3D-printed devices for optimized generation of cold atmospheric plasma to improve decontamination of surfaces from respiratory pathogens
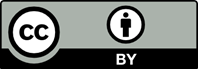
Three-dimensional (3D)-printing technology is instrumental in creating devices for biological applications, including the exploitation of cold atmospheric plasma (CAP). CAP, a partially ionized gas that functions at ambient temperatures, serves as a safe, inexpensive, and effective tool for the inactivation of various pathogens on different surfaces. In this study, we compared three different 3D-printed devices with respect to their ability to provide optimized CAP compositions effective against select respiratory viruses (SARS-CoV-2, influenza virus, adenovirus, and rhinovirus) and the bacterium Pseudomonas aeruginosa, which is associated with serious lung diseases. The transmission of respiratory pathogens via surface contamination may pose a serious health threat, thus highlighting the biological importance of the current study. The properties of a prototype 3D-printed CAP-generating device and two optimized versions were characterized by detecting reactive oxygen and nitrogen species (RONS) in a gaseous environment via infrared spectroscopy and analyzing the composition of the reactive compounds. The virucidal effects of CAP were examined by determining virus infectivity and particle integrity. The bactericidal effect was documented by viability testing and visualization via transmission electron microscopy. The findings indicate that optimization of the 3D-printed devices for CAP production yielded an environment with relatively high amounts of RONS (O3, N2O, NO2, and H2O2), reducing the exposure time required for inactivation of respiratory pathogens by approximately 50%. In addition to reducing infectivity and viability, CAP treatment led to the destruction of viral nucleic acids and physical damage to bacterial cells. Owing to its flexibility and easy implementation, optimized CAP generated by 3D-printed devices provides an attractive inactivation method adaptable for different biological applications, including surface decontamination from viral and bacterial pathogens.
- Fernández A, Noriega E, Thompson A. Inactivation of Salmonella enterica serovar Typhimurium on fresh produce by cold atmospheric gas plasma technology. Food Microbiol. 2013;33(1):24-29. doi: 10.1016/j.fm.2012.08.007
- Baier M, Görgen M, Ehlbeck J, Knorr D, Herppich WB, Schlüter O. Non-thermal atmospheric pressure plasma: Screening for gentle process conditions and antibacterial efficiency on perishable fresh produce. Innov Food Sci Emerg Technol. 2014;22:147-157. doi: 10.1016/j.ifset.2014.01.011
- Hertwig C, Reineke K, Ehlbeck J, Knorr D, Schlüter O. Decontamination of whole black pepper using different cold atmospheric pressure plasma applications. Food Control. 2015;55:221-229. doi: 10.1016/j.foodcont.2015.03.003
- Choi S, Puligundla P, Mok C. Corona discharge plasma jet for inactivation of Escherichia coli O157:H7 and Listeria monocytogenes on inoculated pork and its impact on meat quality attributes. Ann Microbiol. 2016;66(2):685-694. doi: 10.1007/S13213-015-1147-5
