Stereolithography 3D printing of microgroove master moulds for topography-induced nerve guidance conduits
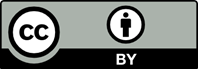
Patients who have peripheral nerve damage from trauma or disease may suffer lifelong disability. Current interventions such as nerve allografts are inadequate due to limited availability of tissue and donor-site morbidity. Commercial nerve guidance conduits are used to bridge the damaged nerve gap and restore function. Typically, however, they lack cell-instructive guidance cues to promote directed regeneration. Tissue-engineered nerve guidance conduits that utilise micro- and nano-topographical architectures have been demonstrated to direct cell behaviour and contact guidance. This study uses projection micro-stereolithography-based three-dimensional (3D) printing to fabricate microgrooved (10–30 μm) master moulds to produce polydimethylsiloxane (PDMS) moulds and solvent cast polycaprolactone and polylactic acid films. The polymer microgrooves were successfully fabricated and were able to be formed into tubular nerve guidance conduits. The surface morphology, roughness, wettability, and thermal properties of the films were characterised. The microgroove topography improved proliferation and induced alignment of SH-SY5Y cells. This facile 3D printing approach is promising for the fabrication of nerve guidance conduits with topographical guidance cues as it obviates the need for using photolithographic techniques. Thus, this approach provides an alternative that is simpler, faster, cheaper, and offers greater design freedom.
- Lackington WA, Ryan AJ, O’Brien FJ. Advances in nerve guidance conduit-based therapeutics for peripheral nerve repair. ACS Biomater Sci Eng. 2017;3(7):1221-1235. doi: 10.1021/acsbiomaterials.6b00500
- Bryan DJ, Tang JB, Doherty SA, et al. Enhanced peripheral nerve regeneration through a poled bioresorbable poly (lactic-co-glycolic acid) guidance channel. J Neural Eng. 2004;1(2):91. doi: 10.1088/1741-2560/1/2/004
- Slavin BR, Sarhane KA, von Guionneau N, et al. Insulin-like growth factor-1: a promising therapeutic target for peripheral nerve injury. Front Bioeng Biotechnol. 2021;9:695850. doi: 10.3389/fbioe.2021.695850
- Pabari A, Yang SY, Seifalian AM, Mosahebi A. Modern surgical management of peripheral nerve gap. J Plast Reconstr Aesthet Surg. 2010;63(12):1941-1948. doi: 10.1016/j.bjps.2009.12.010
- Vijayavenkataraman S. Nerve guide conduits for peripheral nerve injury repair: a review on design, materials and fabrication methods. Acta Biomater. 2020;106:54-69. doi: 10.1016/j.actbio.2020.02.003
- Wang ML, Rivlin M, Graham JG, Beredjiklian PK. Peripheral nerve injury, scarring, and recovery. Connect Tissue Res. 2019;60(1):3-9. doi: 10.1080/03008207.2018.1489381
- Siemionow M, Brzezicki G. Chapter 8: Current techniques and concepts in peripheral nerve repair. Int Rev Neurobiol. 2009;87:141-172. doi: 10.1016/S0074-7742(09)87008-6
- Deumens R, Bozkurt A, Meek MF, et al. Repairing injured peripheral nerves: bridging the gap. Prog Neurobiol. 2010;92(3):245-276. doi: 10.1016/j.pneurobio.2010.10.002
- Suo H, Wang Z, Dai G, Fu J, Yin J, Chang L. Polyacrylonitrile nerve conduits with inner longitudinal grooved textures to enhance neuron directional outgrowth. J Microelectromech Syst. 2018;27(3):457-463. doi: 10.1109/JMEMS.2018.2810097
- Taylor CS, Haycock JW. Biomaterials and scaffolds for repair of the peripheral nervous system. In: Phillips JB, Hercher D, Hausner T, eds. Peripheral Nerve Tissue Engineering and Regeneration. Cham: Springer; 2022: 245-279. doi: 10.1007/978-3-030-21052-6_3
