In situ bioprinting: Tailored printing strategies for regenerative medicine
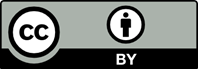
In recent years, three-dimensional (3D) bioprinting has emerged as a revolutionary biological manufacturing technology. Despite significant progress, current bioprinting technologies face critical barriers, such as the need for in vitro maturation of printed tissues before implantation and challenges of prefabricated structures not matching the defect shapes. In situ bioprinting has been introduced to address these challenges by printing customized structures to the wound shape via direct deposition of biological inks at the tissue interface. This paper reviews strategies to optimize printing performance for enhanced tissue repair and analyzes the advantages, challenges, and future directions of in situ bioprinting technologies.
- Li L, Yu F, Shi J, et al. In situ repair of bone and cartilage defects using 3D scanning and 3D printing. Sci Rep. 2017;7(1):9416. doi: 10.1038/s41598-017-10060-3
- Singh S, Choudhury D, Yu F, Mironov V, Naing MW. In situ bioprinting—bioprinting from benchside to bedside? Acta Biomater. 2020;101:14-25. doi: 10.1016/j.actbio.2019.08.045
- Ozbolat IT. Bioprinting scale-up tissue and organ constructs for transplantation. Trends Biotechnol. 2015;33(7): 395-400. doi: 10.1016/j.tibtech.2015.04.005
- Gatenholm B, Lindahl C, Brittberg M, Simonsson S. Collagen 2A type B induction after 3D bioprinting chondrocytes in situ into osteoarthritic chondral tibial lesion. Cartilage. 2021;13(2_SUPPL):1755S-1769S. doi: 10.1177/1947603520903788
- Yang BW, Yin JH, Chen Y, et al. 2D-black-phosphorus-reinforced 3D-printed scaffolds: a stepwise countermeasure for osteosarcoma. Adv Mater. 2018;30(10):1705611. doi: 10.1002/adma.201705611
- Chow T, Wutami I, Lucarelli E, et al. Creating in vitro three-dimensional tumor models: a guide for the biofabrication of a primary osteosarcoma model. Tissue Eng Part B Rev. 2021;27(5):514-529. doi: 10.1089/ten.teb.2020.0254
- Li ZH, Zhao Y, Wang ZH, et al. Engineering multifunctional hydrogel-integrated 3D printed bioactive prosthetic interfaces for osteoporotic osseointegration. Adv Healthc Mater. 2022;11(11):e2102535. doi: 10.1002/adhm.202102535
- Yvanoff C, Willaert RG. Development of bone cell microarrays in microfluidic chips for studying osteocyte-osteoblast communication under fluid flow mechanical loading. Biofabrication. 2022;14(2):025014. doi: 10.1088/1758-5090/ac516e
- Cheng RY, Eylert G, Gariepy J-M, et al. Handheld instrument for wound-conformal delivery of skin precursor sheets improves healing in full-thickness burns. Biofabrication. 2020;12(2):025002. doi: 10.1088/1758-5090/ab6413
- Jiao T, Lian Q, Lian W, et al. Properties of collagen/sodium alginate hydrogels for bioprinting of skin models. J Bionic Eng. 2023;20(1):105-118. doi: 10.1007/s42235-022-00251-8
- Liu J, Zhou Z, Zhang M, et al. Simple and robust 3D bioprinting of full-thickness human skin tissue. Bioengineered. 2022;13(4):10087-10097. doi: 10.1080/21655979.2022.2063651
- Mandrycky C, Wang Z, Kim K, Kim D-H. 3D bioprinting for engineering complex tissues. Biotechnol Adv. 2016;34(4):422-434. doi: 10.1016/j.biotechadv.2015.12.011
- Sithole MN, Kumar P, du Toit LC, et al. A 3D bioprinted in situ conjugated-co-fabricated scaffold for potential bone tissue engineering applications. J Biomed Mater Res Part A. 2018;106(5):1311-1321. doi: 10.1002/jbm.a.36333
- Kilian D, Sembdner P, Bretschneider H, et al. 3D printing of patient-specific implants for osteochondral defects: workflow for an MRI-guided zonal design. Bio-Des Manuf. 2021;4(4):818-832. doi: 10.1007/s42242-021-00153-4
- Kurzyk A, Szumera-Cieckiewicz A, Miloszewska J, Chechlinska M. 3D modeling of normal skin and cutaneous squamous cell carcinoma. A comparative study in 2D cultures, spheroids, and 3D bioprinted systems. Biofabrication. 2024;16(2):025021. doi: 10.1088/1758-5090/ad2b06.
