Preparation and characterization of angled dual- and multi-branched nerve guidance conduits
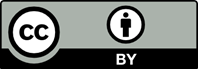
Branched nerve guidance conduit (NGC) provides a promising alternative to autografts for the effective treatment of severe peripheral nerve injuries. Despite this, the impact of branched architecture on nerve regeneration remains unclear, particularly concerning branch angle and number in multi-branched NGCs. In this study, we investigated the effects of branch angle and number on nerve regeneration by preparing and characterizing multi-angled and multi-branched NGCs. We designed and fabricated dual-branched NGCs (DBNs) with various branch angles and multi-branched NGCs (MBNs) through a digital light processing (DLP) printing process. When branched NGCs were implanted to bridge the linear sciatic nerve gap, nerve dual branches with acute (45°), right (90°), or obtuse (120°) branch angles were formed in DBNs, while nerve multi-branches were generated in MBNs. The regenerated nerves in DBNs with various angles exhibited comparable electrophysiological conduction and histological morphologies, indicating that the branch angle of dual-branched NGCs may not affect nerve branch regeneration. In contrast, the diameter of the regenerated nerve branches in MBNs decreased with increasing distance from the scaffold center, highlighting the potential significance of branch number in the design of branched NGCs. This study contributes valuable insights for designing, preparing, and applying branched NGCs, offering potential assistance in advancing nerve regeneration strategies.
- Rodríguez FJ, Valero-Cabré A, Navarro X. Regeneration and functional recovery following peripheral nerve injury. Drug Discov Today Dis Models. 2004;1(2):177-185. doi: 10.1016/j.ddmod.2004.09.008
- Scheib J, Hoke A. Advances in peripheral nerve regeneration. Nat Rev Neurol. 2013;9(12):668-676. doi: 10.1038/nrneurol.2013.227
- Sunderland S. The anatomy and physiology of nerve injury. Muscle Nerve. 1990;13(9):771-784. doi: 10.1002/mus.880130903
- Flores AJ, Lavernia CJ, Owens PW. Anatomy and physiology of peripheral nerve injury and repair. Am J Orthop. 2000;29(3):167-173.
- Muheremu A, Sun JG, Wang XY, Zhang F, Ao Q, Peng J. Combined use of Y-tube conduits with human umbilical cord stem cells for repairing nerve bifurcation defects. Neural Regen Res. 2016;11(4):664-669. doi: 10.4103/1673-5374.180755
- Allgood JE, Roballo KCS, Sparks BB, Bushman JS. The effects of graft source and orientation on outcomes after ablation of a branched peripheral nerve. Front Cell Neurosci. 2022;16:1055490. doi: 10.3389/fncel.2022.1055490
- Bengur FB, Stoy C, Binko MA, et al. Facial nerve repair: Bioengineering approaches in preclinical models. Tissue Eng Part B Rev. 2022;28(2):364-378. doi: 10.1089/ten.TEB.2020.0381
- Haller JR, Shelton C. Medial antebrachial cutaneous nerve: A new donor graft for repair of facial nerve defects at the skull base. Laryngoscope. 1997;107(8):1048-1052. doi: 10.1097/00005537-199708000-00008
- White WM, McKenna MJ, Deschler DG. Use of the thoracodorsal nerve for facial nerve grafting in the setting of pedicled latissimus dorsi reconstruction. Otolaryngol Head Neck Surg. 2006;135(6):962-964. doi: 10.1016/j.otohns.2005.09.017