- Wangensteen KJ, Kalliainen LK. Collagen tube conduits in peripheral nerve repair: a retrospective analysis. Hand. 2010;5(3):273-277. doi: 10.1007/s11552-009-9245-0
- Spivey EC, Khaing ZZ, Shear JB, Schmidt CE. The fundamental role of subcellular topography in peripheral nerve repair therapies. Biomaterials. 2012;33(17): 4264-4276. doi: 10.1016/j.biomaterials.2012.02.043
- Kehoe S, Zhang XF, Boyd D. FDA approved guidance conduits and wraps for peripheral nerve injury: a review of materials and efficacy. Injury. 2012;43(5):553-572. doi: 10.1016/j.injury.2010.12.030
- Papadimitriou L, Manganas P, Ranella A, Stratakis E. Biofabrication for neural tissue engineering applications. Mater Today Bio. 2020;6:100043. doi: 10.1016/j.mtbio.2020.100043
- Tonda‐Turo C, Gnavi S, Ruini F, et al. Development and characterization of novel agar and gelatin injectable hydrogel as filler for peripheral nerve guidance channels. J Tissue Eng Regen Med. 2017;11(1):197-208. doi: 10.1002/term.1902
- Du J, Liu J, Yao S, et al. Prompt peripheral nerve regeneration induced by a hierarchically aligned fibrin nanofiber hydrogel. Acta Biomater. 2017;55:296-309. doi: 10.1016/j.actbio.2017.04.010
- Ma Y, Wang H, Wang Q, Cao X, Gao H. Piezoelectric conduit combined with multi-channel conductive scaffold for peripheral nerve regeneration. Chem Eng J. 2023;452: 139424. doi: 10.1016/j.cej.2022.139424
- Sharma AD, Zbarska S, Petersen EM, Marti ME, Mallapragada SK, Sakaguchi DS. Oriented growth and transdifferentiation of mesenchymal stem cells towards a Schwann cell fate on micropatterned substrates. J Biosci Bioeng. 2016;121(3):325-335. doi: 10.1016/j.jbiosc.2015.07.006
- Long Y, Zhang N, Huang Y, Wen X. Formation of highly aligned grooves on inner surface of semipermeable hollow fiber membrane for directional axonal outgrowth. J Manuf Sci Eng. 2008;130(2):021011. doi: 10.1115/1.2896111
- Yang CY, Huang WY, Chen LH, et al. Neural tissue engineering: the influence of scaffold surface topography and extracellular matrix microenvironment. J Mater Chem B. 2021;9(3):567-584. doi: 10.1039/d0tb01605e
- Ma Y, Gao H, Wang H, Cao X. Engineering topography: effects on nerve cell behaviors and applications in peripheral nerve repair. J Mater Chem B. 2021;9(32):6310-6325. doi: 10.1039/d1tb00782c
- Zhang D, Wu S, Feng J, Duan Y, Xing D, Gao C. Micropatterned biodegradable polyesters clicked with CQAASIKVAV promote cell alignment, directional migration, and neurite outgrowth. Acta Biomater. 2018;74:143-155. doi: 10.1016/j.actbio.2018.05.018
- Miller C, Jeftinija S, Mallapragada S. Micropatterned Schwann cell-seeded biodegradable polymer substrates significantly enhance neurite alignment and outgrowth. Tissue Eng. 2001;7(6):705-715. doi: 10.1089/107632701753337663
- Sun M, Kingham PJ, Reid AJ, Armstrong SJ, Terenghi G, Downes S. In vitro and in vivo testing of novel ultrathin PCL and PCL/PLA blend films as peripheral nerve conduit. J Biomed Mater Res Part A. 2010;93(4):1470-1481. doi: 10.1002/jbm.a.32681
- Hwang CM, Park Y, Park JY, et al. Controlled cellular orientation on PLGA microfibers with defined diameters. Biomed Microdevices. 2009;11(4):739-746. doi: 10.1007/s10544-009-9287-7
- Hsu SH, Lu PS, Ni HC, Su CH. Fabrication and evaluation of microgrooved polymers as peripheral nerve conduits. Biomed Microdevices. 2007;9:665-674. doi: 10.1007/s10544-007-9068-0
- Béduer A, Vieu C, Arnauduc F, Sol JC, Loubinoux I, Vaysse L. Engineering of adult human neural stem cells differentiation through surface micropatterning. Biomaterials. 2012;33(2):504-514. doi: 10.1016/j.biomaterials.2011.09.073