- Fu Z, Hai N, Zhong Y, Sun W. Printing GelMA bioinks: a strategy for building in vitro model to study nanoparticle-based minocycline release and cellular protection under oxidative stress. Biofabrication. 2024;16(2):025040. doi: 10.1088/1758-5090/ad30c3
- Shukla P, Bera AK, Yeleswarapu S, Pati F. High throughput bioprinting using decellularized adipose tissue-based hydrogels for 3D breast cancer modeling. Macromol Biosci. 2024;24:2400035. doi: 10.1002/mabi.202400035
- Wu D, Pang S, Berg J, et al. Bioprinting of perfusable vascularized organ models for drug development via sacrificial-free direct ink writing. Adv Funct Mater. 2024:2314171. doi: 10.1002/adfm.202314171
- Gvaramia D, Fisch P, Flegeau K, et al. Evaluation of bioprinted autologous cartilage grafts in an immunocompetent rabbit model. Adv Ther. 2024;7(6):2300441. doi: 10.1002/adtp.202300441
- Bhar B, Das E, Manikumar K, Mandal BB. 3D bioprinted human skin model recapitulating native-like tissue maturation and immunocompetence as an advanced platform for skin sensitization assessment. Adv Healthc Mater. 2024;13(15):e2303312. doi: 10.1002/adhm.202303312
- Di Buduo CA, Lunghi M, Kuzmenko V, et al. Bioprinting soft 3D models of hematopoiesis using natural silk fibroin-based bioink efficiently supports platelet differentiation. Adv Sci. 2024;11(18):2308276. doi: 10.1002/advs.202308276
- Edri S, Frisch AN, Safina D, et al. 3D bioprinting of multicellular stem cell-derived constructs to model pancreatic cell differentiation. Adv Funct Mater. 2024;34:2315488. doi: 10.1002/adfm.202315488
- Li H, Cheng F, Orgill DP, Yao J, Zhang YS. Handheld bioprinting strategies for in situ wound dressing. In: Jang J, ed. Essays in Biochemistry. Vol. 65. London: Portland Press; 2021:533-543. doi: 10.1042/EBC20200098
- Thai MT, Phan PT, Tran HA, et al. Advanced soft robotic system for in situ 3D bioprinting and endoscopic surgery. Adv Sci. 2023;10(12):2205656. doi: 10.1002/advs.202205656
- Agostinacchio F, Mu X, Dire S, Motta A, Kaplan DL. In situ 3D printing: opportunities with silk inks. Trends Biotechnol. 2021;39(7):719-730. doi: 10.1016/j.tibtech.2020.11.003
- Akilbekova D, Mektepbayeva D. Chapter 5-patient specific in situ 3D printing. In: Kalaskar DM, ed. 3D Printing in Medicine. Cambridge: Woodhead Publishing; 2017:91-113. doi: 10.1016/b978-0-08-100717-4.00004-1
- Campbell PG, Weiss LE. Tissue engineering with the aid of inkjet printers. Expert Opin Biol Ther. 2007;7(8):1123-1127. doi: 10.1517/14712598.7.8.1123
- Samandari M, Mostafavi A, Quint J, Memic A, Tamayol A. In situ bioprinting: intraoperative implementation of regenerative medicine. Trends Biotechnol. 2022;40(10): 1229-1247. doi: 10.1016/j.tibtech.2022.03.009
- Zhao W, Xu T. Preliminary engineering for in situ in vivo bioprinting: a novel micro bioprinting platform for in situ in vivo bioprinting at a gastric wound site. Biofabrication. 2020;12(4):045020. doi: 10.1088/1758-5090/aba4ff
- Nuutila K, Samandari M, Endo Y, et al. In vivo printing of growth factor-eluting adhesive scaffolds improves wound healing. Bioact Mater. 2022;8:296-308. doi: 10.1016/j.bioactmat.2021.06.030
- Wu Y, Ravnic DJ, Ozbolat IT. Intraoperative bioprinting: repairing tissues and organs in a surgical setting. Trends Biotechnol. 2020;38(6):594-605. doi: 10.1016/j.tibtech.2020.01.004
- Chen H, Zhang Y, Zhou D, et al. Mechanical engineering of hair follicle regeneration by in situ bioprinting. Biomater Adv. 2022;142:213127. doi: 10.1016/j.bioadv.2022.213127
