Treatment effects of 3D-printed PCL/Fe3O4@ ZIF-8 magnetic nanocomposite on infected bone defect
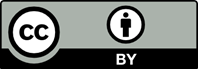
Treatment of large bone defects remains a clinical challenge, especially for defects compounded by infection. It is essential to develop dual functional therapeutic systems that inhibit bacterial growth and promote bone regeneration for infected bone defects treatment. However, the ideal bone substitute biomaterials to repair infected bone defects remain scarce. In this study, we fabricated a novel magnetic nanocomposite with polycaprolactone (PCL)/Fe3O4@zinc-based imidazole zeolite framework-8 (ZIF-8) through three-dimensional (3D) printing technology. The biomaterial characterization, biocompatibility, antibacterial activity, and osteogenic ability of the 3D-printed PCL/Fe3O4@ZIF-8 nanocomposite were systematically investigated in vivo and in vitro. The 3D-printed PCL/Fe3O4@ZIF-8 nanocomposite scaffolds showed a square porous grid structure with rough surface, thermal stability, superparamagnetic character, and slow release of Zn2+. The elevation of Fe3O4@ZIF-8 concentration increased surface roughness and porosity, improved the mechanical properties, and enhanced the saturation magnetization of PCL/Fe3O4@ZIF-8 scaffolds. The PCL/Fe3O4@ZIF-8 scaffolds possessed good biocompatibility and promoted the proliferation and adhesion of rat bone marrow mesenchymal stem cells (BMSCs). The PCL/Fe3O4@ZIF-8 scaffolds also upregulated the expression of osteogenic-related genes and proteins and promoted the osteogenic differentiation of BMSCs by activating the Wnt/β-catenin signaling pathway. Furthermore, the scaffolds showed excellent antibacterial activities, which increased with increasing Fe3O4@ ZIF-8 nanoparticle concentration. In vivo experiments proved that the scaffolds eliminated infection and promoted new bone formation in infected bone defect. Given excellent osteogenic and antibacterial activities, the 3D-printed PCL/Fe3O4@ZIF-8 nanocomposite scaffolds could serve as novel materials for the treatment of infected bone defects.
- Yang Y, Chu L, Yang S, et al. Dual-functional 3D-printed composite scaffold for inhibiting bacterial infection and promoting bone regeneration in infected bone defect models. Acta Biomater. 2018;79:265-275. doi: 10.1016/j.actbio.2018.08.015
- Wang W, Yeung KWK. Bone grafts and biomaterials substitutes for bone defect repair: a review. Bioact Mater. 2017;2(4):224-247. doi: 10.1016/j.bioactmat.2017.05.007
- Kawecki F, Clafshenkel WP, Fortin M, Auger FA, Fradette J. Biomimetic tissue-engineered bone substitutes for maxillofacial and craniofacial repair: the potential of cell sheet technologies. Adv Healthc Mater. 2018;7(6): e1700919. doi: 10.1002/adhm.201700919
- Baki A, Wiekhorst F, Bleul R. Advances in magnetic nanoparticles engineering for biomedical applications-a review. Bioengineering (Basel). 2021;8(10):134. doi: 10.3390/bioengineering8100134
- Cardoso VF, Francesko A, Ribeiro C, Bañobre-López M, Martins P, Lanceros-Mendez S. Advances in magnetic nanoparticles for biomedical applications. Adv Healthc Mater. 2018;7(5). doi: 10.1002/adhm.201700845
- Díaz E, Valle MB, Ribeiro S, Lanceros‑Mendez S, Barandiarán JM. A new approach for the fabrication of cytocompatible PLLA-magnetite nanoparticle composite scaffolds. Int J Mol Sci. 2019;20(19):4664. doi: 10.3390/ijms20194664