- Oh YJ, Song AY, Min SC. Inhibition of Salmonella typhimurium on radish sprouts using nitrogen-cold plasma. Int J Food Microbiol. 2017;249:66-71. doi: 10.1016/J.IJFOODMICRO.2017.03.005
- Van Gils CAJ, Hofmann S, Boekema BKHL, Brandenburg R, Bruggeman PJ. Mechanisms of bacterial inactivation in the liquid phase induced by a remote RF cold atmospheric pressure plasma jet. J Phys D Appl Phys. 2013;46(17):175203. doi: 10.1088/0022-3727/46/17/175203
- Alkawareek MY, Algwari QT, Laverty G, et al. Eradication of Pseudomonas aeruginosa Biofilms by Atmospheric Pressure Non-Thermal Plasma. PLoS One. 2012;7(8):44289. doi: 10.1371/JOURNAL.PONE.0044289
- Laroussi M. Low-temperature plasmas for medicine? IEEE Trans Plasma Sci. 2009;37(6 PART 1):714-725 doi: 10.1109/TPS.2009.2017267
- Moreau M, Orange N, Feuilloley MGJ. Non-thermal plasma technologies: new tools for bio-decontamination. Biotechnol Adv. 2008;26(6):610-617. doi: 10.1016/j.biotechadv.2008.08.001
- Kong MG, Kroesen G, Morfill G, et al. Plasma medicine: an introductory review. New J Phys. 2009;11(11):115012. doi: 10.1088/1367-2630/11/11/115012
- Nosenko T, Shimizu T, Morfill GE. Designing plasmas for chronic wound disinfection. New J Phys. 2009;11(11):115013. doi: 10.1088/1367-2630/11/11/115013
- Heinlin J, Isbary G, Stolz W, et al. Plasma applications in medicine with a special focus on dermatology. J Eur Acad Dermatology Venereol. 2011;25(1):1-11. doi: 10.1111/j.1468-3083.2010.03702.x
- Von Woedtke T, Haertel B, Weltmann KD, Lindequist U. Plasma pharmacy - physical plasma in pharmaceutical applications. Pharmazie. 2013;68(7):492-498. doi: 10.1691/ph.2013.6521
- von Woedtke T, Reuter S, Masur K, Weltmann KD. Plasmas for medicine. Phys Rep. 2013;530(4):291-320. doi: 10.1016/j.physrep.2013.05.005
- Theus AS, Ning L, Kabboul G, et al. 3D bioprinting of nanoparticle-laden hydrogel scaffolds with enhanced antibacterial and imaging properties. iScience. 2022;25(9):104947. doi: 10.1016/j.isci.2022.104947
- Liu H, Xing F, Yu P, et al. A review of biomacromolecule-based 3D bioprinting strategies for structure‒function integrated repair of skin tissues. Int J Biol Macromol. 2024;268(Pt 2). doi: 10.1016/J.IJBIOMAC.2024.131623
- Dubey A, Vahabi H, Kumaravel V. Antimicrobial and Biodegradable 3D Printed Scaffolds for Orthopedic Infections. ACS Biomater Sci Eng. 2023;9(7):4020-4044. doi: 10.1021/acsbiomaterials.3c00115
- Vaňková E, Kašparová P, Khun J, et al. Polylactic acid as a suitable material for 3D printing of protective masks in times of COVID-19 pandemic. PeerJ. 2020;8:1-20. doi: 10.7717/peerj.10259
- Obrová K, Vaňková E, Sláma M, et al. Decontamination of high-efficiency mask filters from respiratory pathogens including SARS-CoV-2 by non-thermal plasma. Front Bioeng Biotechnol. 2022;10(February):1-13. doi: 10.3389/fbioe.2022.815393
- Aboubakr HA, Williams P, Gangal U, et al. Virucidal effect of cold atmospheric gaseous plasma on feline calicivirus, a surrogate for human norovirus. Appl Environ Microbiol. 2015;81(11):3612-3622. doi: 10.1128/AEM.00054-15
- Ahlfeld B, Li Y, Boulaaba A, et al. Inactivation of a foodborne norovirus outbreak strain with nonthermal atmospheric pressure plasma. MBio. 2015;6(1). doi: 10.1128/MBIO.02300-14
