Bioprinting of cell-laden protein-based hydrogels: From cartilage to bone tissue engineering
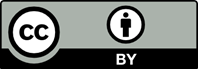
The fabrication of cell-laden protein-based hydrogels (PBHs) for bioprinting necessitates careful consideration of numerous factors to ensure optimal structure and functionality. Bioprinting techniques, such as single-cell, multi-cell, and cell aggregate bioprinting, are employed to encapsulate cells within PBHs bioink, enabling the creation of scaffolds for cartilage and bone regeneration. During the fabrication process, it is imperative to account for biophysical and biochemical factors that influence cell behavior and protein structure within the PBHs. Precise control of crosslinking methods, hydrogel rheological properties, and printing parameters is also crucial to achieve desired scaffold properties without compromising cell viability and protein integrity. This review primarily focuses on the influence of biophysical factors, including composition, microstructure, biodegradation, and crosslinking, as well as biochemical factors, including chemical structure, growth factors, and signaling molecules, on protein structure and cell behavior. Additionally, key considerations for bioprinting PBHs and their impact on the successful regeneration of tissues are discussed. Furthermore, the review highlights current advancements, existing challenges, and promising prospects in the development of cell-laden PBHs for bioprinting applications and the regeneration of bone and cartilage.
- Ozbolat IT, 2017, Bioprinting of osteochondral tissues: A perspective on current gaps and future trends. Int J Bioprint, 3(2). http://doi:10.18063/IJB.2017.02.007
- Deng C, Chang J, Wu C, 2019, Bioactive scaffolds for osteochondral regeneration. J Orthop Transl, 17: 15–25. http://doi:10.1016/j.jot.2018.11.006
- Gonçalves AM, Moreira A, Weber A, et al., 2021, Costa, osteochondral tissue engineering: The potential of electrospinning and additive manufacturing. Pharmaceutics, 13(7): 983. http://doi:10.3390/pharmaceutics13070983
- Moris H, Ghaee A, Karimi M, et al., 2022, Preparation and characterization of Pullulan-based nanocomposite scaffold incorporating Ag-Silica Janus particles for bone tissue engineering. Biomater Adv, 135: 212733. http://doi:10.1016/j.bioadv.2022.212733
- Ghorbani F, Ghalandari B, Khan AL, et al., 2020, Decoration of electrical conductive polyurethane-polyaniline/polyvinyl alcohol matrixes with mussel-inspired polydopamine for bone tissue engineering. Biotechnol Prog, 36(6): e3043. http://doi:10.1002/btpr.3043
- Ghorbani F, Zamanian A, Sahranavard M, 2019, Mussel-inspired polydopamine-mediated surface modification of freeze-cast poly (ε-caprolactone) scaffolds for bone tissue engineering applications. Biomed Eng/Biomed Tech, 65(3): 273–287. http://doi:10.1515/bmt-2019-0061
- Sahranavard M, Zamanian A, Ghorbani F, et al., 2020, A critical review on three dimensional-printed chitosan hydrogels for development of tissue engineering. Bioprinting, 17: e00063. http://doi:10.1016/j.bprint.2019.e00063
- Yilmaz B, Al Rashid A, Mou YA, et al., 2021, Bioprinting: A review of processes, materials and applications. Bioprinting, 23: e00148. https://doi.org/10.1016/j.bprint.2021.e00148
- Ding S, Feng L, Wu J, et al., 2018, Bioprinting of stem cells : Interplay of bioprinting process, bioinks, and stem cell properties. ACS Biomater Sci Eng, 4(9): 3108–3124. http://doi:10.1021/acsbiomaterials.8b00399
- Kundu J, Shim J-H, Jang J, et al., 2015, An additive manufacturing-based PCL-alginate-chondrocyte bioprinted scaffold for cartilage tissue engineering. J Tissue Eng Regen Med, 9(11): 1286–1297. http://doi:10.1002/term.1682
- Ozbolat IT, 2015, Bioprinting scale-up tissue and organ constructs for transplantation. Trends Biotechnol, 33(7): 395–400. http://doi:10.1016/j.tibtech.2015.04.005
- Kačarević Ž, Rider P, Alkildani S, et al., 2018, An introduction to 3D bioprinting: Possibilities, challenges and future aspects. Materials (Basel), 11(11): 2199. http://doi:10.3390/ma11112199
- Ghorbani F, Ghalandari B, Khajehmohammadi M, et al., 2023, Photo-cross-linkable hyaluronic acid bioinks for bone and cartilage tissue engineering applications. Int Mater Rev, 68(6): 1–42. http://doi:10.1080/09506608.2023.2167559
- Raza F, Zafar H, Zhu Y, et al., 2018, A review on recent advances in stabilizing peptides/proteins upon fabrication in hydrogels from biodegradable polymers. Pharmaceutics, 10(1): 16. http://doi:10.3390/pharmaceutics10010016
- Di Bella C, Fosang A, Donati DM, et al., 2015, 3D bioprinting of cartilage for orthopedic surgeons : Reading between the lines. Front Surg, 2: 1–7. http://doi:10.3389/fsurg.2015.00039
- Tolabi H, Davari N, Khajehmohammadi M, et al., 2023, Progress of microfluidic hydrogel‐based scaffolds and organ‐on‐chips for the cartilage tissue engineering. Adv Mater, 35(26): 2208852. http://doi:10.1002/adma.202208852
