F, et al. 3D-printed PCL/Zn scaffolds for bone regeneration with a dose-dependent effect on osteogenesis and osteoclastogenesis. Mater Today Bio. 2022;13:100202. doi: 10.1016/j.mtbio.2021.100202
- Tabatabaei F, Gelin A, Rasoulianboroujeni M, Tayebi L. Coating of 3D printed PCL/TCP scaffolds using homogenized-fibrillated collagen. Colloids Surf B Biointerfaces. 2022;217:112670. doi: 10.1016/j.colsurfb.2022.112670
- Thakkar S, Ghebes CA, Ahmed M, et al. Mesenchymal stromal cell-derived extracellular matrix influences gene expression of chondrocytes. Biofabrication. 2013;5(2):025003. doi: 10.1088/1758-5082/5/2/025003
- Li C, Zhang W, Nie Y, et al. Integrated and bifunctional bilayer 3D printing scaffold for osteochondral defect repair. Adv Funct Mater. 2023;33(20):2214158. doi: 10.1002/adfm.202214158
- Chen C, Zhang QW, Ye Y, Lin LG. Honokiol: a naturally occurring lignan with pleiotropic bioactivities. Chin J Nat Med. 2021;19(7):481-490. doi: 10.1016/S1875-5364(21)60047-X
- Zhu S, Chen P, Chen Y, Li M, Chen C, Lu H. 3D-printed extracellular matrix/polyethylene glycol diacrylate hydrogel incorporating the anti-inflammatory phytomolecule honokiol for regeneration of osteochondral defects. Am J Sports Med. 2020;48(11):2808-2818. doi: 10.1177/0363546520941842
- Liu J, Gao J, Liang Z, et al. Mesenchymal stem cells and their microenvironment. Stem Cell Res Ther. 2022;13(1):429. doi: 10.1186/s13287-022-02985-y
- Shim JH, Jang KM, Hahn SK, et al. Three-dimensional bioprinting of multilayered constructs containing human mesenchymal stromal cells for osteochondral tissue regeneration in the rabbit knee joint. Biofabrication. 2016;8(1):014102. doi: 10.1088/1758-5090/8/1/014102
- Gao G, Schilling AF, Hubbell K, et al. Improved properties of bone and cartilage tissue from 3D inkjet-bioprinted human mesenchymal stem cells by simultaneous deposition and photocrosslinking in PEG-GelMA. Biotechnol Lett. 2015;37(11):2349-2355. doi: 10.1007/s10529-015-1921-2
- Zhang H, Huang H, Hao G, et al. 3D printing hydrogel scaffolds with nanohydroxyapatite gradient to effectively repair osteochondral defects in rats. Adv Funct Mater. 2021;31(1):2006697. doi: 10.1002/adfm.202006697
- Reesink HL, Sutton RM, Shurer CR, et al. Galectin-1 and galectin-3 expression in equine mesenchymal stromal cells (MSCs), synovial fibroblasts and chondrocytes, and the effect of inflammation on MSC motility. Stem Cell Res Ther. 2017;8(1). doi: 10.1186/s13287-017-0691-2
- Loeser RF, Goldring SR, Scanzello CR, Goldring MB. Osteoarthritis: a disease of the joint as an organ. Arthritis Rheum. 2012;64(6):1697-1707. doi: 10.1002/art.34453
- Liu Y, Peng L, Li L, et al. 3D-bioprinted BMSC-laden biomimetic multiphasic scaffolds for efficient repair of osteochondral defects in an osteoarthritic rat model. Biomaterials. 2021;279:121216. doi: 10.1016/j.biomaterials.2021.121216
- Daly AC, Pitacco P, Nulty J, Cunniffe GM, Kelly DJ. 3D printed microchannel networks to direct vascularisation during endochondral bone repair. Biomaterials. 2018;162:34-46. doi: 10.1016/j.biomaterials.2018.01.057
- Galle J, Hoffmann M, Aust G. From single cells to tissue architecture-a bottom-up approach to modelling the spatio-temporal organisation of complex multi-cellular systems. J Mathematical Biology. 2009;58(1-2):261-283. doi: 10.1007/s00285-008-0172-4
- Zhuang P, Sun AX, An J, Chua CK, Chew SY. 3D neural tissue models: from spheroids to bioprinting. Biomaterials. 2018;154:113-133. doi: 10.1016/j.biomaterials.2017.10.002
- Lee EJ, Park SJ, Kang SK, et al. Spherical bullet formation via E-cadherin promotes therapeutic potency of mesenchymal stem cells derived from human umbilical cord blood for myocardial infarction. Mol Ther. 2012;20(7):1424-1433. doi: 10.1038/mt.2012.58
