Extrusion bioprinting from a fluid mechanics perspective
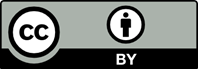
Bioprinting is an emerging technology for fabricating intricate and diverse structures that closely mimic natural tissues and organs for applications, such as tissue engineering, drug delivery, and cancer research. Among the various bioprinting techniques, extrusion-based bioprinting stands out due to its capability to apply a wide range of biomaterials and living cells and its controllability over printed structures. In bioprinting, bioink stored in a syringe is extruded through a nozzle connected to the syringe and deposited onto the printing stage to form 3D structures. The bioprinting process involves the flow of bioink through the syringe and nozzle, then spreading on a printing stage. As a result, fluid mechanics plays a crucial role in extrusion bioprinting. Notably, the biomaterials used in bioprinting are typically non-Newtonian fluids, which have complex viscoelastic and thixotropic behaviors; the influence of these behaviors on the bioprinting process has garnered considerable attention, with various methods employed, including numerical simulations via computational fluid dynamics (CFD). This paper reviews the latest developments in the fluid mechanics of extrusion-based bioprinting to shed light on the challenges and key considerations involved. This review covers the topics of extrusion bioprinting (including driving mechanisms, printability, and cell viability), biomaterial rheology and its effect on bioprinting, multi-material bioprinting, and numerical simulations of bioprinting. Key issues and challenges are also discussed along with recommendations for future research.

- Derakhshanfar S, Mbeleck R, Xu K, Zhang X, Zhong W, Xing M. 3D bioprinting for biomedical devices and tissue engineering: a review of recent trends and advances. Bioact Mater. 2018;3(2):144-156. doi: 10.1016/j.bioactmat.2017.11.008
- DeSimone E, Schacht K, Jungst T, Groll J, Scheibel T. Biofabrication of 3D constructs: fabrication technologies and spider silk proteins as bioinks. Pure Appl Chem. 2015;87(8):737-749. doi: 10.1515/pac-2015-0106
- Gao W, Zhang Y, Ramanujan D, et al. The status, challenges, and future of additive manufacturing in engineering. Computer-Aided Design. 2015;69:65-89. doi: 10.1016/j.cad.2015.04.001
- Ozbolat IT, Yin Yu. Bioprinting toward organ fabrication: challenges and future trends. IEEE Trans Biomed Eng. 2013;60(3):691-699. doi: 10.1109/TBME.2013.2243912
- Farzin A, Miri AK, Sharifi F, et al. 3D-printed sugar-based stents facilitating vascular anastomosis. Adv Healthc Mater. 2018;7(24):1800702. doi: 10.1002/adhm.201800702
- Do AV, Khorsand B, Geary SM, Salem AK. 3D printing of scaffolds for tissue regeneration applications. Adv Healthc Mater. 2015;4(12):1742-1762. doi: 10.1002/adhm.201500168
- Faramarzi N, Yazdi IK, Nabavinia M, et al. Patient-specific bioinks for 3D bioprinting of tissue engineering scaffolds. Adv Healthc Mater. 2018;7(11):1701347. doi: 10.1002/adhm.201701347
- Ozbolat IT, Hospodiuk M. Current advances and future perspectives in extrusion-based bioprinting. Biomaterials. 2016;76:321-343. doi: 10.1016/j.biomaterials.2015.10.076
- Bakarich SE, Gorkin R, Gately R, Naficy S, in het Panhuis M, Spinks GM. 3D printing of tough hydrogel composites with spatially varying materials properties. Addit Manuf. 2017;14:24-30. doi: 10.1016/j.addma.2016.12.003
- Kirchmajer DM, Gorkin R, In Het Panhuis M. An overview of the suitability of hydrogel-forming polymers for extrusion-based 3D-printing. J Mater Chem B. 2015;3: 4105-4117. doi: 10.1039/c5tb00393h
- Tavafoghi M, Darabi MA, Mahmoodi M, et al. Multimaterial bioprinting and combination of processing techniques towards the fabrication of biomimetic tissues and organs. Biofabrication. 2021;13(4):042002. doi: 10.1088/1758-5090/ac0b9a
- Schwab A, Levato R, D’Este M, Piluso S, Eglin D, Malda J. Printability and shape fidelity of bioinks in 3D bioprinting. Chem Rev. 2020;120(19):11028-11055. doi: 10.1021/acs.chemrev.0c00084
- Richard C, Neild A, Cadarso VJ. The emerging role of microfluidics in multi-material 3D bioprinting. Lab Chip. 2020;20(12):2044-2056. doi: 10.1039/c9lc01184f
- Askari M, Afzali Naniz M, Kouhi M, Saberi A, Zolfagharian A, Bodaghi M. Recent progress in extrusion 3D bioprinting of hydrogel biomaterials for tissue regeneration: a comprehensive review with focus on advanced fabrication techniques. Biomater Sci. 2021;9(3):535-573. doi: 10.1039/d0bm00973c
- Zhang J, Allardyce BJ, Rajkhowa R, et al. 3D printing of silk particle-reinforced chitosan hydrogel structures and their properties. ACS Biomater Sci Eng. 2018;4(8):3036-3046. doi: 10.1021/acsbiomaterials.8b00804
- Aydogdu MO, Oner ET, Ekren N, et al. Comparative characterization of the hydrogel added PLA/β-TCP scaffolds produced by 3D bioprinting. Bioprinting. 2019; 13:e00046. doi: 10.1016/j.bprint.2019.e00046
- Yan J, Wang Y, Zhang X, et al. Snakegourd root/Astragalus polysaccharide hydrogel preparation and application in 3D printing. Int J Biol Macromol. 2019;121:309-316. doi: 10.1016/j.ijbiomac.2018.10.008
- Yazdanpanah Z, Johnston JD, Cooper DML, Chen X. 3D bioprinted scaffolds for bone tissue engineering: state-of-the-art and emerging technologies. Front Bioeng Biotechnol. 2022;10(April):824156. doi: 10.3389/fbioe.2022.824156
- Ratner BD, Bryant SJ. Biomaterials: where we have been and where we are going. Annu Rev Biomed Eng. 2004;6:41-75. doi: 10.1146/annurev.bioeng.6.040803.140027
- Caló E, Khutoryanskiy VV. Biomedical applications of hydrogels: a review of patents and commercial products. Eur Polym J. 2015;65:252-267. doi: 10.1016/j.eurpolymj.2014.11.024
- Roehm KD, Madihally SV. Bioprinted chitosan-gelatin thermosensitive hydrogels using an inexpensive 3D printer. Biofabrication. 2018;10(1):015002. doi: 10.1088/1758-5090/aa96dd
- Mora-Boza A, Włodarczyk-Biegun MK, Del Campo A, Vázquez-Lasa B, Román JS. Glycerylphytate as an ionic crosslinker for 3D printing of multi-layered scaffolds with improved shape fidelity and biological features. Biomater Sci. 2019;8(1):506-516. doi: 10.1039/c9bm01271k