- Razaviye MK, Tafti RA, Khajehmohammadi M, 2022, An investigation on mechanical properties of PA12 parts produced by a SLS 3D printer: An experimental approach. CIRP J Manuf Sci Technol, 38: 760–768. http://doi:10.1016/j.cirpj.2022.06.016
- Dababneh AB, Ozbolat IT, 2014, Bioprinting technology: A current state-of-the-art review. J Manuf Sci Eng Trans ASME, 136(6): 1–12. http://doi:10.1115/1.4028512
- Khajehmohammadi M, Azizi Tafti R, Nikukar H, 2023, Effect of porosity on mechanical and biological properties of bioprinted scaffolds. J Biomed Mater Res Part A, 111(2): 245–260. http://doi:10.1002/jbm.a.37455
- Schloss AC, Williams DM, Regan LJ, 2016, Protein-based hydrogels for tissue engineering. Adv Exp Med Biol, 940: 167–177. http://doi:10.1007/978-3-319-39196-0_8
- Davari N, Bakhtiary N, Khajehmohammadi M, et al., 2022, Protein-based hydrogels: Promising materials for tissue engineering. Polymers (Basel), 14: 986. http://doi:10.3390/polym14050986
- Rasheed T, Bilal M, Zhao Y, et al., 2019, Physiochemical characteristics and bone/cartilage tissue engineering potentialities of protein-based macromolecules — A review. Int J Biol Macromol, 121(5): 13–22. http://doi:10.1016/j.ijbiomac.2018.10.009
- Catoira MC, Fusaro L, Di Francesco D, et al., 2019, Overview of natural hydrogels for regenerative medicine applications. J Mater Sci Mater Med, 30(10): 115. http://doi:10.1007/s10856-019-6318-7
- Maia FRA, Oliveira JM, Reis RL, eds., 2023, Handbook of the Extracellular Matrix, Springer International Publishing, Cham. http://doi:10.1007/978-3-030-92090-6
- Włodarczyk-Biegun MK, del Campo A, 2017, 3D bioprinting of structural proteins. Biomaterials, 134: 180–201. http://doi:10.1016/j.biomaterials.2017.04.019
- Lotfi Z, Khakbiz M, Davari N, et al., 2023, Fabrication and multiscale modeling of polycaprolactone/amniotic membrane electrospun nanofiber scaffolds for wound healing. Artif Organs, 00: 1–18. http://doi:10.1111/aor.14518
- Norouzi F, Pourmadadi M, Yazdian F, et al., 2022, PVA-based nanofibers containing chitosan modified with graphene oxide and carbon quantum dot-doped TiO2 enhance wound healing in a rat model. J Funct Biomater, 13(4): 300. http://doi:10.3390/jfb13040300
- Yahay Z, Tolabi H, Delavar F, et al., 2023, Fabrication of meso/macroporous TiO2/PCL composite scaffolds by direct ink writing: The effects of porogen content on the compressive modulus and in vitro behavior. Mater Today Commun, 35: 105769. http://doi:10.1016/j.mtcomm.2023.105769
- Veiga A, Silva IV, Duarte MM, et al., 2021, Current trends on protein driven bioinks for 3D printing. Pharmaceutics, 13(9): 1444. http://doi:10.3390/pharmaceutics13091444
- Khoeini R, Nosrati H, Akbarzadeh A, et al., 2021, Natural and synthetic bioinks for 3D bioprinting. Adv NanoBiomed Res, 1(8): 2000097. http://doi:10.1002/anbr.202000097
- Abascal NC, Regan L, 2018, The past, present and future of protein-based materials. Open Biol, 8(10): 180113. http://doi:10.1098/rsob.180113
- Jeshvaghani PA, Pourmadadi M, Yazdian F, et al., 2023, Synthesis and characterization of a novel, pH-responsive sustained release nanocarrier using polyethylene glycol, graphene oxide, and natural silk fibroin protein by a green nano emulsification method to enhance cancer treatment. Int J Biol Macromol, 226: 1100–1115. http://doi:10.1016/j.ijbiomac.2022.11.226
- Hajiabbas M, Okoro OV, Delporte C, et al., 2023, Proteins and polypeptides as biomaterials inks for 3D printing, in Handbook of the Extracellular Matrix, Springer International Publishing, Cham, 2023, 1–34. http://doi:10.1007/978-3-030-92090-6_15-1
- Gagner JE, Kim W, Chaikof EL, 2014, Designing protein-based biomaterials for medical applications. Acta Biomater, 10(4): 1542–1557. http://doi:10.1016/j.actbio.2013.10.001
- Lee A, Hudson AR, Shiwarski DJ, et al., 2019, 3D bioprinting of collagen to rebuild components of the human heart. Science, 365(6452): 482–487. http://doi:10.1126/science.aav9051
- Łabowska MB, Cierluk K, Jankowska AM, et al., 2021, A review on the adaption of alginate-gelatin hydrogels for 3D cultures and bioprinting. Materials (Basel), 14(4): 858. http://doi:10.3390/ma14040858
- Liu S, Yu J-M, Gan Y-C, et al., 2023, Biomimetic natural biomaterials for tissue engineering and regenerative medicine: New biosynthesis methods, recent advances, and emerging applications. Mil Med Res, 10(1): 16. http://doi:10.1186/s40779-023-00448-w
- Cheung H-Y, Lau K-T, Lu T-P, et al., 2007, A critical review on polymer-based bio-engineered materials for scaffold development. Compos Part B Eng, 38(3): 291–300. http://doi:10.1016/j.compositesb.2006.06.014
- Al Enezy‐Ulbrich MA, Malyaran H, Lange RD, et al., 2020, Impact of reactive amphiphilic copolymers on mechanical properties and cell responses of fibrin‐based hydrogels. Adv Funct Mater, 30(38): 2003528. http://doi:10.1002/adfm.202003528