- Mollarasouli F, Zor E, Ozcelikay G, Ozkan SA. Magnetic nanoparticles in developing electrochemical sensors for pharmaceutical and biomedical applications. Talanta. 2021;226:122108. doi: 10.1016/j.talanta.2021.122108
- Xia Y, Sun J, Zhao L, et al. Magnetic field and nano-scaffolds with stem cells to enhance bone regeneration. Biomaterials. 2018;183:151-170. doi: 10.1016/j.biomaterials.2018.08.040
- Sun J, Fan F, Wang P, Ma S, Song L, Gu N. Orientation-dependent thermogenesis of assembled magnetic nanoparticles in the presence of an alternating magnetic field. Chemphyschem. 2016;17(21):3377-3384. doi: 10.1002/cphc.201600787
- Wu K, Su D, Liu J, Saha R, Wang J-P. Magnetic nanoparticles in nanomedicine: a review of recent advances. Nanotechnology. 2019;30(50):502003. doi: 10.1088/1361-6528/ab4241
- Wang N, Xie Y, Xi Z, et al. Hope for bone regeneration: the versatility of iron oxide nanoparticles. Front Bioeng Biotechnol. 2022;10:937803. doi: 10.3389/fbioe.2022.937803
- Díaz E, Valle MB, Ribeiro S, Lanceros-Méndez S, Barandiaran JM. Development of magnetically active scaffolds for bone regeneration. Nanomaterials (Basel). 2018;8(9):678. doi: 10.3390/nano8090678
- Díaz E, Blanca Valle MLSGALG, Ribeiro S, Lanceros- Mendez S, Barandiarán JM. 3D cytocompatible composites of PCL/magnetite. Materials (Basel). 2019;12(23):3843. doi: 10.3390/ma12233843
- Zhang J, Zhao S, Zhu M, et al. 3D-printed magnetic Fe3O4/ MBG/PCL composite scaffolds with multifunctionality of bone regeneration, local anticancer drug delivery and hyperthermia. J Mater Chem B. 2014;2(43):7583-7595. doi: 10.1039/c4tb01063a
- Qi J, Zhang J, Jia H, et al. Synthesis of silver/Fe3O4@ chitosan@polyvinyl alcohol magnetic nanoparticles as an antibacterial agent for accelerating wound healing. Int J Biol Macromol. 2022;221:1404-1414. doi: 10.1016/j.ijbiomac.2022.09.030
- Wang N, Liang H, Zen K. Molecular mechanisms that influence the macrophage m1-m2 polarization balance. Front Immunol. 2014;5:614. doi: 10.3389/fimmu.2014.00614
- Xia X, Song X, Li Y, et al. Antibacterial and anti-inflammatory ZIF-8@Rutin nanocomposite as an efficient agent for accelerating infected wound healing. Front Bioeng Biotechnol. 2022;10:1026743. doi: 10.3389/fbioe.2022.1026743
- Liu Y, Zhu Z, Pei X, et al. ZIF-8-modified multifunctional bone-adhesive hydrogels promoting angiogenesis and osteogenesis for bone regeneration. ACS Appl Mater Interfaces. 2020;12(33):36978-36995. doi: 10.1021/acsami.0c12090
- Zheng X, Zhang Y, Zou L, et al. Robust ZIF-8/alginate fibers for the durable and highly effective antibacterial textiles. Colloids Surf B Biointerfaces. 2020;193:111127. doi: 10.1016/j.colsurfb.2020.111127
- Xu B, Wang H, Wang W, et al. A single-atom nanozyme for wound disinfection applications. Angew Chem Int Ed Engl. 2019;58(15):4911-4916. doi: 10.1002/anie.201813994
- Schejn A, Mazet T, Falk V, et al. Fe3O4@ZIF-8: magnetically recoverable catalysts by loading Fe3O4 nanoparticles inside a zinc imidazolate framework. Dalton Trans. 2015;44(22):10136-10140. doi: 10.1039/c5dt01191d
- Wu Q, Wang D, Chen C, Peng C, Cai D, Wu Z. Fabrication of Fe3O4/ZIF-8 nanocomposite for simultaneous removal of copper and arsenic from water/soil/swine urine. J Environ Manage. 2021;290:112626. doi: 10.1016/j.jenvman.2021.112626