- McKee D, Osemwengie B, Cox C. Distal digital nerve repair using nerve allograft with a dermal substitute: A case report. Hand (N Y). 2020;15(4):Np47-np50. doi: 10.1177/1558944719854169
- Leechavengvongs S, Witoonchart K, Uerpairojkit C. Penetrating injury to the terminal branches of the posterior interosseous nerve with nerve grafting. J Hand Surg. 2001;26(6):593-595. doi: 10.1054/jhsb.2001.0609
- Terzis JK, Kostas I. Vein grafts used as nerve conduits for obstetrical brachial plexus palsy reconstruction. Plast Reconstr Surg. 2007;120(7):1930-1941. doi: 10.1097/01.prs.0000287391.12943.00
- Stocco E, Barbon S, Emmi A, et al. Bridging gaps in peripheral nerves: From current strategies to future perspectives in conduit design. Int J Mol Sci. 2023;24(11). doi: 10.3390/ijms24119170
- Wu S, Shen W, Ge X, et al. Advances in large gap peripheral nerve injury repair and regeneration with bridging nerve guidance conduits. Macromol Biosci. 2023:e2300078. doi: 10.1002/mabi.202300078
- de Ruiter GC, Spinner RJ, Verhaagen J, Malessy MJ. Misdirection and guidance of regenerating axons after experimental nerve injury and repair. J Neurosurg. 2014;120(2):493-501. doi: 10.3171/2013.8.JNS122300
- Fang Y, Wang C, Liu Z, et al. 3D printed conductive multiscale nerve guidance conduit with hierarchical fibers for peripheral nerve regeneration. Adv Sci (Weinh). 2023;10(12):e2205744. doi: 10.1002/advs.202205744
- Semmler L, Naghilou A, Millesi F, et al. Silk-in-silk nerve guidance conduits enhance regeneration in a rat sciatic nerve injury model. Adv Healthc Mater. 2023;12(11):e2203237. doi: 10.1002/adhm.202203237
- Hasiba-Pappas S, Kamolz LP, Luze H, et al. Does electrical stimulation through nerve conduits improve peripheral nerve regeneration?-A systematic review. J Pers Med. 2023;13(3). doi: 10.3390/jpm13030414
- Jin B, Yu Y, Chen X, et al. Microtubes with gradient decellularized porcine sciatic nerve matrix from microfluidics for sciatic nerve regeneration. Bioact Mater. 2023;21:511-519. doi: 10.1016/j.bioactmat.2022.08.027
- Lin Y, Yu J, Zhang Y, et al. 4D printed tri-segment nerve conduit using zein gel as the ink for repair of rat sciatic nerve large defect. Biomater Adv. 2023;151:213473. doi: 10.1016/j.bioadv.2023.213473
- Zhang D, Yao Y, Duan Y, et al. Surface-anchored graphene oxide nanosheets on cell-scale micropatterned poly(d,l-lactide-co-caprolactone) conduits promote peripheral nerve regeneration. ACS Appl Mater Interfaces. 2020;12(7):7915- 7930. doi: 10.1021/acsami.9b20321
- Zhang DM, Suo HR, Qian J, Yin J, Fu JZ, Huang Y. Physical understanding of axonal growth patterns on grooved substrates: Groove ridge crossing versus longitudinal alignment. Biodes Manuf. 2020;3(4):348-360. doi: 10.1007/s42242-020-00089-1
- Allgood JE, Bittner GD, Bushman JS. Repair and regeneration of peripheral nerve injuries that ablate branch points. Neural Regen Res. 2023;18(12):2564-2568. doi: 10.4103/1673-5374.373679
- Zhang J, Tao J, Cheng H, et al. Nerve transfer with 3D-printed branch nerve conduits. Burns Trauma. 2022;10:tkac010. doi: 10.1093/burnst/tkac010
- Yao L, de Ruiter GC, Wang H, et al. Controlling dispersion of axonal regeneration using a multichannel collagen nerve conduit. Biomaterials. 2010;31(22):5789-5797. doi: 10.1016/j.biomaterials.2010.03.081