- Vernerey FJ, Greenwald EC, Bryant SJ, 2012, Triphasic mixture model of cell-mediated enzymatic degradation of hydrogels. Comput Methods Biomech Biomed Engin, 15(11): 1197–1210. http://doi:10.1080/10255842.2011.585973
- Khan F, Atif M, Haseen M, et al., 2022, Synthesis, classification and properties of hydrogels: Their applications in drug delivery and agriculture. J Mater Chem B, 10(2): 170–203. http://doi:10.1039/D1TB01345A
- Chakraborty J, Ghosh S, 2020, Cellular proliferation, self-assembly, and modulation of signaling pathways in silk fibroin gelatin-based 3D bioprinted constructs. ACS Appl Bio Mater, 3(12): 8309–8320. http://doi:10.1021/acsabm.0c01252
- Bakhtiary N, Ghalandari B, Ghorbani F, et al., 2023, Advances in peptide-based hydrogel for tissue engineering. Polymers (Basel), 15(5): 1068. http://doi:10.3390/polym15051068
- Huettner N, Dargaville TR, Forget A, 2018, Discovering cell-adhesion peptides in tissue engineering: Beyond RGD. Trends Biotechnol, 36(4): 372–383. http://doi:10.1016/j.tibtech.2018.01.008
- Gao G, Cui X, Bone BÁ, et al., 2016, Three-dimensional bioprinting in tissue engineering and regenerative medicine. Biotechnol Lett, 38: 422–434. http://doi:10.1007/s10529-015-1975-1
- Tasoglu S, Demirci U, 2013, Bioprinting for stem cell research. Trends Biotechnol, 31(1): 10–19. http://doi:10.1016/j.tibtech.2012.10.005
- Riba J, Renz N, Niemöller C, et al., 2016, Molecular genetic characterization of individual cancer cells isolated via single-cell printing. PLoS One, 11(9): e0163455. http://doi:10.1371/journal.pone.0163455
- Stumpf F, Schoendube J, Gross A, et al., 2015, Single-cell PCR of genomic DNA enabled by automated single-cell printing for cell isolation. Biosens Bioelectron, 69: 301–306. http://doi:10.1016/j.bios.2015.03.008
- Zhang X, Wei X, Wei Y, et al., 2020, The up-to-date strategies for the isolation and manipulation of single cells. Talanta, 218: 121147. http://doi:10.1016/j.talanta.2020.121147
- Herzenberg LA, Parks D, Sahaf B, et al., 2002, The history and future of the fluorescence activated cell sorter and flow cytometry: A view from stanford. Clin Chem, 48(10): 1819–1827. http://doi:10.1093/clinchem/48.10.1819
- Zhou Y, Shaw D, Lam C, et al., 2018, Beating the odds: The poisson distribution of all input cells during limiting dilution grossly underestimates whether a cell line is clonally-derived or not. Biotechnol Prog, 34(3): 559–569. http://doi:10.1002/btpr.2560
- Gross A, Schoendube J, Zimmermann S, et al., 2015, Technologies for single-cell isolation. Int J Mol Sci, 16(8): 16897–16919. http://doi:10.3390/ijms160816897
- Zhang X, Li T, Liu F, et al., 2019, Comparative analysis of droplet-based ultra-high-throughput single-cell RNA-Seq systems. Mol Cell, 73(1): 130–142.e5. http://doi:10.1016/j.molcel.2018.10.020
- Matuła K, Rivello F, Huck WTS, 2020, Single‐cell analysis using droplet microfluidics. Adv Biosyst, 4(1): 1900188. http://doi:10.1002/adbi.201900188
- Klein AM, Mazutis L, Akartuna I, et al., 2015, Droplet barcoding for single-cell transcriptomics applied to embryonic stem cells. Cell, 161(5): 1187–1201. http://doi:10.1016/j.cell.2015.04.044
- Han X, Wang R, Zhou Y, et al., 2018, Mapping the mouse cell atlas by microwell-seq. Cell, 172(5): 1091–1107.e17. http://doi:10.1016/j.cell.2018.02.001
- Rettig JR, Folch A, 2005, Large-scale single-cell trapping and imaging using microwell arrays. Anal Chem, 77(17): 5628–5634. http://doi:10.1021/ac0505977
- Zhou Y, Basu S, Wohlfahrt KJ, et al., 2016, A microfluidic platform for trapping, releasing and super-resolution imaging of single cells. Sensors Actuators B Chem, 232: 680–691. http://doi:10.1016/j.snb.2016.03.131
- Tan W-H, Takeuchi S, 2007, A trap-and-release integrated microfluidic system for dynamic microarray applications. Proc Natl Acad Sci, 104(4): 1146–1151. http://doi:10.1073/pnas.0606625104
- Calvert P, 2007, Printing cells. Science, 318(5848): 208–209. http://doi:10.1126/science.1144212
- Yusof A, Keegan H, Spillane CD, et al., 2011, Inkjet-like printing of single-cells. Lab Chip, 11(14): 2447. http://doi:10.1039/c1lc20176j
- Wang Y, Wang X, Pan T, et al., 2021, Label-free single-cell isolation enabled by microfluidic impact printing and real-time cellular recognition. Lab Chip, 21(19): 3695–3706. http://doi:10.1039/D1LC00326G
- Schoendube J, Wright D, Zengerle R, et al., 2015, Single-cell printing based on impedance detection. Biomicrofluidics, 9(1): 014117. http://doi:10.1063/1.4907896
- Nagai M, Kato K, Soga S, et al., 2020, Scalable parallel manipulation of single cells using micronozzle array integrated with bidirectional electrokinetic pumps. Micromachines, 11(4): 442. http://doi:10.3390/mi11040442
- Feng L, Sun Y, Ohsumi C, et al., 2013, Accurate dispensing system for single oocytes using air ejection. Biomicrofluidics, 7(5): 054113. http://doi:10.1063/1.4824394