- Pang F, He M, Ge J. Controlled synthesis of Fe3O4/ ZIF-8 nanoparticles for magnetically separable nanocatalysts. Chemistry. 2015;21(18):6879-6887. doi: 10.1002/chem.201405921
- Lin J, Xin P, An L, et al. Fe3O4-ZIF-8 assemblies as pH and glutathione responsive T2-T1 switching magnetic resonance imaging contrast agent for sensitive tumor imaging in vivo. Chem Commun (Camb). 2019;55(4):478-481. doi: 10.1039/c8cc08943d
- Lan H, Gan N, Pan D, et al. Development of a novel magnetic molecularly imprinted polymer coating using porous zeolite imidazolate framework-8 coated magnetic iron oxide as carrier for automated solid phase microextraction of estrogens in fish and pork samples. J Chromatogr A. 2014;1365:35-44. doi: 10.1016/j.chroma.2014.08.096
- Lin Z, Bian W, Zheng J, Cai Z. Magnetic metal-organic framework nanocomposites for enrichment and direct detection of small molecules by negative-ion matrix-assisted laser desorption/ionization time-of-flight mass spectrometry. Chem Commun (Camb). 2015;51(42):8785-8788. doi: 10.1039/c5cc02495a
- Qi Y, Cao X, Abd El-Aty AM, et al. A magnetic zeolitic imidazolate framework nanohybrid for fast and efficient extraction of clothianidin, imidacloprid, acetamiprid, and thiacloprid in Water. J Nanosci Nanotechnol. 2019;19(6):3310-3318. doi: 10.1166/jnn.2019.16138
- Zhao S, Xie K, Guo Y, et al. Fabrication and biological activity of 3D-printed polycaprolactone/magnesium porous scaffolds for critical size bone defect repair. ACS Biomater Sci Eng. 2020;6(9):5120-5131. doi: 10.1021/acsbiomaterials.9b01911
- Yun HM, Ahn SJ, Park KR, et al. Magnetic nanocomposite scaffolds combined with static magnetic field in the stimulation of osteoblastic differentiation and bone formation. Biomaterials. 2016;85:88-98. doi: 10.1016/j.biomaterials.2016.01.035
- Faustini M, Kim J, Jeong GY, et al. Microfluidic approach toward continuous and ultrafast synthesis of metal-organic framework crystals and hetero structures in confined microdroplets. J Am Chem Soc. 2013;135(39):14619-14626. doi: 10.1021/ja4039642
- Mathur T, Singhal S, Khan S, Upadhyay DJ, Fatma T, Rattan A. Detection of biofilm formation among the clinical isolates of Staphylococci: an evaluation of three different screening methods. Indian J Med Microbiol. 2006;24(1):25-29. doi: 10.4103/0255-0857.19890
- Zhang S, Ye J, Liu X, et al. Titanium carbide/zeolite imidazole framework-8/polylactic acid electrospun membrane for near-infrared regulated photothermal/photodynamic therapy of drug-resistant bacterial infections. J Colloid Interface Sci. 2021;599:390-403. doi: 10.1016/j.jcis.2021.04.109
- He L, He T, Xing J, et al. Bone marrow mesenchymal stem cell-derived exosomes protect cartilage damage and relieve knee osteoarthritis pain in a rat model of osteoarthritis. Stem Cell Res Ther. 2020;11(1):276. doi: 10.1186/s13287-020-01781-w
- Karageorgiou V, Kaplan D. Porosity of 3D biomaterial scaffolds and osteogenesis. Biomaterials. 2005;26(27): 5474-5491. doi: 10.1016/j.biomaterials.2005.02.002
- Guo Y, Xie K, Jiang W, et al. In vitro and in vivo study of 3D-printed porous tantalum scaffolds for repairing bone defects. ACS Biomater Sci Eng. 2019;5(2): 1123-1133. doi: 10.1021/acsbiomaterials.8b01094
- Li G, Zhang L, Wang L, et al. Dual modulation of bone formation and resorption with zoledronic acid-loaded biodegradable magnesium alloy implants improves osteoporotic fracture healing: an in vitro and in vivo study. Acta Biomater. 2018;65:486-500. doi: 10.1016/j.actbio.2017.10.033