- Chen XB, Fazel Anvari-Yazdi A, Duan X, et al. Biomaterials / bioinks and extrusion bioprinting. Bioact Mater. 2023;28:511-536. doi: 10.1016/j.bioactmat.2023.06.006
- Akkineni AR, Ahlfeld T, Lode A, Gelinsky M. A versatile method for combining different biopolymers in a core/ shell fashion by 3D plotting to achieve mechanically robust constructs. Biofabrication. 2016;8(4):045001. doi: 10.1088/1758-5090/8/4/045001
- Liu X, Zhao K, Gong T, et al. Delivery of growth factors using a smart porous nanocomposite scaffold to repair a mandibular bone defect. Biomacromolecules. 2014;15(3):1019-1030. doi: 10.1021/bm401911p
- Augst AD, Kong HJ, Mooney DJ. Alginate hydrogels as biomaterials. Macromol Biosci. 2006;6(8):623-633. doi: 10.1002/mabi.200600069
- Izadifar Z, Chang T, Kulyk W, Chen X, Eames BF. Analyzing biological performance of 3D-printed, cell-impregnated hybrid constructs for cartilage tissue engineering. Tissue Eng Part C Methods. 2016;22:173-188. doi: 10.1089/ten.tec.2015.0307
- Naghieh S, Sarker M, Izadifar M, Chen X. Dispensing-based bioprinting of mechanically-functional hybrid scaffolds with vessel-like channels for tissue engineering applications – a brief review. J Mech Behav Biomed Mater. 2018;78:298-314. doi: 10.1016/j.jmbbm.2017.11.037
- Kiyotake EA, Douglas AW, Thomas EE, Nimmo SL, Detamore MS. Development and quantitative characterization of the precursor rheology of hyaluronic acid hydrogels for bioprinting. Acta Biomater. 2019;95:176-187. doi: 10.1016/j.actbio.2019.01.041
- Hölzl K, Lin S, Tytgat L, Van Vlierberghe S, Gu L, Ovsianikov A. Bioink properties before, during and after 3D bioprinting. Biofabrication. 2016;8(3):032002. doi: 10.1088/1758-5090/8/3/032002
- Monika Hospodiuk KKMMDITO. Extrusion-Based Biofabrication in Tissue Engineering and Regenerative Medicine. 1st ed. Springer; 2018.
- Khalil S, Nam J, Sun W. Multi-nozzle deposition for construction of 3D biopolymer tissue scaffolds. Rapid Prototyp J. 2005;11(1):9-17. doi: 10.1108/13552540510573347
- Boularaoui S, Al Hussein G, Khan KA, Christoforou N, Stefanini C. An overview of extrusion-based bioprinting with a focus on induced shear stress and its effect on cell viability. Bioprinting. 2020;20(August):e00093. doi: 10.1016/j.bprint.2020.e00093
- Ning L, Yang B, Mohabatpour F, et al. Process-induced cell damage: pneumatic versus screw-driven bioprinting. Biofabrication. 2020;12(2):025011. doi: 10.1088/1758-5090/ab5f53
- Murphy SV, Atala A. 3D bioprinting of tissues and organs. Nat Biotechnol. 2014;32(8):773-785. doi: 10.1038/nbt.2958
- Daniel X. B. Chen. Extrusion Bioprinting of Scaffolds for Tissue Engineering Applications. 1st ed. Springer Nature; 2019.
- Malekpour A, Chen X. Printability and cell viability in extrusion-based bioprinting from experimental, computational, and machine learning views. J Funct Biomater. 2022;13(2):40. doi: 10.3390/jfb13020040
- Fu Z, Naghieh S, Xu C, Wang C, Sun W, Chen X. Printability in extrusion bioprinting. Biofabrication. 2021;13(3):033001. doi: 10.1088/1758-5090/abe7ab
- Gillispie G, Prim P, Copus J, et al. Assessment methodologies for extrusion-based bioink printability. Biofabrication. 2020;12(2):22003. doi: 10.1088/1758-5090/ab6f0d
- Xu X, Jagota A, Peng S, Luo D, Wu M, Hui CY. Gravity and surface tension effects on the shape change of soft materials. Langmuir. 2013;29(27):8665-8674. doi: 10.1021/la400921h
- Hospodiuk M, Dey M, Sosnoski D, Ozbolat IT. The bioink: a comprehensive review on bioprintable materials. Biotechnol Adv. 2017;35(2):217-239. doi: 10.1016/j.biotechadv.2016.12.006
- Petta D, Grijpma DW, Alini M, Eglin D, D’Este M. Three-dimensional printing of a tyramine hyaluronan derivative with double gelation mechanism for independent tuning of shear thinning and postprinting curing. ACS Biomater Sci Eng. 2018;4(8):3088-3098. doi: 10.1021/acsbiomaterials.8b00416
- Gao T, Gillispie GJ, Copus JS, et al. Optimization of gelatin– alginate composite bioink printability using rheological parameters: a systematic approach. Biofabrication. 2018;10(3):034106. doi: 10.1088/1758-5090/aacdc7
- Saha D, Bhattacharya S. Hydrocolloids as thickening and gelling agents in food: a critical review. J Food Sci Technol. 2010;47(6):587-597. doi: 10.1007/s13197-010-0162-6
- He Y, Yang F, Zhao H, Gao Q, Xia B, Fu J. Research on the printability of hydrogels in 3D bioprinting. Sci Rep. 2016;6(1):29977. doi: 10.1038/srep29977
- Soltan N, Ning L, Mohabatpour F, Papagerakis P, Chen X. Printability and cell viability in bioprinting alginate dialdehyde-gelatin scaffolds. ACS Biomater Sci Eng. 2019;5(6):2976-2987. doi: 10.1021/acsbiomaterials.9b00167
- Lee JM, Ng WL, Yeong WY. Resolution and shape in bioprinting: strategizing towards complex tissue and organ printing. Appl Phys Rev. 2019;6(1):011307. doi: 10.1063/1.5053909
- Ribeiro A, Blokzijl MM, Levato R, et al. Assessing bioink shape fidelity to aid material development in 3D bioprinting. Biofabrication. 2017;10(1):014102. doi: 10.1088/1758-5090/aa90e2
- Ouyang L, Yao R, Zhao Y, Sun W. Effect of bioink properties on printability and cell viability for 3D bioplotting of embryonic stem cells. Biofabrication. 2016;8(3):035020. doi: 10.1088/1758-5090/8/3/035020
- Herrada-Manchón H, Fernández MA, Aguilar E. Essential guide to hydrogel rheology in extrusion 3D printing: how to measure it and why it matters? Gels. 2023;9(7):517. doi: 10.3390/gels9070517
- Cooke ME, Rosenzweig DH. The rheology of direct and suspended extrusion bioprinting. APL Bioeng. 2021;5(1):011502. doi: 10.1063/5.0031475
- Clasen C, Entov V, Bico J, McKinley GH. `Gobbling Drops´ : the jetting / dripping transition in flows of polymeric liquids. J Fluid Mech. 2009;636:5-40. doi: 10.1017/S0022112009008143
- Clanet C, Lasheras JC. Transition from dripping to jetting. J Fluid Mech. 1999;383:307-326. doi: 10.1017/S0022112098004066
- Philip M. Gerhart, Andrew L. Gerhart, John I. Hochstein. Fundamentals of Fluid Mechanics. 8th ed. Wiley; 2018.