- Demirci U, Montesano G, 2007, Single cell epitaxy by acoustic picolitre droplets. Lab Chip, 7(9): 1139. http://doi:10.1039/b704965j
- Guo F, Mao Z, Chen Y, et al., 2016, Three-dimensional manipulation of single cells using surface acoustic waves. Proc Natl Acad Sci, 113(6): 1522–1527. http://doi:10.1073/pnas.1524813113
- Bertassoni LE, 2022, Bioprinting of complex multicellular organs with advanced functionality—recent progress and challenges ahead. Adv Mater, 34(3): 2101321. http://doi:10.1002/adma.202101321
- Daly AC, Prendergast ME, Hughes AJ, et al., 2021, Bioprinting for the biologist. Cell, 184(1): 18–32. http://doi:10.1016/j.cell.2020.12.002
- Barron JA, Krizman DB, Ringeisen BR, 2005, Laser printing of single cells: Statistical analysis, cell viability, and stress. Ann Biomed Eng, 33(2): 121–130. http://doi:10.1007/s10439-005-8971-x
- Yamaguchi S, Ueno A, Akiyama Y, et al., 2012, Cell patterning through inkjet printing of one cell per droplet. Biofabrication, 4(4): 045005. http://doi:10.1088/1758-5082/4/4/045005
- Zhang K, Chou C-K, Xia X, et al., 2014, Block-cell-printing for live single-cell printing. Proc Natl Acad Sci, 111(8): 2948–2953. http://doi:10.1073/pnas.1313661111
- Zhou X, Wu H, Wen H, et al., 2022, Advances in single-cell printing. Micromachines, 13(1): 80. http://doi:10.3390/mi13010080
- Murphy SV, De Coppi P, Atala A, 2020, Opportunities and challenges of translational 3D bioprinting. Nat Biomed Eng, 4(4): 370–380. http://doi:10.1038/s41551-019-0471-7
- Zhang P, Abate AR, 2020, High‐definition single‐cell printing: Cell‐by‐cell fabrication of biological structures. Adv Mater, 32(52): 2005346. http://doi:10.1002/adma.202005346
- Hong S, Lee JY, Hwang C, et al., 2016, Inhibition of Rho-associated protein kinase increases the angiogenic potential of mesenchymal stem cell aggregates via paracrine effects. Tissue Eng Part A, 22(3-4): 233–243. http://doi:10.1089/ten.tea.2015.0289
- Lei J, Trevino E, Temenoff J, 2016, Cell number and chondrogenesis in human mesenchymal stem cell aggregates is affected by the sulfation level of heparin used as a cell coating. J Biomed Mater Res Part A, 104(7): 1817–1829. http://doi:10.1002/jbm.a.35713
- Lin R-Z, Chang H-Y, 2008, Recent advances in three-dimensional multicellular spheroid culture for biomedical research. Biotechnol J, 3(9-10): 1172–1184. http://doi:10.1002/biot.200700228
- Derby B, 2012, Printing and prototyping of tissues and scaffolds. Science, 338(6109): 921–926. http://doi:10.1126/science.1226340
- Marga F, Jakab K, Khatiwala C, et al., 2012, Toward engineering functional organ modules by additive manufacturing. Biofabrication, 4(2): 022001. http://doi:10.1088/1758-5082/4/2/022001
- Marchioli G, van Gurp L, van Krieken PP, et al., 2015, Fabrication of three-dimensional bioplotted hydrogel scaffolds for islets of Langerhans transplantation. Biofabrication, 7(2): 025009. http://doi:10.1088/1758-5090/7/2/025009
- Tan Y, Richards DJ, Trusk TC, et al., 2014, 3D printing facilitated scaffold-free tissue unit fabrication. Biofabrication, 6(2): 024111. http://doi:10.1088/1758-5082/6/2/024111
- Yang X, Sun Y, Wang Q, 2013, A phase field approach for multicellular aggregate fusion in biofabrication. J Biomech Eng, 135: 071005. http://doi:10.1115/1.4024139
- Yang X, Mironov V, Wang Q, 2012, Modeling fusion of cellular aggregates in biofabrication using phase field theories. J Theor Biol, 303: 110–118. http://doi:10.1016/j.jtbi.2012.03.003
- Mironov V, Visconti RP, Kasyanov V, et al., 2009, Organ printing: Tissue spheroids as building blocks. Biomaterials, 30(12): 2164–2174. http://doi:10.1016/j.biomaterials.2008.12.084
- Keriquel V, Oliveira H, Rémy M, et al., 2017, In situ printing of mesenchymal stromal cells, by laser-assisted bioprinting, for in vivo bone regeneration applications. Sci Rep, 7(1): 1778. http://doi:10.1038/s41598-017-01914-x
- Adhikari J, Perwez MS, Das A, et al., 2021, Development of hydroxyapatite reinforced alginate–chitosan based printable biomaterial-ink. Nano-Structures Nano-Objects, 25: 100630. http://doi:10.1016/j.nanoso.2020.100630
- Ozbolat IT, Hospodiuk M, 2016, Current advances and future perspectives in extrusion-based bioprinting. Biomaterials, 76: 321–343. http://doi:10.1016/j.biomaterials.2015.10.076
- Kim SH, Yeon YK, Lee JM, et al., 2018, Precisely printable and biocompatible silk fibroin bioink for digital light processing 3D printing. Nat Commun, 9(1): 1–14. http://doi:10.1038/s41467-018-03759-y
- Mandrycky C, Wang Z, Kim K, et al., 2016, 3D bioprinting for engineering complex tissues. Biotechnol Adv, (4). http://doi:10.1016/j.biotechadv.2015.12.011
- Ren X, Wang F, Chen C, et al., 2016, Engineering zonal cartilage through bioprinting collagen type II hydrogel constructs with biomimetic chondrocyte density gradient. BMC Musculoskelet Disord, 17(1): 1–10. http://doi:10.1186/s12891-016-1130-8