- Gaharwar AK, Singh I, Khademhosseini A. Engineered biomaterials for in situ tissue regeneration. Nat Rev Mater. 2020;5(9):686-705. doi: 10.1038/s41578-020-0209-x
- Levin AA, Karalkin PA, V. Koudan E, et al. Commercial articulated collaborative in situ 3D bioprinter for skin wound healing. Int J Bioprint. 2023;9(2):380-393. doi: 10.18063/ijb.v9i2.675
- Li L, Shi J, Ma K, et al. Robotic in situ 3D bio-printing technology for repairing large segmental bone defects. J Adv Res. 2021;30:75-84. doi: 10.1016/j.jare.2020.11.011
- Moncal KK, Gudapati H, Godzik KP, et al. Intra-operative bioprinting of hard, soft, and hard/soft composite tissues for craniomaxillofacial reconstruction. Adv Funct Mater. 2021;31(29):2010858. doi: 10.1002/adfm.202010858
- Albanna M, Binder KW, Murphy SV, et al. In situ bioprinting of autologous skin cells accelerates wound healing of extensive excisional full-thickness wounds. Sci Rep. 2019;9(1):1856 doi: 10.1038/s41598-018-38366-w
- Zhao W, Hu C, Xu T, et al. Subaqueous bioprinting: a novel strategy for fetal membrane repair with 7-axis robot-assisted minimally invasive surgery. Adv Funct Mater. 2022;32(51):2207496. doi: 10.1002/adfm.202207496
- Christensen K, Compaan A, Chai W, Xia G, Huang Y. In situ printing-then-mixing for biological structure fabrication using intersecting jets. ACS Biomater Sci Eng. 2017;3(12):3687-3694. doi: 10.1021/acsbiomaterials.7b00752
- Keriquel V, Oliveira H, Remy M, et al. In situ printing of mesenchymal stromal cells, by laser-assisted bioprinting, for in vivo bone regeneration applications. Sci Rep. 2017;7(1):1778. doi: 10.1038/s41598-017-01914-x
- Kerouredan O, Hakobyan D, Remy M, et al. In situ prevascularization designed by laser-assisted bioprinting: effect on bone regeneration. Biofabrication. 2019;11(4):045002. doi: 10.1088/1758-5090/ab2620
- Ding H, Chang RC. Simulating image-guided in situ bioprinting of a skin graft onto a phantom burn wound bed. Addit Manuf. 2018;22:708-719. doi: 10.1016/j.addma.2018.06.022
- Liu Y, Luo X, Wu W, et al. Dual cure (thermal/photo) composite hydrogel derived from chitosan/collagen for in situ 3D bioprinting. Int J Biol Macromol. 2021;182:689-700. doi: 10.1016/j.ijbiomac.2021.04.058
- Chen Y, Zhang J, Liu X, et al. Noninvasive in vivo 3D bioprinting. Sci Adv. 2020;6(23):eaba7406. doi: 10.1126/sciadv.aba7406
- Urciuolo A, Poli I, Brandolino L, et al. Intravital three-dimensional bioprinting. Nat Biomed Eng. 2020;4(9):901-915. doi: 10.1038/s41551-020-0568-z
- Chen H, Zhang H, Shen Y, et al. Instant in-situ tissue repair by biodegradable PLA/gelatin nanofibrous membrane using a 3D printed handheld electrospinning device. Front Bioeng Biotechnol. 2021;9:684105. doi: 10.3389/fbioe.2021.684105
- Tianyuan Y, Yi Z, Zhian J, Yuanyuan L. A novel handheld device: application to in situ bioprinting compound dressing for the treatment of wound. Paper presented at: Journal of Physics: Conference Series; 2021. doi: 10.1088/1742-6596/1965/1/012059
- Li X, Lian Q, Li DC, Xin H, Jia SH. Development of a robotic arm based hydrogel additive manufacturing system for in-situ printing. Appl Sci. 2017;7(1):73. doi: 10.3390/app7010073
- Keriquel V, Guillemot F, Arnault I, et al. In vivo bioprinting for computer-and robotic-assisted medical intervention: preliminary study in mice. Biofabrication. 2010;2(1):014101. doi: 10.1088/1758-5082/2/1/014101