- Lu S, Chen W, Wang J, et al. Polydopamine-decorated PLCL conduit to induce synergetic effect of electrical stimulation and topological morphology for peripheral nerve regeneration. Small Methods. 2023;7(2):e2200883. doi: 10.1002/smtd.202200883
- Mobasseri A, Faroni A, Minogue BM, Downes S, Terenghi G, Reid AJ. Polymer scaffolds with preferential parallel grooves enhance nerve regeneration. Tissue Eng Part A. 2015;21(5-6):1152-1162. doi: 10.1089/ten.TEA.2014.0266
- Mobasseri SA, Terenghi G, Downes S. Micro-structural geometry of thin films intended for the inner lumen of nerve conduits affects nerve repair. J Mater Sci Mater Med. 2013;24(7):1639-1647. doi: 10.1007/s10856-013-4922-5
- Hsu SH, Ni HC. Fabrication of the microgrooved/ microporous polylactide substrates as peripheral nerve conduits and in vivo evaluation. Tissue Eng Part A. 2009;15(6):1381-1390. doi: 10.1089/ten.tea.2008.0175
- Litowczenko J, Maciejewska BM, Wychowaniec JK, Koscinski M, Jurga S, Warowicka A. Groove-patterned surfaces induce morphological changes in cells of neuronal origin. J Biomed Mater Res A. 2019;107(10):2244-2256. doi: 10.1002/jbm.a.36733
- Yu S, Liu D, Wang T, Lee YZ, Wong JCN, Song X. Micropatterning of polymer substrates for cell culture. J Biomed Mater Res B Appl Biomater. 2021;109(10): 1525-1533. doi: 10.1002/jbm.b.34811
- Pardo-Figuerez M, Martin NRW, Player DJ, et al. Controlled arrangement of neuronal cells on surfaces functionalized with micropatterned polymer brushes. ACS Omega. 2018;3(10):12383-12391. doi: 10.1021/acsomega.8b01698
- Nam KH, Jamilpour N, Mfoumou E, Wang FY, Zhang DD, Wong PK. Probing mechanoregulation of neuronal differentiation by plasma lithography patterned elastomeric substrates. Sci Rep. 2014;4(1):6965. doi: 10.1038/srep06965
- Klein CL, Scholl M, Maelicke A. Neuronal networks in vitro: formation and organization on biofunctionalized surfaces. J Mater Sci Mater Med. 1999;10(12):721-727. doi: 10.1023/a:1008975105243
- Qin D, Xia Y, Whitesides GM. Soft lithography for micro-and nanoscale patterning. Nat Protoc. 2010;5(3):491-502. doi: 10.1038/nprot.2009.234
- Miller C, Shanks H, Witt A, Rutkowski G, Mallapragada S. Oriented Schwann cell growth on micropatterned biodegradable polymer substrates. Biomaterials. 2001;22(11):1263-1269. doi: 10.1016/s0142-9612(00)00278-7
- Ge Q, Li Z, Wang Z, et al. Projection micro stereolithography based 3D printing and its applications. Int J Extreme Manuf. 2020;2(2):022004. doi: 10.1088/2631-7990/ab8d9a
- Evangelista MS, Perez M, Salibian AA, et al. Single-lumen and multi-lumen poly(ethylene glycol) nerve conduits fabricated by stereolithography for peripheral nerve regeneration in vivo. J Reconstr Microsurg. 2015;31(5): 327-335. doi: 10.1055/s-0034-1395415
- Singh A, Asikainen S, Teotia AK, et al. Biomimetic photocurable three-dimensional printed nerve guidance channels with aligned cryomatrix lumen for peripheral nerve regeneration. ACS Appl Mater Interfaces. 2018;10(50): 43327-43342. doi: 10.1021/acsami.8b11677
- Pateman CJ, Harding AJ, Glen A, et al. Nerve guides manufactured from photocurable polymers to aid peripheral nerve repair. Biomaterials. 2015;49:77-89. doi: 10.1016/j.biomaterials.2015.01.055
- Moreno-Rivas O, Hernández-Velázquez D, Piazza V, Marquez S. Rapid prototyping of microfluidic devices by SL 3D printing and their biocompatibility study for cell culturing. Mater Today Proc. 2019;13:436-445. doi: 10.1016/j.matpr.2019.03.189
- Villegas M, Cetinic Z, Shakeri A, Didar TF. Fabricating smooth PDMS microfluidic channels from low-resolution 3D printed molds using an omniphobic lubricant-infused coating. Anal Chim Acta. 2018;1000:248-255. doi: 10.1016/j.aca.2017.11.063