- Kesti M, Eberhardt C, Pagliccia G, et al., 2015, Bioprinting complex cartilaginous structures with clinically compliant biomaterials. Adv Funct Mater, 25(48): 7406–7417. http://doi:10.1002/adfm.201503423
- López-Marcial GR, Zeng AY, Osuna C, et al., 2018, Agarose-based hydrogels as suitable bioprinting materials for tissue engineering. ACS Biomater Sci Eng, 4(10): 3610–3616. http://doi:10.1021/acsbiomaterials.8b00903
- Luo Y, Luo G, Gelinsky M, et al., 2017, 3D bioprinting scaffold using alginate/polyvinyl alcohol bioinks. Mater Lett, 189: 295–298. http://doi:10.1016/j.matlet.2016.12.009
- Asohan AW, Hashim R, Ku Ishak KM, et al., 2022, Preparation and characterisation of cellulose nanocrystal/ alginate/polyethylene glycol diacrylate (CNC/Alg/PEGDA) hydrogel using double network crosslinking technique for bioprinting application. Appl Sci, 12(2): 771. http://doi:10.3390/app12020771
- Hong S, Sycks D, Chan HF, et al., 2015, 3D printing of highly stretchable and tough hydrogels into complex, cellularized structures. Adv Mater, 27(27): 4035–4040. http://doi:10.1002/adma.201501099
- Kosik-Kozioł A, Costantini M, Bolek T, et al., 2017, PLA short sub-micron fiber reinforcement of 3D bioprinted alginate constructs for cartilage regeneration. Biofabrication, 9(4): 044105. http://doi:10.1088/1758-5090/aa90d7
- Bakhtiary N, Liu C, Ghorbani F, 2021, Bioactive inks development for osteochondral tissue engineering: A mini-review. Gels, 7(4): 274. http://doi:10.3390/gels7040274
- Das S, Pati F, Choi Y-J, et al., 2015, Bioprintable, cell-laden silk fibroin–gelatin hydrogel supporting multilineage differentiation of stem cells for fabrication of three-dimensional tissue constructs. Acta Biomater, 11: 233–246. http://doi:10.1016/j.actbio.2014.09.023
- Duan B, Kapetanovic E, Hockaday LAA, et al., 2014, Three-dimensional printed trileaflet valve conduits using biological hydrogels and human valve interstitial cells. Acta Biomater, 10(5): 1836–1846. http://doi:10.1016/j.actbio.2013.12.005
- Murphy SV, Skardal A, Atala A, 2013, Evaluation of hydrogels for bio-printing applications. J Biomed Mater Res - Part A, 101(1): 272–284. http://doi:10.1002/jbm.a.34326
- Williams D, Thayer P, Martinez H, et al., 2018, A perspective on the physical, mechanical and biological specifications of bioinks and the development of functional tissues in 3D bioprinting. Bioprinting, 9: 19–36. http://doi:10.1016/j.bprint.2018.02.003
- Tolabi H, Bakhtiary N, Sayadi S, et al., 2022, A critical review on polydopamine surface-modified scaffolds in musculoskeletal regeneration. Front Bioeng Biotechnol, 10: 1008360. http://doi:10.3389/fbioe.2022.1008360
- Diamantides N, Wang L, Pruiksma T, et al., 2017, Correlating rheological properties and printability of collagen bioinks: The effects of riboflavin photocrosslinking and pH. Biofabrication, 9(4): 034102. http://doi:10.1088/1758-5090/aa780f
- Moncal KK, Ozbolat V, Datta P, et al., 2019, Thermally-controlled extrusion-based bioprinting of collagen. J Mater Sci Mater Med, 30(5): 55. http://doi:10.1007/s10856-019-6258-2
- Rhee S, Puetzer JL, Mason BN, et al., 2016, 3D bioprinting of spatially heterogeneous collagen constructs for cartilage tissue engineering. ACS Biomater Sci Eng, 2(10): 1800–1805. http://doi:10.1021/acsbiomaterials.6b00288
- Leucht A, Volz A-C, Rogal J, et al., 2020, Advanced gelatin-based vascularization bioinks for extrusion-based bioprinting of vascularized bone equivalents. Sci Rep, 10(1): 5330. http://doi:10.1038/s41598-020-62166-w
- Osidak EO, Kozhukhov VI, Osidak MS, 2020, Collagen as bioink for bioprinting: A comprehensive review. Int J Bioprint, 6(3): 270. http://doi: 10.18063/ijb.v6i3.270
- de Melo BAG, Jodat YA, Cruz EM, et al., 2020, Strategies to use fibrinogen as bioink for 3D bioprinting fibrin-based soft and hard tissues. Acta Biomater, 117: 60–76. http://doi:10.1016/j.actbio.2020.09.024
- Zhou Z, Cui J, Wu S, et al., 2022, Silk fibroin-based biomaterials for cartilage/osteochondral repair. Theranostics, 12(11): 5103–5124. http://doi:10.7150/thno.74548
- Murphy SV, Atala A, 2014, 3D bioprinting of tissues and organs. Nat Biotechnol, 32(8): 773–785. http://doi:10.1038/nbt.2958
- Hölzl K, Lin S, Tytgat L, et al., 2016, Bioink properties before, during and after 3D bioprinting. Biofabrication, 8(3): 032002. http://doi:10.1088/1758-5090/8/3/032002
- Hinton TJ, Jallerat Q, Palchesko RN, et al., 2015, Three-dimensional printing of complex biological structures by freeform reversible embedding of suspended hydrogels. Sci Adv, 1(9): 1500758. http://doi:10.1126/sciadv.1500758
- Zhang J, Wehrle E, Adamek P, et al., 2020, Optimization of mechanical stiffness and cell density of 3D bioprinted cell-laden scaffolds improves extracellular matrix mineralization and cellular organization for bone tissue engineering. Acta Biomater, 114: 307–322. http://doi:10.1016/j.actbio.2020.07.016