- Bae SC, Park SY, Choe W, Ha S Do. Inactivation of murine norovirus-1 and hepatitis A virus on fresh meats by atmospheric pressure plasma jets. Food Res Int. 2015;76: 342-347. doi: 10.1016/j.foodres.2015.06.039
- Lacombe A, Niemira BA, Gurtler JB, et al. Nonthermal inactivation of norovirus surrogates on blueberries using atmospheric cold plasma. Food Microbiol. 2017;63:1-5. doi: 10.1016/j.fm.2016.10.030
- Nayak G, Aboubakr HA, Goyal SM, Bruggeman PJ. Reactive species responsible for the inactivation of feline calicivirus by a two-dimensional array of integrated coaxial microhollow dielectric barrier discharges in air. Plasma Process Polym. 2018;15(1):1700119. doi: 10.1002/PPAP.201700119
- Mohamed H, Nayak G, Rendine N, et al. Non-thermal plasma as a novel strategy for treating or preventing viral infection and associated disease. Front Phys. 2021;9(June):1-25. doi: 10.3389/fphy.2021.683118
- Assadi I, Guesmi A, Baaloudj O, et al. Review on inactivation of airborne viruses using nonthermal plasma technologies: from MS2 to coronavirus. Environ Sci Pollut Res. 2022;29:4880-4892. doi: 10.1007/s11356-021-17486-3
- Filipić A, Gutierrez-Aguirre I, Primc G, Mozetič M, Dobnik D. Cold Plasma, a new hope in the field of virus inactivation. Trends Biotechnol. 2020;38(11):1278-1291. doi: 10.1016/j.tibtech.2020.04.003
- Scholtz V, Vaňková E, Kašparová P, Premanath R, Karunasagar I, Julák J. Nonthermal plasma treatment of ESKAPE pathogens: a review. Front Microbiol. 2021;12(October):1-20. doi: 10.3389/fmicb.2021.737635
- Scholtz V, Julák J, Kříha V. The microbicidal effect of low-temperature plasma generated by corona discharge: comparison of various microorganisms on an agar surface or in aqueous suspension. Plasma Process Polym. 2010; 7(3-4):237-243. doi: 10.1002/ppap.200900072
- Mai-Prochnow A, Murphy AB, McLean KM, Kong MG, Ostrikov K. Atmospheric pressure plasmas: infection control and bacterial responses. Int J Antimicrob Agents. 2014;43(6):508-517. doi: 10.1016/J.IJANTIMICAG.2014.01.025
- Aboubakr HA, Mor SK, Higgins L, et al. Cold argon-oxygen plasma species oxidize and disintegrate capsid protein of feline calicivirus. PLoS One. 2018;13(3):e0194618. doi: 10.1371/journal.pone.0194618
- Aboubakr HA, Nauertz A, Luong NT, et al. In vitro antiviral activity of clove and ginger aqueous extracts against feline calicivirus, a surrogate for human norovirus. J Food Prot. 2016;79(6):1001-1012. doi: 10.4315/0362-028X.JFP-15-593
- Xu D, Ning N, Xu Y, et al. Effect of cold atmospheric plasma treatment on the metabolites of human leukemia cells. Cancer Cell Int. 2019;19(1):1-12. doi: 10.1186/s12935-019-0856-4
- Zimmermann JL, Dumler K, Shimizu T, et al. Effects of cold atmospheric plasmas on adenoviruses in solution. J Phys D Appl Phys. 2011;44(50):505201. doi: 10.1088/0022-3727/44/50/505201
- Sakudo A, Toyokawa Y, Imanishi Y, Murakami T. Crucial roles of reactive chemical species in modification of respiratory syncytial virus by nitrogen gas plasma. Mater Sci Eng C. 2017;74:131-136. doi: 10.1016/j.msec.2017.02.007