- Jonathan Tjong AGT, John PF. Solvent extraction of 3D printed molds for soft lithography. R Soc Chem. 2020
- Schneider CA, Rasband WS, Eliceiri KW. NIH image to ImageJ: 25 years of image analysis. Nat Methods. 2012;9(7):671-675. doi: 10.1038/nmeth.2089
- Vertuccio L, Gorrasi G, Sorrentino A, Vittoria V. Nano clay reinforced PCL/starch blends obtained by high energy ball milling. Carbohydr Polym. 2009;75(1):172-179. doi: 10.1016/j.carbpol.2008.07.020
- Tonazzini I, Cecchini A, Elgersma Y, Cecchini M. Interaction of SH-SY5Y cells with nanogratings during neuronal differentiation: comparison with primary neurons. Adv Healthc Mater. 2014;3(4):581-587. doi: 10.1002/adhm.201300216
- Alvim Valente C, Cesar Chagastelles P, Fontana Nicoletti N, et al. Design and optimization of biocompatible polycaprolactone/poly (l-lactic-co-glycolic acid) scaffolds with and without microgrooves for tissue engineering applications. J Biomed Mater Res Part A. 2018;106(6): 1522-1534. doi: 10.1002/jbm.a.36355
- Carmona VB, Corrêa AC, Marconcini JM, Mattoso LHC. Properties of a biodegradable ternary blend of thermoplastic starch (TPS), poly (ε-caprolactone)(PCL) and poly (lactic acid)(PLA). J Polym Environ. 2015;23(1): 83-89. doi: 10.1007/s10924-014-0666-7
- Vieille B, Albouy W, Chevalier L, Taleb L. About the influence of stamping on thermoplastic-based composites for aeronautical applications. Compos Part B: Eng. 2013;45(1):821-834. doi: 10.1016/j.compositesb.2012.07.047
- Kostakova E, Mészáros L, Maskova G, Blazkova L, Turcsan T, Lukas D. Crystallinity of electrospun and centrifugal spun polycaprolactone fibers: a comparative study. J Nanomater. 2017;1-9. doi: 10.1155/2017/8952390
- Huang B, Wang Y, Vyas C, Bartolo P. Crystal growth of 3D poly(ε-caprolactone) based bone scaffolds and its effects on the physical properties and cellular interactions. Adv Sci. 2023;10(1):2203183. doi: 10.1002/advs.202203183
- Sun M, Downes S. Physicochemical characterisation of novel ultra-thin biodegradable scaffolds for peripheral nerve repair. J Mater Sci Mater Med. 2009;20(5):1181-1192. doi: 10.1007/s10856-008-3671-3
- Dong J, Liu J, Li X, Liang Q, Xu X. Relationship between the Young’s modulus and the crystallinity of cross‐linked poly (ε‐caprolactone) as an immobilization membrane for cancer radiotherapy. Glob Chall. 2020;4(8):2000008. doi: 10.1002/gch2.202000008
- Borschel GH, Kia KF, Kuzon WM, Jr., Dennis RG. Mechanical properties of acellular peripheral nerve. J Surg Res. 2003;114(2):133-139. doi: 10.1016/s0022-4804(03)00255-5
- Rydevik BL, Kwan MK, Myers RR, et al. An in vitro mechanical and histological study of acute stretching on rabbit tibial nerve. J Orthop Res. 1990;8(5):694-701. doi: 10.1002/jor.1100080511
- Kerns J, Piponov H, Helder C, Amirouche F, Solitro G, Gonzalez M. Mechanical properties of the human tibial and peroneal nerves following stretch with histological correlations. Anat Rec. 2019;302(11):2030-2039. doi: 10.1002/ar.24250
- Rahmati M, Silva EA, Reseland JE, Heyward CA, Haugen HJ. Biological responses to physicochemical properties of biomaterial surface. Chem Soc Rev. 2020;49(15):5178-5224. doi: 10.1039/D0CS00103A
- Patr T, Glória A. Mechanical and biological behaviour of PCL and PCL/PLA scaffolds for tissue engineering applications. Chem Eng Trans. 2013;32:1645-1650. doi: 10.3303/CET1332275
- Zhang H, Guo J, Wang Y, Shang L, Chai R, Zhao Y. Natural polymer‐derived bioscaffolds for peripheral nerve regeneration. Adv Funct Mater. 2022;32(41):2203829. doi: 10.1002/adfm.202203829