- Zhu Z, Guo S-Z, Hirdler T, et al. 3D printed functional and biological materials on moving freeform surfaces. Adv Mater. 2018;30(23):e1707495. doi: 10.1002/adma.201707495
- Adib AA, Sheikhi A, Shahhosseini M, et al. Direct-write 3D printing and characterization of a GelMA-based biomaterial for intracorporeal tissue engineering. Biofabrication. 2020;12(4):045006. doi: 10.1088/1758-5090/ab97a1
- Skardal A, Mack D, Kapetanovic E, et al. Bioprinted amniotic fluid-derived stem cells accelerate healing of large skin wounds. Stem Cells Transl Med. 2012;1(11):792-802. doi: 10.5966/sctm.2012-0088
- Russell CS, Mostafavi A, Quint JP, et al. In situ printing of adhesive hydrogel scaffolds for the treatment of skeletal muscle injuries. ACS Appl Bio Mater. 2020;3(3):1568-1579. doi: 10.1021/acsabm.9b01176
- Quint JP, Mostafavi A, Endo Y, et al. In vivo printing of nanoenabled scaffolds for the treatment of skeletal muscle injuries. Adv Healthc Mater. 2021;10(10):e2002152. doi: 10.1002/adhm.202002152
- Di Bella C, Duchi S, O’Connell CD, et al. In situ handheld three-dimensional bioprinting for cartilage regeneration. J Tissue Eng Regen Med. 2018;12(3):611-621. doi: 10.1002/term.2476
- Duchi S, Onofrillo C, O’Connell CD, et al. Handheld co-axial bioprinting: application to in situ surgical cartilage repair. Sci Rep. 2017;7(1):5837. doi: 10.1038/s41598-017-05699-x
- Onofrillo C, Duchi S, O’Connell CD, et al. Biofabrication of human articular cartilage: a path towards the development of a clinical treatment. Biofabrication. 2018;10(4):045006. doi: 10.1088/1758-5090/aad8d9
- Mostafavi A, Abdullah T, Russell CS, et al. In situ printing of scaffolds for reconstruction of bone defects. Acta Biomater. 2021;127:313-326. doi: 10.1016/j.actbio.2021.03.009
- Campos DFD, Zhang S, Kreimendahl F, et al. Hand-held bioprinting for de novo vascular formation applicable to dental pulp regeneration. Connect Tissue Res. 2020;61(2):205-215. doi: 10.1080/03008207.2019.1640217
- Zhou C, Yang Y, Wang J, et al. Ferromagnetic soft catheter robots for minimally invasive bioprinting. Nat Commun. 2021;12(1):5072. doi: 10.1038/s41467-021-25386-w
- Abdelrahim AA, Hong S, Song JM. Integrative in situ photodynamic therapy-induced cell death measurement of 3D-bioprinted MCF-7 tumor spheroids. Anal Chem. 2022;94(40):13936-13943. doi: 10.1021/acs.analchem.2c03022
- Chaudhry MS, Czekanski A. Surface slicing and toolpath planning for in-situ bioprinting of skin implants. Biofabrication. 2024;16(2):025030. doi: 10.1088/1758-5090/ad30c4
- Zhao M, Wang J, Zhang J, et al. Functionalizing multi-component bioink with platelet-rich plasma for customized in-situ bilayer bioprinting for wound healing. Mater Today Bio. 2022;16:100334. doi: 10.1016/j.mtbio.2022.100334
- Liu X, Wang X, Zhang L, et al. 3D liver tissue model with branched vascular networks by multimaterial bioprinting. Adv Healthcare Mater. 2021;10(23):e2101405. doi: 10.1002/adhm.202101405
- Zhao W, Chen H, Zhang Y, et al. Adaptive multi-degree-of-freedom in situ bioprinting robot for hair-follicle-inclusive skin repair: A preliminary study conducted in mice. Bioeng Transl Med. 2022;7(3):e10303. doi: 10.1002/btm2.10303
- Ma K, Zhao T, Yang L, et al. Application of robotic-assisted in situ 3D printing in cartilage regeneration with HAMA hydrogel: an in vivo study. J Adv Res. 2020;23:123-132. doi: 10.1016/j.jare.2020.01.010