- Liliang Ouyang. 3D bioprinting of thermal-sensitive bioink. In: Study on Microextrusion-Based 3D Bioprinting and Bioink Crosslinking Mechanisms. Springer; 2019.
- Savart F. M’emoire sur la constitution des veines liquides lanc’ees par des ori ces circulaires en mince paroi. Ann Chim (Paris). 1833;53:337-386.
- Plateau J. Statique Expe’rimentale et The’orique des Liquides. In: Gauthier-Villars et Cie; 1873.
- Lord R. Investigations in capillarity: the size of drops. - The liberation of gas from supersaturated solutions. - Colliding jets. - The tension of contaminated water-surfaces. Curious observation. Philos Magaz J Sci. 1899;48(293):321-337.
- Chen XB, Ke H. Effects of fluid properties on dispensing processes for electronics packaging. IEEE Trans Electron Pack Manuf. 2006;29(2):75-82. doi: 10.1109/TEPM.2006.874964
- De Maria C, Vozzi G, Moroni L. Multimaterial, heterogeneous, and multicellular three-dimensional bioprinting. MRS Bull. 2017;42(8):578-584. doi: 10.1557/mrs.2017.165
- You F, Wu X, Zhu N, Lei M, Eames BF, Chen X. 3D printing of porous cell-laden hydrogel constructs for potential applications in cartilage tissue engineering. ACS Biomater Sci Eng. 2016;2(7). doi: 10.1021/acsbiomaterials.6b00258
- Ning L, Sun H, Lelong T, et al. 3D bioprinting of scaffolds with living Schwann cells for potential nerve tissue engineering applications. Biofabrication. 2018;10(3):035014. doi: 10.1088/1758-5090/aacd30
- Ning L, Betancourt N, Schreyer DJ, Chen X. Characterization of cell damage and proliferative ability during and after bioprinting. ACS Biomater Sci Eng. 2018;4(11):3906-3918. doi: 10.1021/acsbiomaterials.8b00714
- Xu HQ, Liu JC, Zhang ZY, Xu CX. A review on cell damage, viability, and functionality during 3D bioprinting. Mil Med Res. 2022;9(1):1-15. doi: 10.1186/s40779-022-00429-5
- Fakhruddin K, Hamzah MSA, Razak SIA. Effects of extrusion pressure and printing speed of 3D bioprinted construct on the fibroblast cells viability. IOP Conf Ser Mater Sci Eng. 2018;440(1):012042. doi: 10.1088/1757-899X/440/1/012042
- Ning L, Chen X. A brief review of extrusion-based tissue scaffold bio-printing. Biotechnol J. 2017;12(8):1200-1210. doi: 10.1002/biot.201600671
- Bae YB, Jang HK, Shin TH, et al. Microfluidic assessment of mechanical cell damage by extensional stress. Lab Chip. 2016;16(1):96-103. doi: 10.1039/C5LC01006C
- Aguado BA, Mulyasasmita W, Su J, Lampe KJ, Heilshorn SC. Improving viability of stem cells during syringe needle flow through the design of hydrogel cell carriers. Tissue Eng Part A. 2012;18(7-8):806-815. doi: 10.1089/ten.tea.2011.0391
- Down LA, Papavassiliou DV, O’Rear EA. Significance of extensional stresses to red blood cell lysis in a shearing flow. Ann Biomed Eng. 2011;39(6):1632-1642. doi: 10.1007/s10439-011-0262-0
- Byron Bird R, Stewart WE, Lightfoot EN. Transport Phenomena. 2nd ed. Wiley; 2009.