- Martyniak K, Lokshina A, Cruz MA, et al., 2022, Biomaterial composition and stiffness as decisive properties of 3D bioprinted constructs for type II collagen stimulation. Acta Biomater, 152: 221–234. http://doi:10.1016/j.actbio.2022.08.058
- Allen NB, Abar B, Johnson L, et al., 2022, 3D-bioprinted GelMA-gelatin-hydroxyapatite osteoblast-laden composite hydrogels for bone tissue engineering. Bioprinting, 26: e00196. http://doi:10.1016/j.bprint.2022.e00196
- Lee JS, Park HS, Jung H, et al., 2020, Park, 3D-printable photocurable bioink for cartilage regeneration of tonsil-derived mesenchymal stem cells. Addit Manuf, 33: 101136. http://doi:10.1016/j.addma.2020.101136
- Bandyopadhyay A, Mandal BB, Bhardwaj N, 2022, 3D bioprinting of photo‐crosslinkable silk methacrylate (SilMA)‐polyethylene glycol diacrylate (PEGDA) bioink for cartilage tissue engineering. J Biomed Mater Res Part A, 110: 884–898. http://doi:10.1002/jbm.a.37336
- Rajput M, Mondal P, Yadav P, et al., 2022, Light-based 3D bioprinting of bone tissue scaffolds with tunable mechanical properties and architecture from photocurable silk fibroin. Int J Biol Macromol, 202: 644–656. http://doi:10.1016/j.ijbiomac.2022.01.081
- Unagolla JM, Jayasuriya AC, 2020, Hydrogel-based 3D bioprinting: A comprehensive review on cell-laden hydrogels, bioink formulations, and future perspectives. Appl Mater Today, 18: 100479. http://doi:10.1016/j.apmt.2019.100479
- Koo Y, Choi E-J, Lee J, et al., 2018, 3D printed cell-laden collagen and hybrid scaffolds for in vivo articular cartilage tissue regeneration. J Ind Eng Chem, 66: 343–355. http://doi:10.1016/j.jiec.2018.05.049
- Turnbull G, Clarke J, Picard F, et al., 2018, 3D bioactive composite scaffolds for bone tissue engineering. Bioact Mater, 3: 278–314. http://doi:10.1016/j.bioactmat.2017.10.001
- Zhang B, Huang J, Narayan RJ, 2020, Gradient scaffolds for osteochondral tissue engineering and regeneration. J Mater Chem B, 8: 8149–8170. http://doi:10.1039/D0TB00688B
- Diaz-Gomez L, Kontoyiannis PD, Melchiorri AJ, et al., 2019, Three-dimensional printing of tissue engineering scaffolds with horizontal pore and composition gradients. Tissue Eng Part C Methods, 25: 411–420. http://doi:10.1089/ten.tec.2019.0112
- Wang M, Li W, Mille LS, et al., 2022, Digital light processing based bioprinting with composable gradients. Adv Mater, 34: 2107038. http://doi:10.1002/adma.202107038
- Nicolas J, Magli S, Rabbachin L, et al., 2020, 3D extracellular matrix mimics: Fundamental concepts and role of materials chemistry to influence stem cell fate. Biomacromolecules, 21: 1968–1994. http://doi:10.1021/acs.biomac.0c00045
- Guvendiren M, Burdick JA, 2012, Stiffening hydrogels to probe short- and long-term cellular responses to dynamic mechanics. Nat Commun, 3: 792. http://doi:10.1038/ncomms1792
- Ji S, Almeida E, Guvendiren M, 2019, 3D bioprinting of complex channels within cell-laden hydrogels. Acta Biomater 95: 214–224. http://doi:10.1016/j.actbio.2019.02.038
- Martinez AW, Caves JM, Ravi S, et al., 2014, Effects of crosslinking on the mechanical properties, drug release and cytocompatibility of protein polymers. Acta Biomater, 10: 26–33. http://doi:10.1016/j.actbio.2013.08.029
- FitzSimons TM, Anslyn EV, Rosales AM, 2022, Effect of pH on the properties of hydrogels cross-linked via dynamic thia-michael addition bonds. ACS Polym Au, 2: 129–136. http://doi:10.1021/acspolymersau.1c00049
- Bustamante-Torres M, Romero-Fierro D, Arcentales-Vera B, et al., 2021, Hydrogels classification according to the physical or chemical interactions and as stimuli-sensitive materials. Gels, 7: 182. http://doi:10.3390/gels7040182
- Zhang YS, Khademhosseini A, 2017, Advances in engineering hydrogels. Science, 356: eaaf3627. http://doi:10.1126/science.aaf3627
- Lee J, Yeo M, Kim W, et al., 2018, Development of a tannic acid cross-linking process for obtaining 3D porous cell-laden collagen structure. Int J Biol Macromol, 110: 497–503. http://doi:10.1016/j.ijbiomac.2017.10.105
- Ruedinger F, Lavrentieva A, Blume C, et al., 2015, Hydrogels for 3D mammalian cell culture: a starting guide for laboratory practice. Appl Microbiol Biotechnol, 99: 623–636. http://doi:10.1007/s00253-014-6253-y
- Sang S, Mao X, Cao Y, et al., 2023, 3D bioprinting using synovium-derived MSC-laden photo-cross-linked ECM bioink for cartilage regeneration. ACS Appl Mater Interfaces, 15: 8895–8913. http://doi:10.1021/acsami.2c19058
- Singh YP, Bandyopadhyay A, Mandal BB, 2019, 3D bioprinting using cross-linker-free silk–gelatin bioink for cartilage tissue engineering. ACS Appl Mater Interfaces, 11: 33684–33696. http://doi:10.1021/acsami.9b11644
- Kara Özenler A, Distler T, Tihminlioglu F, et al., 2023, Fish scale containing alginate dialdehyde-gelatin bioink for bone tissue engineering. Biofabrication, 15: 025012. http://doi:10.1088/1758-5090/acb6b7