- Al-Kharusi G, Dunne NJ, Little S, Levingstone TJ. The role of machine learning and design of experiments in the advancement of biomaterial and tissue engineering research. Bioengineering (Basel). 2022;9(10):561. doi: 10.3390/bioengineering9100561
- Albouy M, Desanlis A, Brosset S, et al. A preliminary study for an intraoperative 3D bioprinting treatment of severe burn injuries. Plast Reconstr Surg Glob Open. 2022;10(1):e4056. doi: 10.1097/GOX.0000000000004056
- Chen H, Ma X, Gao T, et al. Robot-assisted in situ bioprinting of gelatin methacrylate hydrogels with stem cells induces hair follicle-inclusive skin regeneration. Biomed Pharmacother. 2023;158:114140. doi: 10.1016/j.biopha.2022.114140
- Simeunovic A, Wolf K, Tierling K, Hoelzle DJ. A surgical robot for intracorporeal additive manufacturing of tissue engineering constructs. IEEE Rob Autom Lett. 2022;7(3):7495-7502. doi: 10.1109/LRA.2022.3183752
- Fortunato GM, Sigismondi S, Nicoletta M, et al. Analysis of the robotic-based in situ bioprinting workflow for the regeneration of damaged tissues through a case study. Bioengineering (Basel). 2023;10(5):560. doi: 10.3390/bioengineering10050560
- Colosi C, Costantini M, Barbetta A, Dentini M. Microfluidic bioprinting of heterogeneous 3D tissue constructs. Methods Mol Biol. 2017;1612:369-380. doi: 10.1007/978-1-4939-7021-6_26
- Xie M, Shi Y, Zhang C, et al. In situ 3D bioprinting with bioconcrete bioink. Nat Commun. 2022;13(1):3597. doi: 10.1038/s41467-022-30997-y
- Shi E, Lou L, Warburton L, Rubinsky B. Three-dimensional printing in combined cartesian and curvilinear coordinates. J Med Device. 2022;16(4):044502. doi: 10.1115/1.4055064
- Fortunato GM, Batoni E, Pasqua I, et al. Automatic photo-cross-linking system for robotic-based in situ bioprinting. ACS Biomater Sci Eng. 2023;9(12):6926-6934. doi: 10.1021/acsbiomaterials.3c00898
- Kucukdeger E, Johnson BN. Closed-loop controlled conformal 3D printing on moving objects via tool-localized object position sensing. J Manuf Processes. 2023;89:39-49. doi: 10.1016/j.jmapro.2023.01.020.
- Yang S, Wang L, Chen Q, Xu M. In situ process monitoring and automated multi-parameter evaluation using optical coherence tomography during extrusion-based bioprinting. Addit Manuf. 2021;47:102251. doi: 10.1016/j.addma.2021.102251
- Yang S, Chen Q, Wang L, Xu M. In situ defect detection and feedback control with three-dimensional extrusion-based bioprinter-associated optical coherence tomography. Int J Bioprint. 2023;9(1):47-62. doi: 10.18063/ijb.v9i1.624
- Tashman JW, Shiwarski DJ, Coffin B, et al. In situ volumetric imaging and analysis of FRESH 3D bioprinted constructs using optical coherence tomography. Biofabrication. 2023;15(1):014102. doi: 10.1088/1758-5090/ac975e
- Kerouredan O, Ribot E, Fricain J-C, Devillard R, Miraux S. Magnetic resonance imaging for tracking cellular patterns obtained by laser-assisted bioprinting. Sci Rep. 2018;8(1):15777. doi: 10.1038/s41598-018-34226-9
- Zhou Y, Liao S, Chu Y, et al. An injectable bioink with rapid prototyping in the air and in-situ mild polymerization for 3D bioprinting. Biofabrication. 2021;13(4):045026. doi: 10.1088/1758-5090/ac23e4
- Kotlarz M, Ferreira AM, Gentile P, Russell SJ, Dalgarno K. Droplet-based bioprinting enables the fabrication of cell-hydrogel-microfibre composite tissue precursors. Bio-Des Manuf. 2022;5(3):512-528. doi: 10.1007/s42242-022-00192-5