- Cogswell FN. Measuring the extensional rheology of polymer melts. Trans Soc Rheol. 1972;16(3):383-403. doi: 10.1122/1.549257
- Mitsoulis E, Hatzikiriakos SG, Christodoulou K, Vlassopoulos D. Sensitivity analysis of the Bagley correction to shear and extensional rheology. Rheol Acta. 1998;37(5):438-448. doi: 10.1007/s003970050131
- Han S, Kim CM, Jin S, Kim TY. Study of the process-induced cell damage in forced extrusion bioprinting. Biofabrication. 2021;13(3):035048. doi: 10.1088/1758-5090/ac0415
- Chirianni F, Vairo G, Marino M. Development of process design tools for extrusion-based bioprinting: From numerical simulations to nomograms through reduced-order modeling. Comput Methods Appl Mech Eng. 2024;419:116685. doi: 10.1016/j.cma.2023.116685
- Li M, Tian X, Zhu N, Schreyer DJ, Chen X. Modeling process-induced cell damage in the biodispensing process. Tissue Eng Part C Methods. 2010;16(3):533-542. doi: 10.1089/ten.tec.2009.0178
- Li M, Tian X, Schreyer DJ, Chen X. Effect of needle geometry on flow rate and cell damage in the dispensing-based biofabrication process. Biotechnol Prog. 2011;27(6):1777-1784. doi: 10.1002/btpr.679
- Chhabra RP, Richardson JF. Non-Newtonian Dlow and Applied Rheology. Oxford, UK: Butterworth-Heinemann 2nd ed.; 2008. doi: 10.1016/B978-0-7506-8532-0.X0001-7
- Byron Bird R, Robert C, Armstrong OH. Dynamics of Polymeric Liquids. 2nd ed. John Wiley & Sons; 1987. doi: 10.1002/aic.690340623
- Cooke ME, Rosenzweig DH. The rheology of direct and suspended extrusion bioprinting. APL Bioeng. 2021;5(1):011502. doi: 10.1063/5.0031475
- Ostwald W. Ueber die rechnerische Darstellung des Strukturgebietes der Viskosität. Kolloid-Zeitschrift. 1929;47(2):176-187. doi: 10.1007/BF01496959
- de Waele A. Viscometry and plastometry. J Oil and Colour Chemists’ Assoc. 1923;6(38):33-88.
- Carreau PiJ. Rheological equations from molecular network theories. Trans Soc Rheol. 1972;16(1):99-127. doi: 10.1122/1.549276.
- Yasuda K. Investigation of the Analogies Berween Viscometric and Linear Viscoelastic Properties of Polystyrene Fluids. Massachusetts Institute of Technology; 1979. http://hdl.handle.net/1721.1/16043
- Cross MM. Rheology of non-Newtonian fluids: a new flow equation for pseudoplastic systems. J Colloid Sci. 1965;20(5):417-437. doi: 10.1016/0095-8522(65)90022-X
- Herschel VWH, Bulkley R. Ronsistenzmessungen yon ( ummi-BenzollGsungen). Colloid Polym Sci. 1926;39:291-300. doi: 10.1007/BF01432034
- Nelson AZ, Schweizer KS, Rauzan BM, Nuzzo RG, Vermant J, Ewoldt RH. Designing and transforming yield-stress fluids. Curr Opin Solid State Mater Sci. 2019;23(5):100758. doi: 10.1016/j.cossms.2019.06.002
- Pereira RF, Sousa A, Barrias CC, Bártolo PJ, Granja PL. A single-component hydrogel bioink for bioprinting of bioengineered 3D constructs for dermal tissue engineering. Mater Horiz. 2018;5(6):1100-1111. doi: 10.1039/C8MH00525G
- Ramesh S, Harrysson OLA, Rao PK, et al. Extrusion bioprinting: Recent progress, challenges, and future opportunities. Bioprinting. 2021;21(November 2020): e00116. doi: 10.1016/j.bprint.2020.e00116
- Nair K, Gandhi M, Khalil S, et al. Characterization of cell viability during bioprinting processes. Biotechnol J. 2009;4(8):1168-1177. doi: 10.1002/biot.200900004
- Jiang Y, Zhou J, Feng C, Shi H, Zhao G, Bian Y. Rheological behavior, 3D printability and the formation of scaffolds with cellulose nanocrystals/gelatin hydrogels. J Mater Sci. 2020;55(33):15709-15725. doi: 10.1007/s10853-020-05128-x
- Ouyang L, Armstrong JPK, Lin Y, et al. Expanding and optimizing 3D bioprinting capabilities using complementary network bioinks. Sci Adv. 2020;6(38):eabc5529. doi: 10.1126/sciadv.abc5529
- Paxton N, Smolan W, Böck T, Melchels F, Groll J, Jungst T. Proposal to assess printability of bioinks for extrusion-based bioprinting and evaluation of rheological properties governing bioprintability. Biofabrication. 2017;9(4):044107. doi: 10.1088/1758-5090/aa8dd8
- Tuladhar S, Nelson C, Habib A. Rheological study of highly thixotropic hydrogels for 3D bio-printing processes. In: Ghate A, Krishnaiyer K, Paynabar K, eds. Proceedings of the 2021 IISE Annual Conference; 2021.
- Diañez I, Gallegos C, Brito-de la Fuente E, et al. 3D printing in situ gelification of κ-carrageenan solutions: effect of printing variables on the rheological response. Food Hydrocoll. 2019;87:321-330. doi: 10.1016/j.foodhyd.2018.08.010
- Herrada-Manchón H, Celada L, Rodríguez-González D, Alejandro Fernández M, Aguilar E, Chiara MD. Three-dimensional bioprinted cancer models: A powerful platform for investigating tunneling nanotube-like cell structures in complex microenvironments. Mater Sci Eng: C. 2021;128:112357. doi: 10.1016/j.msec.2021.112357
- Chen Y, Wang Y, Yang Q, et al. A novel thixotropic magnesium phosphate-based bioink with excellent printability for application in 3D printing. J Mater Chem B. 2018;6(27):4502-4513. doi: 10.1039/C8TB01196F
- Jungst T, Smolan W, Schacht K, Scheibel T, Groll J. Strategies and molecular design criteria for 3D printable hydrogels. Chem Rev. 2016;116(3):1496-1539. doi: 10.1021/acs.chemrev.5b00303
- Ma J, Lin Y, Chen X, Zhao B, Zhang J. Flow behavior, thixotropy and dynamical viscoelasticity of sodium alginate aqueous solutions. Food Hydrocoll. 2014;38:119-128. doi: 10.1016/j.foodhyd.2013.11.016
- Cheng Y, Qin H, Acevedo NC, Jiang X, Shi X. 3D printing of extended-release tablets of theophylline using hydroxypropyl methylcellulose (HPMC) hydrogels. Int J Pharm. 2020;591:119983. doi: 10.1016/j.ijpharm.2020.119983
- Sombatsompop N, Sergsiri S. Die swell ratio of polystyrene melt from an electro-magnetized capillary die in an extrusion rheometer: effects of barrel diameter, shear rate and die temperature. Polym Adv Technol. 2004;15(8):472-480. doi: 10.1002/pat.490
- Michal Bathory. Modelling and analysis of flows of viscoelastic fluids Beyond the Navier–Stokes equations. Res Outreach. 2022;(132). doi: 10.32907/RO-132-3331282634
- Boger DV, Binnington RJ. Experimental removal of the re‐entrant corner singularity in tubular entry flows. J Rheol. 1994;38(2):333-349. doi: 10.1122/1.550517
- Maklad O, Poole RJ. A review of the second normal-stress difference; its importance in various flows, measurement techniques, results for various complex fluids and theoretical predictions. J Nonnewton Fluid Mech. 2021;292:104522. doi: 10.1016/j.jnnfm.2021.104522
- R J Poole. The Deborah and Weissenberg numbers. Rheol Bull. 2012;52(2):32-39.