- Zhu W, Tringale KR, Woller SA, et al. Rapid continuous 3D printing of customizable peripheral nerve guidance conduits. Mater Today (Kidlington). 2018;21(9):951-959. doi: 10.1016/j.mattod.2018.04.001
- Isaacs J, Mallu S, Yan W, Little B. Consequences of oversizing: Nerve-to-nerve tube diameter mismatch. J Bone Joint Surg Am. 2014;96(17):1461-1467. doi: 10.2106/JBJS.M.01420
- Ma Y, Gao H, Wang H, Cao X. Engineering topography: Effects on nerve cell behaviors and applications in peripheral nerve repair. J Mater Chem B. 2021;9(32):6310-6325. doi: 10.1039/d1tb00782c
- Moore AM, Kasukurthi R, Magill CK, Farhadi HF, Borschel GH, Mackinnon SE. Limitations of conduits in peripheral nerve repairs. Hand (N Y). 2009;4(2):180-186. doi: 10.1007/s11552-008-9158-3
- Giusti G, Shin RH, Lee JY, Mattar TG, Bishop AT, Shin AY. The influence of nerve conduits diameter in motor nerve recovery after segmental nerve repair. Microsurgery. 2014;34(8):646-652. doi: 10.1002/micr.22312
- Hoffman-Kim D, Mitchel JA, Bellamkonda RV. Topography, cell response, and nerve regeneration. Annu Rev Biomed Eng. 2010;12:203-231. doi: 10.1146/annurev-bioeng-070909-105351
- Petcu EB, Midha R, McColl E, Popa-Wagner A, Chirila TV, Dalton PD. 3D printing strategies for peripheral nerve regeneration. Biofabrication. 2018;10(3):032001. doi: 10.1088/1758-5090/aaaf50
- Sarker M, Naghieh S, McInnes AD, Schreyer DJ, Chen XB. Strategic design and fabrication of nerve guidance conduits for peripheral nerve regeneration. Biotechnol J. 2018;13(7):1700635. doi: 10.1002/biot.201700635
- Vijayavenkataraman S, Zhang S, Thaharah S, Sriram G, Lu WF, Fuh JYH. Electrohydrodynamic jet 3D printed nerve guide conduits (NGCs) for peripheral nerve injury repair. Polymers. 2018;10(7):753. doi: 10.3390/polym10070753
- Kim JH, Kim I, Seol YJ, et al. Neural cell integration into 3D bioprinted skeletal muscle constructs accelerates restoration of muscle function. Nat Commun. 2020;11(1):1025. doi: 10.1038/s41467-020-14930-9
- Zhang J, Hu Q, Wang S, Tao J, Gou M. Digital light processing based three-dimensional printing for medical applications. Int J Bioprint. 2020;6(1):242. doi: 10.18063/ijb.v6i1.242
- Akbari M, Khademhosseini A. Tissue bioprinting for biology and medicine. Cell. 2022;185(15):2644-2648. doi: 10.1016/j.cell.2022.06.015
- Johnson BN, Lancaster KZ, Zhen G, et al. 3D printed anatomical nerve regeneration pathways. Adv Funct Mater. 2015;25(39):6205-6217. doi: 10.1002/adfm.201501760
- Johnson BN, Jia X. 3D printed nerve guidance channels: computer-aided control of geometry, physical cues, biological supplements and gradients. Neural Regen Res. 2016;11(10):1568-1569. doi: 10.4103/1673-5374.193230
- Jain P, Kathuria H, Dubey N. Advances in 3D bioprinting of tissues/organs for regenerative medicine and in-vitro models. Biomaterials. 2022;287:121639. doi: 10.1016/j.biomaterials.2022.121639
- Suri S, Han LH, Zhang W, Singh A, Chen S, Schmidt CE. Solid freeform fabrication of designer scaffolds of hyaluronic acid for nerve tissue engineering. Biomed Microdevices. 2011;13(6):983-993. doi: 10.1007/s10544-011-9568-9
- Fairbanks BD, Schwartz MP, Bowman CN, Anseth KS. Photoinitiated polymerization of PEG-diacrylate with lithium phenyl-2,4,6-trimethylbenzoylphosphinate: Polymerization rate and cytocompatibility. Biomaterials. 2009;30(35):6702-6707. doi: 10.1016/j.biomaterials.2009.08.055