- Zhao D-k, Xu H-q, Yin J, Yang H-y. Inkjet 3D bioprinting for tissue engineering and pharmaceutics. J Zhejiang Univ Sci A. 2022;23(12):955-973. doi: 10.1631/2023.A2200569
- Limon SM, Quigley C, Sarah R, Habib A. Advancing scaffold porosity through a machine learning framework in extrusion based 3D bioprinting. Front Mater. 2024;10:1337485. doi: 10.3389/fmats.2023.1337485
- Qiao Q, Zhang X, Yan Z, et al. The use of machine learning to predict the effects of cryoprotective agents on the GelMA-based bioinks used in extrusion cryobioprinting. Bio-Des Manuf. 2023;6(4):464-477. doi: 10.1007/s42242-023-00244-4
- Huang X, Ng WL, Yeong WY. Predicting the number of printed cells during inkjet-based bioprinting process based on droplet velocity profile using machine learning approaches. J Intell Manuf. 2023;35(5). doi: 10.1007/s10845-023-02167-4
- Pagan E, Stefanek E, Seyfoori A, et al. A handheld bioprinter for multi-material printing of complex constructs. Biofabrication. 2023;15(3):035012. doi: 10.1088/1758-5090/acc42c.
- Hakimi N, Cheng R, Leng L, et al. Handheld skin printer: in situ formation of planar biomaterials and tissues. Lab Chip. 2018;18(10):1440-1451. doi: 10.1039/c7lc01236e.
- Zhou FY, Xin LJ, Wang SY, et al. Portable handheld “SkinPen” loaded with biomaterial ink for in situ wound healing. ACS Appl Mater Interfaces. 2023;15(23):27568-27585. doi: 10.1021/acsami.3c02825
- Ying G, Manriquez J, Wu D, et al. An open-source handheld extruder loaded with pore-forming bioink for in situ wound dressing. Mater Today Bio. 2020;8:100074. doi: 10.1016/j.mtbio.2020.100074
- Mostafavi A, Samandari M, Karvar M, et al. Colloidal multiscale porous adhesive (bio)inks facilitate scaffold integration. Appl Phys Rev. 2021;8(4):041415. doi: 10.1063/5.0062823
- Shi Y, Tang S, Yuan X, et al. In situ 4D printing of polyelectrolyte/magnetic composites for sutureless gastric perforation sealing. Adv Mater. 2023;36:2307601. doi: 10.1002/adma.202307601
- Yang Y, Yu Z, Lu X, et al. Minimally invasive bioprinting for in situ liver regeneration. Bioact Mater. 2023;26:465-477. doi: 10.1016/j.bioactmat.2023.03.011
- Debbi L, Machour M, Dahis D, et al. Ultrasound mediated polymerization for cell delivery, drug delivery, and 3D printing. Small Methods. 2024:2301197. doi: 10.1002/smtd.202301197
- Zhao W, Hu C, Lin S, et al. A closed-loop minimally invasive 3D printing strategy with robust trocar identification and adaptive alignment. Addit Manuf. 2023;73:103701. doi: 10.1016/j.addma.2023.103701
- Goker M, Derici US, Gokyer S, et al. Spatial growth factor delivery for 3D bioprinting of vascularized bone with adipose-derived stem/stromal cells as a single cell source. ACS Biomater Sci Eng. 2024;10(3):1607-1619. doi: 10.1021/acsbiomaterials.3c01222
- Enrico A, Voulgaris D, Ostmans R, et al. 3D microvascularized tissue models by laser-based cavitation molding of collagen. Adv Mater. 2022;34(11):e2109823. doi: 10.1002/adma.202109823
- Hu Y, Xiong Y, Zhu Y, et al. Copper-epigallocatechin gallate enhances therapeutic effects of 3D-printed dermal scaffolds in mitigating diabetic wound scarring. ACS Appl Mater Interfaces. 2023;15(32):38230-38246. doi: 10.1021/acsami.3c04733
- Lu S, Wang X, Li W, Zu Y, Xiao J. Injectable 3D-printed porous scaffolds for adipose stem cell delivery and endometrial regeneration. Adv Funct Mater. 2023;33(34): 2303368. doi: 10.1002/adfm.202303368