- Wu Q, Therriault D, Heuzey MC. Processing and properties of chitosan inks for 3D printing of hydrogel microstructures. ACS Biomater Sci Eng. 2018;4(7):2643-2652. doi: 10.1021/acsbiomaterials.8b00415
- Vlachopoulos J, Polychronopoulos N. Basic concepts in polymer melt rheology and their importance in processing. In: Kontopoulou M, eds. Applied Polymer Rheology. John Wiley & Sons, Inc.; 2011:1-27. doi: 10.1002/9781118140611.ch1
- Koopmans RJ. Die swell or extrudate swell. In: Karger-Kocsis J, ed. Polypropylene: An A-Z Reference. Kluwer Publishers; 1999.
- Sodupe-Ortega E, Sanz-Garcia A, Pernia-Espinoza A, Escobedo-Lucea C. Accurate calibration in multi-material 3D bioprinting for tissue engineering. Materials (Basel). 2018;11(8):1402. doi: 10.3390/ma11081402
- Anna SL, Rogers C, McKinley GH. On controlling the kinematics of a filament stretching rheometer using a real-time active control mechanism. J Nonnewton Fluid Mech. 1999;87(2-3):307-335. doi: 10.1016/S0377-0257(99)00072-5
- Ooi YW, Sridhar T. Extensional rheometry of fluid S1. J Nonnewton Fluid Mech. 1994;52(2):153-162. doi: 10.1016/0377-0257(94)80047-2
- McKinley GH, Tripathi A, Yao M. Extensional rheometry of polymeric fluids and the uniaxial elongation of viscoelastic filaments. In: Kruijt PGM, Meijer HEH, van de Vosse FN, eds. 15th Annual Meeting of the International Polymer Processing Society; 1999.
- Göhl J, Markstedt K, Mark A, Håkansson K, Gatenholm P, Edelvik F. Simulations of 3D bioprinting: Predicting bioprintability of nanofibrillar inks. Biofabrication. 2018;10(3):34105. doi: 10.1088/1758-5090/aac872
- Zaeri A, Zgeib R, Cao K, Zhang F, Chang RC. Numerical analysis on the effects of microfluidic-based bioprinting parameters on the microfiber geometrical outcomes. Sci Rep. 2022;12(1):3364. doi: 10.1038/s41598-022-07392-0
- Hemasian Etefagh A, Razfar MR. Numerical study of the process parameters affecting the feature size of a microfiber fabricated by microfluidic-based bioprinting. Proc Instit Mech Eng E: J Process Mech Eng. 2024. doi: 10.1177/09544089241236265
- Ma J, Lin Y, Chen X, Zhao B, Zhang J. Flow behavior, thixotropy and dynamical viscoelasticity of sodium alginate aqueous solutions. Food Hydrocoll. 2014;38:119-128. doi: 10.1016/j.foodhyd.2013.11.016
- Talluri DJS, Nguyen HT, Avazmohammadi R, Miri AK. Ink rheology regulates stability of bioprinted strands. J Biomech Eng. 2022;144(7) 074503. doi: 10.1115/1.4053404
- Gregory T, Benhal P, Scutte A, et al. Rheological characterization of cell-laden alginate-gelatin hydrogels for 3D biofabrication. J Mech Behav Biomed Mater. 2022;136:105474. doi: 10.1016/j.jmbbm.2022.105474
- López-Marcial GR, Zeng AY, Osuna C, Dennis J, García JM, O’Connell GD. Agarose-based hydrogels as suitable bioprinting materials for tissue engineering. ACS Biomater Sci Eng. 2018;4(10):3610-3616. doi: 10.1021/acsbiomaterials.8b00903
- Benchabane A, Bekkour K. Rheological properties of carboxymethyl cellulose (CMC) solutions. Colloid Polym Sci. 2008;286(10):1173-1180. doi: 10.1007/s00396-008-1882-2
- Ramezani H, Mohammad Mirjamali S, He Y. Simulations of extrusion 3d printing of chitosan hydrogels. Appl Sci. 2022;12(15):7530. doi: 10.3390/app12157530
- Rahimnejad M, Labonté-Dupuis T, Demarquette NR, Lerouge S. A rheological approach to assess the printability of thermosensitive chitosan-based biomaterial inks. Biomed Mater. 2021;16(1):015003. doi: 10.1088/1748-605X/abb2d8
- Lan X, Adesida A, Boluk Y. Rheological and viscoelastic properties of collagens and their role in bioprinting by micro-extrusion. Biomed Mater. 2022;17(6):062005. doi: 10.1088/1748-605X/ac9b06
- Andrade RD, Skurtys O, Osorio F, Zuluaga R, Gañán P, Castro C. Rheological and physical properties of gelatin suspensions containing cellulose nanofibers for potential coatings. Food Sci Technol Int. 2015;21(5):332-341. doi: 10.1177/1082013214535944
- Kokol V, Pottathara YB, Mihelčič M, Perše LS. Rheological properties of gelatine hydrogels affected by flow- and horizontally-induced cooling rates during 3D cryo-printing. Colloids Surf A Physicochem Eng Asp. 2021;616:126356. doi: 10.1016/j.colsurfa.2021.126356
- Tirella A, De Maria C, Criscenti G, Vozzi G, Ahluwalia A. The PAM 2 system: a multilevel approach for fabrication of complex three-dimensional microstructures. Rapid Prototyp J. 2012;18(4):299-307. doi: 10.1108/13552541211231725
- Naghieh S, Karamooz-Ravari MR, Sarker M, Karki E, Chen X. Influence of crosslinking on the mechanical behavior of 3D printed alginate scaffolds: experimental and numerical approaches. J Mech Behav Biomed Mater. 2018;80:111-118. doi: 10.1016/j.jmbbm.2018.01.034
- Sarker Md, Izadifar M, Schreyer D, Chen X. Influence of ionic crosslinkers (Ca 2+ /Ba 2+ /Zn 2+ ) on the mechanical and biological properties of 3D Bioplotted Hydrogel Scaffolds. J Biomater Sci Polym Ed. 2018;29(10):1126-1154. doi: 10.1080/09205063.2018.1433420
- Pati F, Jang J, Ha DH, et al. Printing three-dimensional tissue analogues with decellularized extracellular matrix bioink. Nat Commun. 2014;5(1):3935. doi: 10.1038/ncomms4935
- Chang R, Nam J, Sun W. Effects of dispensing pressure and nozzle diameter on cell survival from solid freeform fabrication-based direct cell writing. Tissue Eng Part A. 2008;14(1):41-48. doi: 10.1089/ten.a.2007.0004