- Shirahama H, Lee BH, Tan LP, Cho NJ. Precise tuning of facile one-pot gelatin methacryloyl (GelMA) synthesis. Sci Rep. 2016;6:31036. doi: 10.1038/srep31036
- Liu X, Tao J, Liu J, et al. 3D printing enabled customization of functional microgels. ACS Appl Mater Interfaces. 2019;11(13):12209-12215. doi: 10.1021/acsami.8b18701
- Tao J, Zhang J, Du T, et al. Rapid 3D printing of functional nanoparticle-enhanced conduits for effective nerve repair. Acta Biomater. 2019;90:49-59. doi: 10.1016/j.actbio.2019.03.047
- Xu X, Tao J, Wang S, et al. 3D printing of nerve conduits with nanoparticle-encapsulated RGFP966. Appl Mater Today. 2019;16:247-256. doi: 10.1016/j.apmt.2019.05.014
- Gu Y, Ji Y, Zhao Y, et al. The influence of substrate stiffness on the behavior and functions of Schwann cells in culture. Biomaterials. 2012;33(28): 6672-6681. doi: 10.1016/j.biomaterials.2012.06.006
- Dalamagkas K, Tsintou M, Seifalian A. Advances in peripheral nervous system regenerative therapeutic strategies: A biomaterials approach. Mater Sci Eng C Mater Biol Appl. 2016;65:425-432. doi: 10.1016/j.msec.2016.04.048
- Zhu W, Ma X, Gou M, Mei D, Zhang K, Chen S. 3D printing of functional biomaterials for tissue engineering. Curr Opin Biotechnol. 2016;40:103-112. doi: 10.1016/j.copbio.2016.03.014
- Wu W, Dong Y, Liu H, et al. 3D printed elastic hydrogel conduits with 7,8-dihydroxyflavone release for peripheral nerve repair. Mater Today Bio. 2023;20:100652. doi: 10.1016/j.mtbio.2023.100652
- Tao J, Liu H, Wu W, et al. 3D‐printed nerve conduits with live platelets for effective peripheral nerve repair. Adv Funct Mater. 2020;30(42):2004272. doi: 10.1002/adfm.202004272
- Politis MJ. Specificity in mammalian peripheral nerve regeneration at the level of the nerve trunk. Brain Res. 1985;328(2):271-276. doi: 10.1016/0006-8993(85)91038-8
- Brushart TM, Seiler WAt. Selective reinnervation of distal motor stumps by peripheral motor axons. Exp Neurol. 1987;97(2):289-300. doi: 10.1016/0014-4886(87)90090-2
- Robinson GA, Madison RD. Motor neurons can preferentially reinnervate cutaneous pathways. Exp Neurol. 2004;190(2):407-413. doi: 10.1016/j.expneurol.2004.08.007
- Brushart TM, Gerber J, Kessens P, Chen YG, Royall RM. Contributions of pathway and neuron to preferential motor reinnervation. J Neurosci. 1998;18(21):8674-8681. doi: 10.1523/jneurosci.18-21-08674.1998
- Lacomis D. Electrophysiology of neuromuscular disorders in critical illness. Muscle Nerve. 2013;47(3):452-463. doi: 10.1002/mus.23615
- Wolthers M, Moldovan M, Binderup T, Schmalbruch H, Krarup C. Comparative electrophysiological, functional, and histological studies of nerve lesions in rats. Microsurgery. 2005;25(6):508-519. doi: 10.1002/micr.20156
- Vijayavenkataraman S. Nerve guide conduits for peripheral nerve injury repair: A review on design, materials and fabrication methods. Acta Biomater. 2020;106:54-69. doi: 10.1016/j.actbio.2020.02.003
- Conforti L, Gilley J, Coleman MP. Wallerian degeneration: An emerging axon death pathway linking injury and disease. Nat Rev Neurosci. 2014;15(6):394-409. doi: 10.1038/nrn3680
- Evangelista MS, Perez M, Salibian AA, et al. Single-lumen and multi-lumen poly(ethylene glycol) nerve conduits fabricated by stereolithography for peripheral nerve regeneration in vivo. J Reconstr Microsurg. 2015;31(5):327-335. doi: 10.1055/s-0034-1395415