- Ouyang L, Armstrong JPK, Chen Q, Lin Y, Stevens MM. Void-free 3D bioprinting for in situ endothelialization and microfluidic perfusion. Adv Funct Mater. 2020;30(1):1908349. doi: 10.1002/adfm.201908349
- Muhammad M, Willems C, Rodriguez-Fernandez J, Gallego-Ferrer G, Groth T. Synthesis and characterization of oxidized polysaccharides for in situ forming hydrogels. Biomolecules. 2020;10(8):1185. doi: 10.3390/biom10081185
- Baptista M, Joukhdar H, Alcala-Orozco CR, et al. Silk fibroin photo-lyogels containing microchannels as a biomaterial platform for in situ tissue engineering. Biomater Sci. 2020;8(24):7093-7105. doi: 10.1039/d0bm01010c
- Chen X, Ranjan VD, Liu S, et al. In situ formation of 3D conductive and cell-laden graphene hydrogel for electrically regulating cellular behavior. Macromol Biosci. 2021;21(4):e2000374. doi: 10.1002/mabi.202000374
- Li C, Faulkner-Jones A, Dun AR, et al. Rapid formation of a supramolecular polypeptide-DNA hydrogel for in situ three-dimensional multilayer bioprinting. Angew Chem Int Ed. 2015;54(13):3957-3961. doi: 10.1002/anie.201411383
- Moeinzadeh S, Park Y, Lin S, Yang YP. In-situ stable injectable collagen-based hydrogels for cell and growth factor delivery. Materialia (Oxf). 2021;15:100954. doi: 10.1016/j.mtla.2020.100954
- Alruwaili M, Lopez JA, McCarthy K, Reynaud EG, Rodriguez BJ. Liquid-phase 3D bioprinting of gelatin alginate hydrogels: influence of printing parameters on hydrogel line width and layer height. Bio-Des Manuf. 2019;2(3):172-180. doi: 10.1007/s42242-019-00043-w
- Handral HK, Natu VP, Cao T, et al. Emerging trends and prospects of electroconductive bioinks for cell-laden and functional 3D bioprinting. Bio-Des Manuf. 2022;5(2): 396-411. doi: 10.1007/s42242-021-00169-w
- Lai S, Wu T, Shi C, et al. Triple-layered core-shell fiber dressings with enduring platelet conservation and sustained growth factor release abilities for chronic wound healing. Regener Biomater. 2024;11:rbae034. doi: 10.1093/rb/rbae034
- Kang MS, Kwon M, Lee SY, et al. In situ crosslinkable collagen-based hydrogels for 3D printing of dermis-mimetic constructs. ECS J Solid State Sci Technol. 2022;11(4): 045014. doi: 10.1149/2162-8777/ac6897
- Kang MS, Kang JI, Thi PL, et al. Three-dimensional printable gelatin hydrogels incorporating graphene oxide to enable spontaneous myogenic differentiation. ACS Macro Lett. 2021;10(4):426-432. doi: 10.1021/acsmacrolett.0c00845
- Miri AK, Nieto D, Iglesias L, et al. Microfluidics-enabled multimaterial maskless stereolithographic bioprinting. Adv Mater. 2018;30(27):e1800242. doi: 10.1002/adma.201800242
- Hwangbo H, Lee H, Jin E-J, et al. Bio-printing of aligned GelMa-based cell-laden structure for muscle tissue regeneration. Bioact Mater. 2021;8:57-70. doi: 10.1016/j.bioactmat.2021.06.031
- Jalandhra GK, Molley TG, Hung T-t, Roohani I, Kilian KA. In situ formation of osteochondral interfaces through “bone-ink” printing in tailored microgel suspensions. Acta Biomater. 2023;156:75-87. doi: 10.1016/j.actbio.2022.08.052
- de Melo BAG, Jodat YA, Mehrotra S, et al. 3D printed cartilage-like tissue constructs with spatially controlled mechanical properties. Adv Funct Mater. 2019;29(51):1906330. doi: 10.1002/adfm.201906330
- Li C, Wang J, Yang W, et al. 3D-printed hydrogel particles containing PRP laden with TDSCs promote tendon repair in a rat model of tendinopathy. J Nanobiotechnol. 2023;21(1):177. doi: 10.1186/s12951-023-01892-5