- Ashammakhi N, Ahadian S, Xu C, et al. Bioinks and bioprinting technologies to make heterogeneous and biomimetic tissue constructs. Mater Today Bio. 2019;1:100008. doi: 10.1016/j.mtbio.2019.100008
- Ozbolat IT, Chen H, Yu Y. Development of “Multi-arm Bioprinter” for hybrid biofabrication of tissue engineering constructs. Robot Comput Integr Manuf. 2014;30(3):295-304. doi: 10.1016/j.rcim.2013.10.005
- Hong S, Kim JS, Jung B, Won C, Hwang C. Coaxial bioprinting of cell-laden vascular constructs using a gelatin-tyramine bioink. Biomater Sci. 2019;7(11):4578-4587. doi: 10.1039/c8bm00618k
- Gao G, Lee JH, Jang J, et al. Tissue Engineered Bio-Blood- Vessels Constructed Using a Tissue-Specific Bioink and 3D Coaxial Cell Printing Technique: A Novel Therapy for Ischemic Disease. Adv Funct Mater. 2017;27(33):1700798. doi: 10.1002/adfm.201700798
- Shao L, Gao Q, Xie C, Fu J, Xiang M, He Y. Directly coaxial 3D bioprinting of large-scale vascularized tissue constructs. Biofabrication. 2020;12(3):035014. doi: 10.1088/1758-5090/ab7e76
- Millik SC, Dostie AM, Karis DG, et al. 3D printed coaxial nozzles for the extrusion of hydrogel tubes toward modeling vascular endothelium. Biofabrication. 2019;11(4):45009. doi: 10.1088/1758-5090/ab2b4d
- Gao Q, He Y, Fu JZ, Liu A, Ma L. Coaxial nozzle-assisted 3D bioprinting with built-in microchannels for nutrients delivery. Biomaterials. 2015;61:203-215. doi: 10.1016/j.biomaterials.2015.05.031
- Mistry P, Aied A, Alexander M, Shakesheff K, Bennett A, Yang J. Bioprinting using mechanically robust core– shell cell-laden hydrogel strands. Macromol Biosci. 2017;17(6)1600472. doi: 10.1002/mabi.201600472
- Tomasina C, Bodet T, Mota C, Moroni L, Camarero- Espinosa S. Bioprinting vasculature: materials, cells and emergent techniques. Materials (Basel). 2019;12(17):2701. doi: 10.3390/ma12172701
- Cameron T, Naseri E, MacCallum B, Ahmadi A. Development of a disposable single-nozzle printhead for 3D bioprinting of continuous multi-material constructs. Micromachines (Basel). 2020;11(5):459. doi: 10.3390/mi11050459
- Nelson C, Tuladhar S, Habib A. Designing an interchangeable multi-material nozzle system for the three-dimensional bioprinting process. J Med Device. 2023;17(2):1-8. doi: 10.1115/1.4055249
- Puertas-Bartolomé M, Włodarczyk-Biegun MK, Del Campo A, Vázquez-Lasa B, Román JS. 3D printing of a reactive hydrogel bio-ink using a static mixing tool. Polymers (Basel). 2020;12(9):1-17. doi: 10.3390/polym12091986
- Rocca M, Fragasso A, Liu W, Heinrich MA, Zhang YS. Embedded multimaterial extrusion bioprinting. SLAS Technol. 2018;23(2):154-163. doi: 10.1177/2472630317742071
- Liu W, Zhang YS, Heinrich MA, et al. Rapid continuous multimaterial extrusion bioprinting. Adv Mater. 2017;29(3):10.1002/adma.201604630. doi: 10.1002/adma.201604630
- Puryear III JR, Yoon JK, Kim Y. Advanced fabrication techniques of microengineered physiological systems. Micromachines (Basel). 2020;11(8):730. doi: 10.3390/mi11080730
- Kantak C, Beyer S, Trau D. A novel microfluidic droplet manipulation method for fabrication of reverse-phase two layer layer-by-layer protein microcapsules. In: Zengerle R, eds. 17th Int. Conf. on Miniaturized Systems for Chemistry and Life Sciences (MicroTAS 2013) ; 2013.
- Shin SR, Kilic T, Zhang YS, et al. Label‐free and regenerative electrochemical microfluidic biosensors for continual monitoring of cell secretomes. Adv Sci. 2017;4(5):1600522. doi: 10.1002/advs.201600522
- Bsoul A, Pan S, Cretu E, Stoeber B, Walus K. Design, microfabrication, and characterization of a moulded PDMS/ SU-8 inkjet dispenser for a Lab-on-a-Printer platform technology with disposable microfluidic chip. Lab Chip. 2016;16(17):3351-3361. doi: 10.1039/C6LC00636A
- Davoodi E, Sarikhani E, Montazerian H, et al. Extrusion and microfluidic-based bioprinting to fabricate biomimetic tissues and organs. Adv Mater Technol. 2020;5(8):1901044. doi: 10.1002/admt.201901044
- Hakimi N, Cheng R, Leng L, et al. Handheld skin printer: in situ formation of planar biomaterials and tissues. Lab Chip. 2018;18(10):1440-1451. doi: 10.1039/c7lc01236e
- Onoe H, Okitsu T, Itou A, et al. Metre-long cell-laden microfibres exhibit tissue morphologies and functions. Nat Mater. 2013;12(6):584-590. doi: 10.1038/nmat3606
- Raja N, Yun H suk. A simultaneous 3D printing process for the fabrication of bioceramic and cell-laden hydrogel core/shell scaffolds with potential application in bone tissue regeneration. J Mater Chem B. 2016;4(27):4707-4716. doi: 10.1039/C6TB00849F
- Angelozzi M, Miotto M, Penolazzi L, et al. Composite ECM-alginate microfibers produced by microfluidics as scaffolds with biomineralization potential. Mater Sci Eng C. 2015;56:141-153. doi: 10.1016/j.msec.2015.06.004
- Lee C, Abelseth E, de la Vega L, Willerth SM. Bioprinting a novel glioblastoma tumor model using a fibrin-based bioink for drug screening. Mater Today Chem. 2019; 12:78-84. doi: 10.1016/j.mtchem.2018.12.005
- Pati F, Jang J, Lee JW, and D.-W. Cho C. Extrusion Bioprinting. In: Atala A, Yoo JJ, eds. Extrusion Bioprinting, in Essentials of 3D Biofabrication and Translation. 1st ed. Academic Press; 2015.