- Sakudo A, Misawa T, Shimizu N, Imanishi Y. N₂ gas plasma inactivates influenza virus mediated by oxidative stress. Front Biosci (Elite Ed). 2014;6(1):69-79. doi: 10.2741/E692
- Sakudo A, Toyokawa Y, Imanishi Y. Nitrogen gas plasma generated by a static induction thyristor as a pulsed power supply inactivates adenovirus. PLoS One. 2016;11(6):e0157922. doi: 10.1371/JOURNAL.PONE.0157922
- Alkawareek MY, Algwari QT, Gorman SP, Graham WG, O’Connell D, Gilmore BF. Application of atmospheric pressure nonthermal plasma for the in vitro eradication of bacterial biofilms. FEMS Immunol Med Microbiol. 2012;65(2):381-384. doi: 10.1111/j.1574-695X.2012.00942.x
- Haertel B, Woedtke T von, Weltmann KD, Lindequist U. Non-thermal atmospheric-pressure plasma possible application in wound healing. Biomol Ther. 2014;22(6):477-490. doi: 10.4062/biomolther.2014.105
- Guo L, Xu R, Gou L, et al. Mechanism of virus inactivation by cold atmospheric-pressure plasma and plasma-activated water. Appl Environ Microbiol. 2018;84(17):1-10. doi: 10.1128/AEM.00726-18
- Dolezalova E, Lukes P. Membrane damage and active but nonculturable state in liquid cultures of Escherichia coli treated with an atmospheric pressure plasma jet. Bioelectrochemistry. 2015;103:7-14. doi: 10.1016/J.BIOELECHEM.2014.08.018
- Haas M, Fürhacker P, Hodek J, et al. Detection of viable SARS-CoV-2 on the hands of hospitalized children with COVID-19. Clin Microbiol Infect. 2023;29(9):1211-1213. doi: 10.1016/J.CMI.2023.06.012
- Nogueira F, Obrova K, Haas M, et al. Intestinal shedding of SARS-CoV-2 in children: no evidence for infectious potential. Microorganisms. 2023;11(1):33. doi: 10.3390/microorganisms11010033
- Lion T. Adenovirus persistence, reactivation, and clinical management. FEBS Lett. 2019;593(24):3571-3582. doi: 10.1002/1873-3468.13576
- Lion T, Wold W. Adenoviruses. In: Knipe D, Howley P, eds. Chapter in Fields Virology, 7th ed. Wolters Kluver Health / Lippincott, Williams Wilkins 2022, 129-171. https://www.livres-medicaux.com/infectiologie-virologie-bacteriologie/23602-fields-virology-dna-viruses-vol-2-7th-ed.html. Accessed July 25, 2023.
- Leung NHL. Transmissibility and transmission of respiratory viruses. Nat Rev Microbiol. 2021;19(8):528-545. doi: 10.1038/s41579-021-00535-6
- Kirtipal N, Bharadwaj S, Gu S. From SARS to SARS-CoV-2, insights on structure, pathogenicity and immunity aspects of pandemic human coronaviruses. Infect Genet Evol. 2020;85(January):15. doi: 10.1016/j.meegid.2020.104502
- Kumar V. Influenza in children. Indian J Pediatr. 2017;84(2):139-143. doi: 10.1007/s12098-016-2232-x
- Lion T. Adenovirus infections in immunocompetent and immunocompromised patients. Clin Microbiol Rev. 2014;27(3):441-462. doi: 10.1128/CMR.00116-13
- Piotrowska Z, Vázquez M, Shapiro ED, et al. Rhinoviruses are a major cause of wheezing and hospitalization in children less than 2 years of age. Pediatr Infect Dis J. 2009;28(1):25-29. doi: 10.1097/INF.0b013e3181861da0
- Fazeli N, Momtaz H. Virulence gene profiles of multidrug-resistant Pseudomonas aeruginosa isolated from Iranian hospital infections. Iran Red Crescent Med J. 2014;16(10). doi: 10.5812/ircmj.15722