- Chakraborty J, Fernández-Pérez J, van Kampen KA, et al., 2023, Development of a biomimetic arch-like 3D bioprinted construct for cartilage regeneration using gelatin methacryloyl and silk fibroin-gelatin bioinks. Biofabrication, 15: 035009. http://doi:10.1088/1758-5090/acc68f
- Chen P, Zheng L, Wang Y, et al., 2019, Desktop-stereolithography 3D printing of a radially oriented extracellular matrix/mesenchymal stem cell exosome bioink for osteochondral defect regeneration. Theranostics, 9: 2439–2459. http://doi:10.7150/thno.31017
- Cidonio G, Alcala-Orozco CR, Lim KS, et al., 2019, Osteogenic and angiogenic tissue formation in high fidelity nanocomposite Laponite-gelatin bioinks. Biofabrication, 11: 035027. http://doi:10.1088/1758-5090/ab19fd
- Irmak G, Gümüşderelioğlu M, 2020, Photo-activated platelet-rich plasma (PRP)-based patient-specific bio-ink for cartilage tissue engineering. Biomed Mater, 15: 065010. http://doi:10.1088/1748-605X/ab9e46
- Bai Z, Guo X-H, Tang C, et al., 2018, Effects of artesunate on the expressions of insulin-like growth factor-1, osteopontin and C-telopeptides of type II collagen in a rat model of osteoarthritis. Pharmacology, 101: 1–8. http://doi:10.1159/000479160
- Street J, Bao M, DeGuzman L, et al., 2002, Vascular endothelial growth factor stimulates bone repair by promoting angiogenesis and bone turnover. Proc Natl Acad Sci, 99: 9656–9661. http://doi:10.1073/pnas.152324099
- Mi L, Liu H, Gao Y, et al., 2017, Injectable nanoparticles/ hydrogels composite as sustained release system with stromal cell-derived factor-1α for calvarial bone regeneration. Int J Biol Macromol, 101: 341–347. http://doi:10.1016/j.ijbiomac.2017.03.098
- Fujioka-Kobayashi M, Ota MS, Shimoda A, et al., 2012, Cholesteryl group- and acryloyl group-bearing pullulan nanogel to deliver BMP2 and FGF18 for bone tissue engineering. Biomaterials, 33: 7613–7620. http://doi:10.1016/j.biomaterials.2012.06.075
- Fahimipour F, Dashtimoghadam E, Mahdi Hasani-Sadrabadi M, et al., 2019, Enhancing cell seeding and osteogenesis of MSCs on 3D printed scaffolds through injectable BMP2 immobilized ECM-Mimetic gel. Dent Mater, 35: 990–1006. http://doi:10.1016/j.dental.2019.04.004
- Groll J, Burdick JA, Cho D-W, et al., 2018, A definition of bioinks and their distinction from biomaterial inks. Biofabrication, 11: 013001. http://doi:10.1088/1758-5090/aaec52
- Colombo M, Bianchi A, 2010, Click chemistry for the synthesis of RGD-containing integrin ligands. Molecules, 15: 178–197. http://doi:10.3390/molecules15010178
- Panwar A, Tan L, 2016, Current status of bioinks for micro-extrusion-based 3D bioprinting. Molecules, 21: 685. http://doi:10.3390/molecules21060685
- Byambaa B, Annabi N, Yue K, et al., 2017, Bioprinted osteogenic and vasculogenic patterns for engineering 3D bone tissue. Adv Healthc Mater, 6: 1700015. http://doi:10.1002/adhm.201700015
- Wang B, Díaz-Payno PJ, Browe DC, et al., 2021, Affinity-bound growth factor within sulfated interpenetrating network bioinks for bioprinting cartilaginous tissues. Acta Biomater, 128: 130–142. http://doi:10.1016/j.actbio.2021.04.016
- Xie M, Wu D, Li G, et al., 2021, Exosomes targeted towards applications in regenerative medicine. Nano Sel, 2: 880–908. http://doi:10.1002/nano.202000251
- Zhou Q, Cai Y, Lin X, 2020, The dual character of exosomes in osteoarthritis: Antagonists and therapeutic agents. Acta Biomater, 105: 15–25. http://doi:10.1016/j.actbio.2020.01.040
- Isaeva EV, Beketov EE, Demyashkin GA, et al., 2022, Cartilage formation in vivo using high concentration collagen-based bioink with MSC and decellularized ECM granules. Int J Mol Sci, 23: 2703. http://doi:10.3390/ijms23052703
- Marcu IC, Illaste A, Heuking P, et al., 2015, Functional characterization and comparison of intercellular communication in stem cell-derived cardiomyocytes. Stem Cells, 33: 2208–2218. http://doi:10.1002/stem.2009
- Tang J, Peng R, Ding J, 2010, The regulation of stem cell differentiation by cell-cell contact on micropatterned material surfaces. Biomaterials, 31: 2470–2476. http://doi:10.1016/j.biomaterials.2009.12.006
- Xu F, Sridharan B, Wang S, et al., 2011, Embryonic stem cell bioprinting for uniform and controlled size embryoid body formation. Biomicrofluidics, 5: 022207. http://doi:10.1063/1.3580752
- Bourget J-M, Kérourédan O, Medina M, et al., 2016, Patterning of endothelial cells and mesenchymal stem cells by laser-assisted bioprinting to study cell migration. Biomed Res Int, 2016: 1–7. http://doi:10.1155/2016/3569843
- Kolesky DB, Truby RL, Gladman AS, et al., 2014, 3D bioprinting of vascularized, heterogeneous cell-laden tissue constructs. Adv Mater, 26: 3124–3130. http://doi:10.1002/adma.201305506
- Jakus AE, Rutz AL, Shah RN, 2016, Advancing the field of 3D biomaterial printing. Biomed Mater, 11: 014102. http://doi:10.1088/1748-6041/11/1/014102
- Ovsianikov A, James Y, Vladimir M, 2018, 3D printing and biofabrication. Springer International Publishing.