- Hardin JO, Ober TJ, Valentine AD, Lewis JA. Microfluidic printheads for multimaterial 3d printing of viscoelastic inks. Adv Mater. 2015;27(21):3279-3284. doi: 10.1002/adma.201500222
- du Chatinier DN, Figler KP, Agrawal P, Liu W, Zhang YS. The potential of microfluidics-enhanced extrusion bioprinting. Biomicrofluidics. 2021;15(4):041304. doi: 10.1063/5.0033280
- Utada AS, Fernandez-Nieves A, Stone HA, Weitz DA. Dripping to jetting transitions in coflowing liquid streams. Phys Rev Lett. 2007;99(9):094502. doi: 10.1103/PhysRevLett.99.094502
- Derzsi L, Kasprzyk M, Plog JP, Garstecki P. Flow focusing with viscoelastic liquids. Phys Fluids. 2013;25(9):092001. doi: 10.1063/1.4817995
- Ober TJ, Foresti D, Lewis JA. Active mixing of complex fluids at the microscale. Proc Natl Acad Sci USA. 2015;112(40):12293-12298. doi: 10.1073/pnas.1509224112
- Hessel V, Löwe H, Schönfeld F. Micromixers—a review on passive and active mixing principles. Chem Eng Sci. 2005;60(8-9):2479-2501. doi: 10.1016/j.ces.2004.11.033
- Grace HP. Dispersion phenomena in high viscosity immiscible fluid systems and application of static mixers as dispersion devices in such systems. Chem Eng Commun. 1982;14(3-6):225-277. doi: 10.1080/00986448208911047
- Chávez-Madero C, De León-Derby MD, Samandari M, et al. Using chaotic advection for facile high-throughput fabrication of ordered multilayer micro-and nanostructures: continuous chaotic printing. Biofabrication. 2020;12(3): 035023. doi: 10.1088/1758-5090/ab84cc
- Ober TJ, Foresti D, Lewis JA. Active mixing of complex fluids at the microscale. Proc Natl Acad Sci USA. 2015;112(40):12293-12298. doi: 10.1073/pnas.1509224112
- Kuzucu M, Vera G, Beaumont M, et al. Extrusion-based 3D bioprinting of gradients of stiffness, cell density, and immobilized peptide using thermogelling hydrogels. ACS Biomater Sci Eng. 2021;7(6):2192-2197. doi: 10.1021/acsbiomaterials.1c00183
- Giachini PAGS, Gupta SS, Wang W, et al. Additive manufacturing of cellulose-based materials with continuous, multidirectional stiffness gradients. Sci Adv. 2020;6(8):1-12. doi: 10.1126/sciadv.aay0929
- Bracaglia LG, Smith BT, Watson E, Arumugasaamy N, Mikos AG, Fisher JP. 3D printing for the design and fabrication of polymer-based gradient scaffolds. Acta Biomater. 2017;56:3-13. doi: 10.1016/j.actbio.2017.03.030
- Nadernezhad A, Khani N, Skvortsov GA, et al. Multifunctional 3D printing of heterogeneous hydrogel structures. Sci Rep. 2016;6(September):1-12. doi: 10.1038/srep33178
- Samandari M, Alipanah F, Majidzadeh-A K, Alvarez MM, Trujillo-De Santiago G, Tamayol A. Controlling cellular organization in bioprinting through designed 3D microcompartmentalization. Appl Phys Rev. 2021;8(2): 021404. doi: 10.1063/5.0040732
- Ceballos‐González CF, Bolívar‐Monsalve EJ, Quevedo‐ Moreno DA, et al. Plug‐and‐play multimaterial chaotic printing/bioprinting to produce radial and axial micropatterns in hydrogel filaments. Adv Mater Technol. 2023;8(17):2202208. doi: 10.1002/admt.202202208
- Snyder J, Son AR, Hamid Q, Wu H, Sun W. Hetero-cellular prototyping by synchronized multi-material bioprinting for rotary cell culture system. Biofabrication. 2016;8(1):015002. doi: 10.1088/1758-5090/8/1/015002
- Colosi C, Shin SR, Manoharan V, et al. Microfluidic bioprinting of heterogeneous 3D tissue constructs using low-viscosity bioink. Adv Mater. 2016;28(4):677-684. doi: 10.1002/adma.201503310
- Stroock AD, Dertinger SKW, Ajdari A, Mezić I, Stone HA, Whitesides GM. Chaotic mixer for microchannels. Science (1979). 2002;295(5555):647-651. doi: 10.1126/science.1066238
- Idaszek J, Costantini M, Karlsen TA, et al. 3D bioprinting of hydrogel constructs with cell and material gradients for the regeneration of full-thickness chondral defect using a microfluidic printing head. Biofabrication. 2019;11(4):044101. doi: 10.1088/1758-5090/ab2622
- Li M, Tian X, Zhu N, Schreyer DJ, Chen X. Modeling process-induced cell damage in the biodispensing process. Tissue Eng Part C Methods. 2010;16(3):533-542. doi: 10.1089/ten.TEC.2009.0178
- Metzner YC and AB. An analysis of apparent slip flow of polymer solutions. Rheol Acta. 1986;25:28-35.