- Ranjan Prasad R, Shree V, Kumar R, Kala K, Kumar P. Prevalence and antibiotic sensitivity of Pseudomonas aeruginosa isolated from CSOM in NMCH, Patna, India. Int J Curr Microbiol Appl Sci. 2017;6(6):2912-2916. doi: 10.20546/ijcmas.2017.606.345
- Khun J, Machková A, Kašparová P, et al. Non-thermal plasma sources based on cometary and point-to-ring discharges. Molecules. 2022;27(1):238. doi: 10.3390/molecules27010238
- Kašparová P, Vaňková E, Paldrychová M, et al. Nonthermal plasma causes Pseudomonas aeruginosa biofilm release to planktonic form and inhibits production of Las-B elastase, protease and pyocyanin. Front Cell Infect Microbiol. 2022;12(September):1-16. doi: 10.3389/fcimb.2022.993029
- Ngaosuwankul N, Noisumdaeng P, Komolsiri P, et al. Research influenza A viral loads in respiratory samples collected from patients infected with pandemic H1N1, seasonal H1N1 and H3N2 viruses. Virol J. 2010;7(1):1-7. doi: 10.1186/1743-422X-7-75/TABLES/4
- Machala Z, Tarabová B, Sersenová D, Janda M, Hensel K. Chemical and antibacterial effects of plasma activated water: correlation with gaseous and aqueous reactive oxygen and nitrogen species, plasma sources and air flow conditions. J Phys D Appl Phys. 2019;52(3):034002. doi: 10.1088/1361-6463/aae807
- Kučerová K, Machala Z, Hensel K. Transient spark discharge generated in various N2/O2 gas mixtures: reactive species in the gas and water and their antibacterial effects. Plasma Chem Plasma Process. 2020;40(3):749-773. doi: 10.1007/s11090-020-10082-2
- Lukes P, Dolezalova E, Sisrova I, Clupek M. Aqueous-phase chemistry and bactericidal effects from an air discharge plasma in contact with water: evidence for the formation of peroxynitrite through a pseudo-second-order postdischarge reaction of H2O2 and HNO2. Plasma Sources Sci Technol. 2014;23(1):015019. doi: 10.1088/0963-0252/23/1/015019
- Wang Z, Liu L, Liu D, et al. Combination of NOx mode and O3 mode air discharges for water activation to produce a potent disinfectant. Plasma Sources Sci Technol. 2022;31(5):05LT01. doi: 10.1088/1361-6595/ac60c0
- Dasan BG, Onal-Ulusoy B, Pawlat J, Diatczyk J, Sen Y, Mutlu M. A New and Simple Approach for Decontamination of Food Contact Surfaces with Gliding Arc Discharge Atmospheric Non-Thermal Plasma. Food Bioprocess Technol. 2017;10(4):650-661. doi: 10.1007/S11947-016-1847-2
- Belgacem Z Ben, Carre G, Charpentier E, et al. Innovative nonthermal plasma disinfection process inside sealed bags: Assessment of bactericidal and sporicidal effectiveness in regard to current sterilization norms. PLoS One. 2017;12(6):e0180183. doi: 10.1371/JOURNAL.PONE.0180183
- Lunov O, Zablotskii V, Churpita O, et al. The interplay between biological and physical scenarios of bacterial death induced by nonthermal plasma. Biomaterials. 2016; 82:71-83. doi: 10.1016/J.BIOMATERIALS.2015.12.027
- Scholtz V, Pazlarova J, Souskova H, Khun J, Julak J. Nonthermal plasma---a tool for decontamination and disinfection. Biotechnol Adv. 2015;33(6 Pt 2):1108-1119. doi: 10.1016/J.BIOTECHADV.2015.01.002
- Alkawareek MY, Gorman SP, Graham WG, Gilmore BF. Potential cellular targets and antibacterial efficacy of atmospheric pressure nonthermal plasma. Int J Antimicrob Agents. 2014;43(2):154-160. doi: 165.