- Fennema E, Rivron N, Rouwkema J, van Blitterswijk C, De Boer J. Spheroid culture as a tool for creating 3D complex tissues. Trends Biotechnol. 2013;31(2):108-115. doi: 10.1016/j.tibtech.2012.12.003
- Zhang J, Xin W, Qin Y, et al. “All-in-one” zwitterionic granular hydrogel bioink for stem cell spheroids production and 3D bioprinting. Chem Eng J. 2022;430:132713. doi: 10.1016/j.cej.2021.132713
- Kim K, Yeatts A, Dean D, Fisher JP. Stereolithographic bone scaffold design parameters: osteogenic differentiation and signal expression. Tissue Eng Part B Rev. 2010;16(5):523-539. doi: 10.1089/ten.TEB.2010.0171
- Karageorgiou V, Kaplan D. Porosity of 3D biomaterial scaffolds and osteogenesis. Biomaterials. 2005;26(27):5474-5491. doi: 10.1016/j.biomaterials.2005.02.002
- Nowicki MA, Castro NJ, Plesniak MW, Zhang LG. 3D printing of novel osteochondral scaffolds with graded microstructure. Nanotechnology. 2016;27(41):414001. doi: 10.1088/0957-4484/27/41/414001
- Peng Y, Zhuang Y, Liu Y, et al. Bioinspired gradient scaffolds for osteochondral tissue engineering. Exploration (Beijing). 2023;3(4):20210043. doi: 10.1002/exp.20210043
- Mironov V, Visconti RP, Kasyanov V, Forgacs G, Drake CJ, Markwald RR. Organ printing: tissue spheroids as building blocks. Biomaterials. 2009;30(12):2164-2174. doi: 10.1016/j.biomaterials.2008.12.084
- Diloksumpan P, de Ruijter M, Castilho M, et al. Combining multi-scale 3D printing technologies to engineer reinforced hydrogel-ceramic interfaces. Biofabrication. 2020;12(2):025014. doi: 10.1088/1758-5090/ab69d9
- Zhai X, Ruan C, Ma Y, et al. 3D-bioprinted osteoblast-laden nanocomposite hydrogel constructs with induced microenvironments promote cell viability, differentiation, and osteogenesis both in vitro and in vivo. Adv Sci (Weinh). 2018;5(3):1700550. doi: 10.1002/advs.201700550
- Park JY, Choi JC, Shim JH, et al. A comparative study on collagen type I and hyaluronic acid dependent cell behavior for osteochondral tissue bioprinting. Biofabrication. 2014;6(3):035004. doi: 10.1088/1758-5082/6/3/035004
- Critchley S, Sheehy EJ, Cunniffe G, et al. 3D printing of fibre-reinforced cartilaginous templates for the regeneration of osteochondral defects. Acta Biomater. 2020; 113:130-143. doi: 10.1016/j.actbio.2020.05.040
- Zhang Y, Li D, Liu Y, et al. 3D-bioprinted anisotropic bicellular living hydrogels boost osteochondral regeneration via reconstruction of cartilage-bone interface. Innovation (Camb). 2024;5(1):100542. doi: 10.1016/j.xinn.2023.100542
- Wu M, Wu S, Chen W, Li Y-P. The roles and regulatory mechanisms of TGF-β and BMP signaling in bone and cartilage development, homeostasis and disease. Cell Res. 2024;34(2):101-123. doi: 10.1038/s41422-023-00918-9
- Gao F, Xu Z, Liang Q, et al. Direct 3D printing of high strength biohybrid gradient hydrogel scaffolds for efficient repair of osteochondral defect. Adv Funct Mater. 2018;28(13):1706644. doi: 10.1002/adfm.201706644
- Dorcemus DL, Kim HS, Nukavarapu SP. Gradient scaffold with spatial growth factor profile for osteochondral interface engineering. Biomed Mater. 2021;16(3):035021. doi: 10.1088/1748-605X/abd1ba
- Qiao Z, Lian M, Han Y, et al. Bioinspired stratified electrowritten fiber-reinforced hydrogel constructs with layer-specific induction capacity for functional osteochondral regeneration. Biomaterials. 2021;266:120385. doi: 10.1016/j.biomaterials.2020.120385
- Chen C, Chen Y, Li M, et al. Functional decellularized fibrocartilaginous matrix graft for rotator cuff enthesis regeneration: a novel technique to avoid in-vitro loading of cells. Biomaterials. 2020;250:119996. doi: 10.1016/j.biomaterials.2020.119996