- Spicer PP, Kretlow JD, Young S, Jansen JA, Kasper FK, Mikos AG. Evaluation of bone regeneration using the rat critical size calvarial defect. Nat Protoc. 2012;7(10):1918-1929. doi: 10.1038/nprot.2012.113
- Niu H, Yang DL, Fu JW, Gao T, Wang J-X. Mechanical behavior and reinforcement mechanism of nanoparticle cluster fillers in dental resin composites: simulation and experimental study. Dent Mater. 2022;38(11):1801-1811. doi: 10.1016/j.dental.2022.09.015
- Kamonkhantikul K, Arksornnukit M, Takahashi H. Antifungal, optical, and mechanical properties of polymethylmethacrylate material incorporated with silanized zinc oxide nanoparticles. Int J Nanomedicine. 2017;12:2353-2360. doi: 10.2147/IJN.S132116
- Chen ZY, Gao S, Zhang YW, Zhou R-B, Zhou F. Antibacterial biomaterials in bone tissue engineering. J Mater Chem B. 2021;9(11):2594-2612. doi: 10.1039/d0tb02983a
- Dong Y, Liu W, Lei Y, et al. Effect of gelatin sponge with colloid silver on bone healing in infected cranial defects. Mater Sci Eng C Mater Biol Appl. 2017;70(Pt 1):371-377. doi: 10.1016/j.msec.2016.09.015
- Gandolfi MG, Zamparini F, Degli Esposti M, et al. Highly porous polycaprolactone scaffolds doped with calcium silicate and dicalcium phosphate dihydrate designed for bone regeneration. Mater Sci Eng C Mater Biol Appl. 2019;102:341-361. doi: 10.1016/j.msec.2019.04.040
- Preethi AM, Bellare JR. Concomitant effect of quercetin-and magnesium-doped calcium silicate on the osteogenic and antibacterial activity of scaffolds for bone regeneration. Antibiotics (Basel). 2021;10(10):1170. doi: 10.3390/antibiotics10101170
- Yang Y, Zan J, Shuai Y, et al. In situ growth of a metal-organic framework on graphene oxide for the chemo-photothermal therapy of bacterial infection in bone repair. ACS Appl Mater Interfaces. 2022;14(19):21996-22005. doi: 10.1021/acsami.2c04841
- Bo L, Yue Y, Haiyan W, et al. Zeolitic imidazolate framework-8 triggers the inhibition of arginine biosynthesis to combat methicillin-resistant Staphylococcus aureus. Small. 2023;19(14):e2205682. doi: 10.1002/smll.202205682
- Wenqin L, Wenying W, Xiaopei W, Zhaoab Y, Dai H. The antibacterial and antibiofilm activities of mesoporous hollow Fe3O4 nanoparticles in an alternating magnetic field. Biomater Sci. 2020;8(16):4492-4507. doi: 10.1039/d0bm00673d
- Wu D, Kang L, Tian J, et al. Exosomes derived from bone mesenchymal stem cells with the stimulation of Fe3O4 nanoparticles and static magnetic field enhance wound healing through upregulated miR-21-5p. Int J Nanomedicine. 2020;15:7979-7993. doi: 10.2147/IJN.S275650
- Henstock JR, Rotherham M, Rashidi H, Shakesheff KM, El Haj AJ. Remotely activated mechanotransduction via magnetic nanoparticles promotes mineralization synergistically with bone morphogenetic protein 2: applications for injectable cell therapy. Stem Cells Transl Med. 2014;3(11):1363-1374. doi: 10.5966/sctm.2014-0017
- Wang Q, Chen B, Cao M, et al. Response of MAPK pathway to iron oxide nanoparticles in vitro treatment promotes osteogenic differentiation of hBMSCs. Biomaterials. 2016;86:11-20. doi: 10.1016/j.biomaterials.2016.02.004
- Hu S, Zhou Y, Zhao Y, et al. Enhanced bone regeneration and visual monitoring via superparamagnetic iron oxide nanoparticle scaffold in rats. J Tissue Eng Regen Med. 2018;12(4):e2085-e2098. doi: 10.1002/term.2641