- Jablonowski H, Hänsch MAC, Dünnbier M, et al. Plasma jet’s shielding gas impact on bacterial inactivation. Biointerphases. 2015;10(2):029506. doi: 10.1116/1.4916533
- Machala Z, Tarabova B, Hensel K, Spetlikova E, Sikurova L, Lukes P. Formation of ROS and RNS in water electro-sprayed through transient spark discharge in air and their bactericidal effects. Plasma Process Polym. 2013;10(7):649-659. doi: 10.1002/PPAP.201200113
- Oehmigen K, Hähnel M, Brandenburg R, Wilke C, Weltmann KD, Von Woedtke T. The role of acidification for antimicrobial activity of atmospheric pressure plasma in liquids. Plasma Process Polym. 2010;7(3-4):250-257. doi: 10.1002/PPAP.200900077
- Liu Z, Xu D, Liu D, et al. Production of simplex RNS and ROS by nanosecond pulse N2/O2 plasma jets with homogeneous shielding gas for inducing myeloma cell apoptosis. J Phys D Appl Phys. 2017;50(19):195204. doi: 10.1088/1361-6463/aa66f0
- Ke Z, Thopan P, Fridman G, et al. Effect of N2/O2 composition on inactivation efficiency of Escherichia coli by discharge plasma at the gas-solution interface. Clin Plasma Med. 2017;7-8:1-8. doi: 10.1016/j.cpme.2017.05.001
- Thurston-Enriquez JA, Haas CN, Jacangelo J, Riley K, Gerba CP. Inactivation of Feline Calicivirus and Adenovirus Type 40 by UV Radiation. Appl Environ Microbiol. 2003;69(1): 577-582. doi: 10.1128/AEM.69.1.577-582.2003
- Von Woedtke T, Laroussi M, Gherardi M. Foundations of plasmas for medical applications. Plasma Sources Sci Technol. 2022;31(5):054002. doi: 10.1088/1361-6595/AC604F
- Bisag A, Isabelli P, Laurita R, et al. Cold atmospheric plasma inactivation of aerosolized microdroplets containing bacteria and purified SARS-CoV-2 RNA to contrast airborne indoor transmission. Plasma Process Polym. 2020;17(10):1-8. doi: 10.1002/ppap.202000154
- Kobza J, Geremek M, Dul L. Ozone concentration levels in urban environments—upper Silesia region case study. Int J Environ Res Public Heal 2021, Vol 18, Page 1473. 2021;18(4):1473. doi: 10.3390/IJERPH18041473
- Wolfgruber S, Loibner M, Puff M, Melischnig A, Zatloukal K. SARS-CoV-2 neutralizing activity of ozone on porous and nonporous materials. N Biotechnol. 2022;66:36-45. doi: 10.1016/j.nbt.2021.10.001
- Mileto D, Mancon A, Staurenghi F, et al. Inactivation of SARS-CoV-2 in the liquid phase: are aqueous hydrogen peroxide and sodium percarbonate efficient decontamination agents? ACS Chem Heal Saf. 2021;28(4):260-267. doi: 10.1021/acs.chas.0c00095
- Mentel R, Schirrmacher R, Kewitsch A. Inactivation of viruses with hydrogen dioxide. Vopr Virusol. 1977;22(6): 731-733. Accessed March 30, 2023. http://www.ncbi.nlm.nih.gov/pubmed/203115
- Amanna IJ, Raué HP, Slifka MK. Development of a new hydrogen peroxide–based vaccine platform. Nat Med 2012 186. 2012;18(6):974-979. doi: 10.1038/nm.2763
- Termini J. Hydroperoxide-induced DNA damage and mutations. Mutat Res Mol Mech Mutagen. 2000; 450(1-2):107-124. doi: 10.1016/S0027-5107(00)00019-1
- Wang H, Zhao T, Yang S, Zou L, Wang X, Zhang Y. Reactive force field-based molecular dynamics simulation of the interaction between plasma reactive oxygen species and the receptor-binding domain of the spike protein in the capsid protein of SARS-CoV-2. J Phys D Appl Phys. 2021;55(9):095401. doi: 10.1088/1361-6463/AC360E
- Sahun M, Privat-Maldonado A, Lin A, et al. Inactivation of SARS-CoV-2 and other enveloped and non-enveloped viruses with non-thermal plasma for hospital disinfection. ACS Sustain Chem Eng. 2023;11(13):5206-5215. doi: 10.1021/acssuschemeng.2c07622