- Askari AT, Unzek S, Popovic ZB, et al. Effect of stromal-cell-derived factor 1 on stem-cell homing and tissue regeneration in ischaemic cardiomyopathy. Lancet. 2003;362(9385):697-703. doi: 10.1016/s0140-6736(03)14232-8
- Wang C, Dong J, Liu F, Liu N, Li L. 3D-printed PCL@BG scaffold integrated with SDF-1α-loaded hydrogel for enhancing local treatment of bone defects. J Biol Eng. 2024;18(1):1. doi: 10.1186/s13036-023-00401-4
- Luo H, Wang Z, Yu F, et al. Injectable and microporous microgel assembly with sequential bioactive factor release for the endogenous repair of nucleus pulposus. Adv Funct Mater. 2024;34(25):2315592. doi: 10.1002/adfm.202315592
- Li JH, Li Y, Huang D, Yao M. Role of stromal cell-derived factor-1 in endothelial progenitor cell-mediated vascular repair and regeneration. Tissue Eng Regen Med. 2021;18(5):747-758. doi: 10.1007/s13770-021-00366-9
- Zhou J, Li Q, Tian Z, Yao Q, Zhang M. Recent advances in 3D bioprinted cartilage-mimicking constructs for applications in tissue engineering. Mater Today Bio. 2023;23:100870. doi: 10.1016/j.mtbio.2023.100870
- Hoang DM, Pham PT, Bach TQ, et al. Stem cell-based therapy for human diseases. Signal Transduct Target Ther. 2022;7(1):272. doi: 10.1038/s41392-022-01134-4
- Kalluri R, LeBleu VS. The biology, function, and biomedical applications of exosomes. 2020;367(6478):eaau6977. doi: 10.1126/science.aau6977
- Kilian D, Ahlfeld T, Akkineni AR, Bernhardt A, Gelinsky M, Lode A. 3D bioprinting of osteochondral tissue substitutes – in vitro-chondrogenesis in multi-layered mineralized constructs. Sci Rep. 2020;10(1):8277. doi: 10.1038/s41598-020-65050-9
- Zhang S, Chuah SJ, Lai RC, Hui JHP, Lim SK, Toh WS. MSC exosomes mediate cartilage repair by enhancing proliferation, attenuating apoptosis and modulating immune reactivity. Biomaterials. 2018;156:16-27. doi: 10.1016/j.biomaterials.2017.11.028
- Sun T, Feng Z, He W, et al. Novel 3D-printing bilayer GelMA-based hydrogel containing BP, β-TCP and exosomes for cartilage-bone integrated repair. Biofabrication. 2023;16(1):015008. doi: 10.1088/1758-5090/ad04fe
- Li Q, Yu H, Zhao F, et al. 3D printing of microenvironment-specific bioinspired and exosome-reinforced hydrogel scaffolds for efficient cartilage and subchondral bone regeneration. Adv Sci (Weinh). 2023;10(26):e2303650. doi: 10.1002/advs.202303650
- Zhou X, Liu S, Lu Y, Wan M, Cheng J, Liu J. MitoEVs: a new player in multiple disease pathology and treatment. J Extracell Vesicles. 2023;12(4):e12320. doi: 10.1002/jev2.12320
- Sanz-Ros J, Mas-Bargues C, Romero-García N, Huete- Acevedo J, Dromant M, Borrás C. The potential use of mitochondrial extracellular vesicles as biomarkers or therapeutical tools. Int J Mol Sci. 2023;24(8):7005. doi: 10.3390/ijms24087005
- König T, McBride HM. Mitochondrial-derived vesicles in metabolism, disease, and aging. Cell Metab. 2024;36(1):21-35. doi: 10.1016/j.cmet.2023.11.014
- Chen P, Zheng L, Wang Y, et al. Desktop-stereolithography 3D printing of a radially oriented extracellular matrix/ mesenchymal stem cell exosome bioink for osteochondral defect regeneration. Theranostics. 2019;9(9):2439-2459. doi: 10.7150/thno.31017
- Stichler S, Jungst T, Schamel M, et al. Thiol-ene clickable poly(glycidol) hydrogels for biofabrication. Ann Biomed Eng. 2017;45(1):273-285. doi: 10.1007/s10439-016-1633-3
- Collins MN, Ren G, Young K, Pina S, Reis RL, Oliveira JM. Scaffold fabrication technologies and structure/function properties in bone tissue engineering. Adv Funct Mater. 2021;31(21):2010609. doi: 10.1002/adfm.202010609
- Xu W, Jambhulkar S, Zhu Y, et al. 3D printing for polymer/ particle-based processing: a review. Compos. B Eng. 2021;223:109102. doi: 10.1016/j.compositesb.2021.109102