- Zhai QK, Wang XK, Tan XX, Li L. Reconstruction for facial nerve defects of zygomatic or marginal mandibular branches using upper buccal or cervical branches. J Craniofac Surg. 2015;26(1):245-247. doi: 10.1097/scs.0000000000001196
- Chowdhry S, Yoder EM, Cooperman RD, Yoder VR, Wilhelmi BJ. Locating the cervical motor branch of the facial nerve: Anatomy and clinical application. Plast Reconstr Surg. 2010;126(3):875-879. doi: 10.1097/PRS.0b013e3181e3b374
- Tao J, Hu Y, Wang S, et al. A 3D-engineered porous conduit for peripheral nerve repair. Sci Rep. 2017;7:46038. doi: 10.1038/srep46038
- Yao Z, Yan L-W, Qiu S, et al. Customized scaffold design based on natural peripheral nerve fascicle characteristics for biofabrication in tissue regeneration. Biomed Res Int. 2019;20193845780. doi: 10.1155/2019/3845780
- Park JH, Jang J, Lee JS, Cho DW. Three-dimensional printing of tissue/organ analogues containing living cells. Ann Biomed Eng. 2017;45(1):180-194. doi: 10.1007/s10439-016-1611-9
- You S, Xiang Y, Hwang HH, et al. High cell density and high-resolution 3D bioprinting for fabricating vascularized tissues. Sci Adv. 2023;9(8):eade7923. doi: 10.1126/sciadv.ade7923
- Huang Y, Wu W, Liu H, et al. 3D printing of functional nerve guide conduits. Burns Trauma. 2021;9:tkab011. doi: 10.1093/burnst/tkab011
- Maki Y, Yoshizu T, Tsubokawa N. Selective regeneration of motor and sensory axons in an experimental peripheral nerve model without endorgans. Scand J Plast Reconstr Surg Hand Surg. 2005;39(5):257-260. doi: 10.1080/0284431051006510
- Bolleboom A, de Ruiter GCW, Coert JH, Tuk B, Holstege JC, van Neck JW. Novel experimental surgical strategy to prevent traumatic neuroma formation by combining a 3D-printed Y-tube with an autograft. J Neurosurg. 2019;130(1):184-196. doi: 10.3171/2017.8.Jns17276
- Shahriari D, Loke G, Tafel I, et al. Scalable fabrication of porous microchannel nerve guidance scaffolds with complex geometries. Adv Mater. 2019;31(30):e1902021. doi: 10.1002/adma.201902021
- Chiu DT, Smahel J, Chen L, Meyer V. Neurotropism revisited. Neurol Res. 2004;26(4):381-387. doi: 10.1179/016164104225013815
- Abernethy DA, Thomas PK, Rud A, King RH. Mutual attraction between emigrant cells from transected denervated nerve. J Anat. 1994;184(Pt 2):239-249.
- Hu M, Xiao H, Niu Y, Liu H, Zhang L. Long-term follow-up of the repair of the multiple-branch facial nerve defect using acellular nerve allograft. J Oral Maxillofac Surg. 2016;74(1):218.e1-11. doi: 10.1016/j.joms.2015.08.005
- Cheesborough JE, Smith LH, Kuiken TA, Dumanian GA. Targeted muscle reinnervation and advanced prosthetic arms. Semin Plast Surg. 2015;29(1):62-72. doi: 10.1055/s-0035-1544166
- Szwedowski D, Ambrozy J, Grabowski R, Dallo I, Mobasheri A. Diagnosis and treatment of the most common neuropathies following knee injuries and reconstructive surgery - A narrative review. Heliyon. 2021;7(9):e08032. doi: 10.1016/j.heliyon.2021.e08032
- Liu Q, Li S, Zhang Y, et al. Anatomic basis and clinical effect of selective dorsal neurectomy for patients with lifelong premature ejaculation: A randomized controlled trial. J Sex Med. 2019;16(4):522-530. doi: 10.1016/j.jsxm.2019.01.319
- Moon du G. Is there a place for surgical treatment of premature ejaculation? Transl Androl Urol. 2016;5(4):502-507. doi: 10.21037/tau.2016.05.06