- Xia Y, Sun J, Zhao L, et al. Magnetic field and nano-scaffolds with stem cells to enhance bone regeneration. Biomaterials. 2018;183:151-170. doi: 10.1016/j.biomaterials.2018.08.040
- Meng J, Xiao B, Zhang Y, et al. Super-paramagnetic responsive nanofibrous scaffolds under static magnetic field enhance osteogenesis for bone repair in vivo. Sci Rep. 2013;3:2655. doi: 10.1038/srep02655
- Huang Z, He Y, Chang X, et al. A magnetic iron oxide/ polydopamine coating can improve osteogenesis of 3D-printed porous titanium scaffolds with a static magnetic field by upregulating the TGFβ-smads pathway. Adv Healthc Mater. 2020;9(14):e2000318. doi: 10.1002/adhm.202000318
- Yun HM, Ahn SJ, Park KR, et al. Magnetic nanocomposite scaffolds combined with static magnetic field in the stimulation of osteoblastic differentiation and bone formation. Biomaterials. 2016;85:88-98. doi: 10.1016/j.biomaterials.2016.01.035
- Zhuang J, Lin S, Dong L, Cheng K, Weng W. Magnetically actuated mechanical stimuli on Fe3O4/mineralized collagen coatings to enhance osteogenic differentiation of the MC3T3-E1 cells. Acta Biomater. 2018;71:49-60. doi: 10.1016/j.actbio.2018.03.009
- Marycz K, Alicka M, Kornicka-Garbowska K, et al. Promotion through external magnetic field of osteogenic differentiation potential in adipose-derived mesenchymal stem cells: design of polyurethane/poly(lactic) acid sponges doped with iron oxide nanoparticles. J Biomed Mater Res B Appl Biomater. 2020;108(4):1398-1411. doi: 10.1002/jbm.b.34488
- Cojocaru FD, Balan V, Verestiuc L. Advanced 3D magnetic scaffolds for tumor-related bone defects. Int J Mol Sci. 2022;23(24):16190. doi: 10.3390/ijms232416190
- Yang W, Zhong Y, He C, et al. Electrostatic self-assembly of pFe3O4 nanoparticles on graphene oxide: a co-dispersed nanosystem reinforces PLLA scaffolds. J Adv Res. 2020;24:191-203. doi: 10.1016/j.jare.2020.04.009
- Li M, Liu J, Cui X, et al. Osteogenesis effects of magnetic nanoparticles modified-porous scaffolds for the reconstruction of bone defect after bone tumor resection. Regen Biomater. 2019;6(6):373-381. doi: 10.1093/rb/rbz019
- Hong FT. Magnetic field effects on biomolecules, cells, and living organisms. Biosystems. 1995;36(3):187-229. doi: 10.1016/0303-2647(95)01555-y
- Zhao Y, Fan T, Chen J, et al. Magnetic bioinspired micro/nanostructured composite scaffold for bone regeneration. Colloids Surf B Biointerfaces. 2019;174:70-79. doi: 10.1016/j.colsurfb.2018.11.003
- Gao LN, An Y, Lei M, et al. The effect of the coumarin-like derivative osthole on the osteogenic properties of human periodontal ligament and jaw bone marrow mesenchymal stem cell sheets. Biomaterials. 2013;34(38):9937-9951. doi: 10.1016/j.biomaterials.2013.09.017
- Xia Y, Sun J, Zhao L, et al. Magnetic field and nano-scaffolds with stem cells to enhance bone regeneration. Biomaterials. 2018;183:151-170. doi: 10.1016/j.biomaterials.2018.08.040
- Hong G, He X, Shen Y, et al. Chrysosplenetin promotes osteoblastogenesis of bone marrow stromal cells via Wnt/β-catenin pathway and enhances osteogenesis in estrogen deficiency-induced bone loss. Stem Cell Res Ther. 2019;10(1):277. doi: 10.1186/s13287-019-1375-x
- MacDonald BT, Tamai K, He X. Wnt/beta-catenin signaling: components, mechanisms, and diseases. Dev Cell. 2009;17(1):9-26. doi: 10.1016/j.devcel.2009.06.016