- Reid JA, Mollica PA, Johnson GD, Ogle RC, Bruno RD, Sachs PC. Accessible bioprinting: Adaptation of a low-cost 3D-printer for precise cell placement and stem cell differentiation. Biofabrication. 2016;8(2):025017. doi: 10.1088/1758-5090/8/2/025017
- Li M, Tian X, Kozinski JA, Chen X, Hwang DK. Modeling mechanical cell damage in the bioprinting process employing a conical needle. J Mech Med Biol. 2015;15(05):1550073. doi: 10.1142/S0219519415500736
- Martanto W, Baisch SM, Costner EA, Prausnitz MR, Smith MK. Fluid dynamics in conically tapered microneedles. AIChE J. 2005;51(6):1599-1607. doi: 10.1002/aic.10424
- Leppiniemi J, Lahtinen P, Paajanen A, et al. 3D-printable bioactivated nanocellulose-alginate hydrogels. ACS Appl Mater Interfaces. 2017;9(26):21959-21970. doi: 10.1021/acsami.7b02756
- Liravi F, Darleux R, Toyserkani E. Additive manufacturing of 3D structures with non-Newtonian highly viscous fluids: finite element modeling and experimental validation. Addit Manuf. 2017;13:113-123. doi: 10.1016/j.addma.2016.10.008
- Billiet T, Gevaert E, De Schryver T, Cornelissen M, Dubruel P. The 3D printing of gelatin methacrylamide cell-laden tissue-engineered constructs with high cell viability. Biomaterials. 2014;35(1):49-62. doi: 10.1016/j.biomaterials.2013.09.078
- Lee KY, Kong HJ, Larson RG, Mooney DJ. Hydrogel formation via cell crosslinking. Adv Mater. 2003;15(21):1828-1832. doi: 10.1002/adma.200305406
- Park H, Kang SW, Kim BS, Mooney DJ, Lee KY. Shear-reversibly crosslinked alginate hydrogels for tissue engineering. Macromol Biosci. 2009;9(9):895-901. doi: 10.1002/mabi.200800376
- Das S, Chowdhury AR, Datta P. Modelling cell deformations in bioprinting process using a multicompartment-smooth particle hydrodynamics approach. Proc Inst Mech Eng H. 2022;236(6):867-881. doi: 10.1177/09544119221089720
- Müller M, Öztürk E, Arlov Ø, Gatenholm P, Zenobi- Wong M. Alginate sulfate–nanocellulose bioinks for cartilage bioprinting applications. Ann Biomed Eng. 2017;45(1):210-223. doi: 10.1007/s10439-016-1704-5
- Ates G, Bartolo P. Computational fluid dynamics for the optimization of internal bioprinting parameters and mixing conditions. Int J Bioprint. 2023;9(6):0219. doi: 10.36922/ijb.0219
- Chand R, Muhire BS, Vijayavenkataraman S. Computational fluid dynamics assessment of the effect of bioprinting parameters in extrusion bioprinting. Int J Bioprint. 2022;8(2):45-60. doi: 10.18063/ijb.v8i2.545
- Magalhães IP, de Oliveira PM, Dernowsek J, Casas EB Las, Casas MS Las. Investigation of the effect of nozzle design on rheological bioprinting properties using computational fluid dynamics. Revista Materia. 2019;24(3):12401. doi: 10.1590/s1517-707620190003.0714
- Li Y, Liu Y, Jiang C, Li S, Liang G, Hu Q. A reactor-like spinneret used in 3D printing alginate hollow fiber: a numerical study of morphological evolution. Soft Matter. 2016;12(8):2392-2399. doi: 10.1039/c5sm02733k
- Li S, Liu Y, Li Y, Zhang Y, Hu Q. Computational and experimental investigations of the mechanisms used by coaxial fluids to fabricate hollow hydrogel fibers. Chem Eng Process. Process Intensif. 2015;95:98-104. doi: 10.1016/j.cep.2015.05.018
- Nyande BW, Thomas MK, Lakerveld R. CFD analysis of a kenics static mixer with a low pressure drop under laminar flow conditions. Ind Eng Chem Res. 2021;60(14):5264-5277. doi: 10.1021/acs.iecr.1c00135
- Ates G, Bartolo P. Numerical simulation of multimaterial polymer mixing for bioprinting applications. J Addit Manuf Technol. 2021;1:12–14. doi: 10.18416/JAMTECH.2111606
- Blaeser A, Duarte Campos DF, Puster U, Richtering W, Stevens MM, Fischer H. Controlling shear stress in 3D bioprinting is a key factor to balance printing resolution and stem cell integrity. Adv Healthc Mater. 2016;5(3): 326-333. doi: 10.1002/adhm.201500677
- Mammoli AA, Brebbia CA, eds. Computational Methods in Multiphase Flow. Vol III. WIT; 2005.
- Ramezani H, Mohammad Mirjamali S, He Y. Simulations of extrusion 3D printing of chitosan hydrogels. Appl Sci. 2022;12(15):7530. doi: 10.3390/app12157530
- Emmermacher J, Spura D, Cziommer J, et al. Engineering considerations on extrusion-based bioprinting: interactions of material behavior, mechanical forces and cells in the printing needle. Biofabrication. 2020;12(2):025022. doi: 10.1088/1758-5090/ab7553
- Phan‐Thien N. A nonlinear network viscoelastic model. J Rheol. 1978;22(3):259-283. doi: 10.1122/1.549481
- Thien NP, Tanner RI. A new constitutive equation derived from network theory. J Nonnewton Fluid Mech. 1977;2(4):353-365. doi: 10.1016/0377-0257(77)80021-9
- Brackbill JU, Kothe DB, Zemach C. A continuum method for modeling surface tension. J Comput Phys. 1992;100(2):335-354. doi: 10.1016/0021-9991(92)90240-Y
- On the formulation of rheological equations of state. Proc R Soc Lond A Math Phys Sci. 1950;200(1063):523-541. doi: 10.1098/rspa.1950.0035
- Phan-Thien N, Mai-Duy N. Understanding Viscoelasticity. Springer International Publishing; 2017. doi: 10.1007/978-3-319-62000-8
- Mustapha SMFDS, Phillips TN. A dynamic nonlinear regression method for the determination of the discrete relaxation spectrum. J Phys D Appl Phys. 2000;33(10):1219-1229. doi: 10.1088/0022-3727/33/10/313