- Engler AJ, Sen S, Sweeney HL, Discher DE. Matrix elasticity directs stem cell lineage specification. Cell. 2006;126(4):677-689. doi: 10.1016/j.cell.2006.06.044
- Zhou X, Tenaglio S, Esworthy T, et al. Three-dimensional printing biologically inspired DNA-based gradient scaffolds for cartilage tissue regeneration. ACS Appl Mater Interfaces. 2020;12(29):33219-33228. doi: 10.1021/acsami.0c07918
- Demoly F, Dunn ML, Wood KL, Qi HJ, André JC. The status, barriers, challenges, and future in design for 4D printing. Mater Des. 2021;212:110193. doi: 10.1016/j.matdes.2021.110193
- Agarwala S, Goh GL, Goh GD, Dikshit V, Yeong WY. Chapter 10 – 3D and 4D printing of polymer/CNTs-based conductive composites. In: Sadasivuni KK, Deshmukh K, Almaadeed MA, eds. 3D and 4D Printing of Polymer Nanocomposite Materials. Elsevier; 2020:297-324. doi:10.1016/B978-0-12-816805-9.00010-7
- Ryan KR, Down MP, Banks CE. Future of additive manufacturing: overview of 4D and 3D printed smart and advanced materials and their applications. Chem Eng J. 2021;403:126162. doi: 10.1016/j.cej.2020.126162
- Pintus E, Baldassarri M, Perazzo L, Natali S, Ghinelli D, Buda R. Stem cells in osteochondral tissue engineering. Adv Exp Med Biol. 2018;1058:359-372. doi: 10.1007/978-3-319-76711-6_16
- Rennerfeldt DA, Van Vliet KJ. Concise review: when colonies are not clones: evidence and implications of intracolony heterogeneity in mesenchymal stem cells. Stem Cells. 2016;34(5):1135-1141. doi: 10.1002/stem.2296
- Marędziak M, Marycz K, Tomaszewski KA, Kornicka K, Henry BM. The influence of aging on the regenerative potential of human adipose derived mesenchymal stem cells. Stem Cells Int. 2016;2016:2152435. doi: 10.1155/2016/2152435
- Elkhenany H, Amelse L, Caldwell M, Abdelwahed R, Dhar M. Impact of the source and serial passaging of goat mesenchymal stem cells on osteogenic differentiation potential: implications for bone tissue engineering. J Anim Sci Biotechnol. 2016;7:16. doi: 10.1186/s40104-016-0074-z
- Volk SW, Wang Y, Hankenson KD. Effects of donor characteristics and ex vivo expansion on canine mesenchymal stem cell properties: implications for MSC-based therapies. Cell Transplant. 2012;21(10):2189-2200. doi: 10.3727/096368912x636821
- Baxter FR, Bowen CR, Turner IG, Dent AC. Electrically active bioceramics: a review of interfacial responses. Ann Biomed Eng. 2010;38(6):2079-2092. doi: 10.1007/s10439-010-9977-6
- Jiang L, Wang Y, Liu Z, et al. Three-dimensional printing and injectable conductive hydrogels for tissue engineering application. Tissue Eng Part B Rev. 2019;25(5):398-411. doi: 10.1089/ten.TEB.2019.0100
- Wei K, Zhu M, Sun Y, et al. Robust biopolymeric supramolecular “Host−Guest Macromer” hydrogels reinforced by in situ formed multivalent nanoclusters for cartilage regeneration. Macromolecules. 2016; 49(3):866-875. doi: 10.1021/acs.macromol.5b02527
- Salzlechner C, Haghighi T, Huebscher I, et al. Adhesive hydrogels for maxillofacial tissue regeneration using minimally invasive procedures. Adv Healthc Mater. 2020;9(4):e1901134. doi: 10.1002/adhm.201901134
- Khare D, Basu B, Dubey AK. Electrical stimulation and piezoelectric biomaterials for bone tissue engineering applications. Biomaterials. 2020;258:120280. doi: 10.1016/j.biomaterials.2020.120280
- Zhang X, Zhang C, Lin Y, et al. Nanocomposite membranes enhance bone regeneration through restoring physiological electric microenvironment. ACS Nano. 2016;10(8):7279-7286. doi: 10.1021/acsnano.6b02247
- Deng C, Zhou Q, Zhang M, et al. Bioceramic scaffolds with antioxidative functions for ROS scavenging and osteochondral regeneration. Adv Sci (Weinh). 2022;9(12):e2105727. doi: 10.1002/advs.202105727