- Morgan FLC, Moroni L, Baker MB, 2020, Dynamic bioinks to advance bioprinting. Adv Healthc Mater, 9: 1901798. http://doi:10.1002/adhm.201901798
- Nicodemus GD, Bryant SJ, 2008, Cell encapsulation in biodegradable hydrogels for tissue engineering applications. Tissue Eng Part B Rev, 14: 149–165. http://doi:10.1089/ten.teb.2007.0332
- Li H, Tan C, Li L, 2018, Review of 3D printable hydrogels and constructs. Mater Des, 159: 20–38. http://doi:10.1016/j.matdes.2018.08.023
- Mu X, Agostinacchio F, Xiang N, et al., 2021, Recent advances in 3D printing with protein-based inks. Prog Polym Sci, 115: 101375. http://doi:10.1016/j.progpolymsci.2021.101375
- Simorgh S, Milan PB, Saadatmand M, et al., 2021, Human olfactory mucosa stem cells delivery using a collagen hydrogel: As a potential candidate for bone tissue engineering. Materials (Basel), 14: 1–17. http://doi:10.3390/ma14143909
- Wu X, Rapoport TA, 2018, Mechanistic insights into ER-associated protein degradation. Curr Opin Cell Biol, 53: 22–28. http://doi:10.1016/j.ceb.2018.04.004
- Verbeek CJR, van den Berg LE, 2010, Extrusion processing and properties of protein-based thermoplastics. Macromol Mater Eng, 295(1): 10–21. http://doi:10.1002/mame.200900167
- Rutz AL, Lewis PL, Shah RN, 2017, Toward next-generation bioinks: Tuning material properties pre- and post-printing to optimize cell viability. MRS Bull, 42(8): 563–570. http://doi:10.1557/mrs.2017.162
- Kabirian F, Mozafari M, 2020, Decellularized ECM-derived bioinks: Prospects for the future. Methods, 171: 108–118. http://doi:10.1016/j.ymeth.2019.04.019
- Pugliese R, Beltrami B, Regondi S, et al., 2021, Polymeric biomaterials for 3D printing in medicine: An overview. Ann 3D Print Med, 2: 100011. http://doi:10.1016/j.stlm.2021.100011
- Parker ST, Domachuk P, Amsden J, et al., 2009, Biocompatible silk printed optical waveguides. Adv Mater, 21: 2411–2415. http://doi:10.1002/adma.200801580
- Ramesh S, Harrysson OLA, Rao PK, et al., 2021, Extrusion bioprinting: Recent progress, challenges, and future opportunities. Bioprinting, 21: e00116. http://doi:10.1016/j.bprint.2020.e00116
- Bedell ML, Navara AM, Du Y, et al., 2020, Polymeric systems for bioprinting. Chem Rev, 120(19): 10744–10792. http://doi:10.1021/acs.chemrev.9b00834
- Chakraborty J, Mu X, Pramanick A, et al., 2022, Recent advances in bioprinting using silk protein-based bioinks. Biomaterials, 287: 121672. http://doi:10.1016/j.biomaterials.2022.121672
- Zhou J, Zhang B, Shi L, et al., 2014, Regenerated silk fibroin films with controllable nanostructure size and secondary structure for drug delivery. ACS Appl Mater Interfaces, 6(24): 21813–21821. http://doi:10.1021/am502278b
- Nikoomanzari E, Karbasi M, Melo WCMA, et al., 2022, Impressive strides in antibacterial performance amelioration of Ti-based implants via plasma electrolytic oxidation (PEO): A review of the recent advancements. Chem Eng J, 441: 136003. http://doi:10.1016/j.cej.2022.136003
- Adhikari J, Roy A, Das A, et al., 2021, Effects of processing parameters of 3D bioprinting on the cellular activity of bioinks. Macromol Biosci, 21(1): 2000179. http://doi:10.1002/mabi.202000179
- Hull SM, Brunel LG, Heilshorn SC, 2022, 3D bioprinting of cell‐laden hydrogels for improved biological functionality. Adv Mater, 34(2): 2103691. http://doi:10.1002/adma.202103691