- Li J, Huang Y, Song J, et al. Cartilage regeneration using arthroscopic flushing fluid-derived mesenchymal stem cells encapsulated in a one-step rapid cross-linked hydrogel. Acta Biomater. 2018;79:202-215. doi: 10.1016/j.actbio.2018.08.029
- Blaeser A, Million N, Campos DFD, et al. Laser-based in situ embedding of metal nanoparticles into bioextruded alginate hydrogel tubes enhances human endothelial cell adhesion. Nano Res. 2016;9(11):3407-3427. doi: 10.1007/s12274-016-1218-3
- Khoshnood N, Shahrezaee MH, Shahrezaee M, Zamanian A. Three-dimensional bioprinting of tragacanth/ hydroxyapaptite modified alginate bioinks for bone tissue engineering with tunable printability and bioactivity. J Appl Polym Sci. 2022;139(36):e52833. doi: 10.1002/app.52833
- Bandyopadhyay A, Mandal BB, Bhardwaj N. 3D bioprinting of photo-crosslinkable silk methacrylate (SilMA)-polyethylene glycol diacrylate (PEGDA) bioink for cartilage tissue engineering. J Biomed Mater Res Part A. 2022;110(4):884-898. doi: 10.1002/jbm.a.37336
- Zhang M, Qian T, Deng Z, Hang F. 3D printed double-network alginate hydrogels containing polyphosphate for bioenergetics and bone regeneration. Int J Biol Macromol. 2021;188:639-648. doi: 10.1016/j.ijbiomac.2021.08.066
- Koo Y, Kim G. New strategy for enhancing in situ cell viability of cell-printing process via piezoelectric transducer-assisted three-dimensional printing. Biofabrication. 2016;8(2):025010. doi: 10.1088/1758-5090/8/2/025010
- Krishnadoss V, Kanjilal B, Masoumi A, et al. Programmable bio-ionic liquid functionalized hydrogels for in situ 3D bioprinting of electronics at the tissue interface. Mater Today Adv. 2023;17:100352. doi: 10.1016/j.mtadv.2023.100352
- Yin J, Zhao D, Liu J. Trends on physical understanding of bioink printability. Bio-Des Manuf. 2019;2(1):50-54. doi: 10.1007/s42242-019-00033-y
- Wang X, Yang C, Yu Y, Zhao Y. In situ 3D bioprinting living photosynthetic scaffolds for autotrophic wound healing. Research (Wash D C). 2022;2022:9794745. doi: 10.34133/2022/9794745
- Hann SY, Cui H, Esworthy T, Zhang LG. 4D thermo-responsive smart hiPSC-CM cardiac construct for myocardial cell therapy. Int J Nanomed. 2023;18:1809-1821. doi: 10.2147/IJN.S402855
- Joshi A, Choudhury S, Baghel VS, et al. 4D printed programmable shape-morphing hydrogels as intraoperative self-folding nerve conduits for sutureless neurorrhaphy. Adv Healthcare Mater. 2023;12(24):e2300701. doi: 10.1002/adhm.202300701
- Liu B, Li H, Meng F, et al. 4D printed hydrogel scaffold with swelling-stiffening properties and programmable deformation for minimally invasive implantation. Nat Commun. 2024;15(1):1587. doi: 10.1038/s41467-024-45938-0
- Luo K, Wang L, Wang M-X, et al. 4D printing of biocompatible scaffolds via in situ photo-crosslinking from shape memory copolyesters. ACS Appl Mater Interfaces. 2023;15(37):44373-44383. doi: 10.1021/acsami.3c10747
- Li W, Wang M, Mille LS, et al. A smartphone-enabled portable digital light processing 3D printer. Adv Mater. 2021;33(35):2102153. doi: 10.1002/adma.202102153
- Warth N, Berg M, Schumacher L, et al. Bioprint FirstAid: a handheld bioprinter for first aid utilization on space exploration missions. Acta Astronaut. 2024;215:194-204. doi: 10.1016/j.actaastro.2023.11.033
- Tomooka Y, Spothelfer D, Puiggali-Jou A, et al. Minimally invasive in situ bioprinting using tube-based material transfer. at - Automatisierungstechnik. 2023;71(7):562-571. doi: 10.1515/auto-2023-0060