Evaluation of a synthetic peptide-based bioink (PeptiInk Alpha 1) for in vitro 3D bioprinting of cartilage tissue models
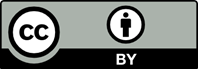
Cartilage pathology in human disease is poorly understood and requires further research. Various attempts have been made to study cartilage pathologies using in vitro human cartilage models as an alternative for preclinical research. Three-dimensional (3D) bioprinting is a technique that has been used to 3D-bioprint cartilage tissue models in vitro using animal-derived materials such as gelatine or hyaluronan, which present challenges in terms of scalability, reproducibility, and ethical concerns. We present an assessment of synthetic self-assembling peptides as bioinks for bioprinted human in vitro cartilage models. Primary human chondrocytes were mixed with PeptiInk Alpha 1, 3D-bioprinted and cultured for 14 days, and compared with 3D chondrocyte pellet controls. Cell viability was assessed through LIVE/DEAD assays and DNA quantification. High cell viability was observed in the PeptiInk culture, while a fast decrease in DNA levels was observed in the 3D pellet control. Histological evaluation using hematoxylin and eosin staining and immunofluorescence labeling for SOX-9, collagen type II, and aggrecan showed a homogeneous cell distribution in the 3D-bioprinted PeptiInks as well as high expression of chondrogenic markers in both control and PeptiInk cultures. mRNA expression levels assessed by - qRT-PCR (quantitative real time-polymerase chain reaction) confirmed chondrogenic cell behavior. These data showed promise in the potential use of PeptiInk Alpha 1 as a bioprintable manufacturing material for human cartilage in vitro models.
- Gibofsky A, 2012, Overview of epidemiology, pathophysiology, and diagnosis of rheumatoid arthritis. Am J Manag Care, 18(13 Suppl): S295–S302.
- Jafarzadeh SR, Felson DT, 2018, Updated estimates suggest a much higher prevalence of arthritis in United States adults than previous ones. Arthritis Rheumatol, 70(2): 185–192. https://pubmed.ncbi.nlm.nih.gov/29178176
- Buckwalter JA, Mow VC, Ratcliffe A, 1994, Restoration of injured or degenerated articular cartilage. J Am Acad Orthop Surg, 2(4): 192–201.
- O’Hara BP, Urban JP, Maroudas A, 1990, Influence of cyclic loading on the nutrition of articular cartilage. Ann Rheum Dis, 49(7): 536–539.
- Chang AA, Reuther MS, Briggs KK, et al., 2012, In vivo implantation of tissue-engineered human nasal septal neocartilage constructs: A pilot study. Otolaryngol Head Neck Surg, 146(1): 46–52. https://pubmed.ncbi.nlm.nih.gov/22031592
- Jin CZ, Cho JH, Choi BH, et al., 2011, The maturity of tissue-engineered cartilage in vitro affects the repairability for osteochondral defect. Tissue Eng Part A, 17(23–24): 3057– 3065. https://pubmed.ncbi.nlm.nih.gov/21736425
- Neybecker P, Henrionnet C, Pape E, et al., 2018, In vitro and in vivo potentialities for cartilage repair from human advanced knee osteoarthritis synovial fluid-derived mesenchymal stem cells. Stem Cell Res Ther, 9(1): 329. https://doi.org/10.1186/s13287-018-1071-2
- Sanjurjo-Rodríguez C, Castro-Viñuelas R, Hermida-Gómez T, et al., 2017, Human cartilage engineering in an in vitro repair model using collagen scaffolds and mesenchymal stromal cells. Int J Med Sci, 14(12): 1257–1262. https://pubmed.ncbi.nlm.nih.gov/29104482
- Wu Y, Kennedy P, Bonazza N, et al., 2021, Three-dimensional bioprinting of articular cartilage: A systematic review. Cartilage, 12(1): 76–92.
- Ng WL, Chua CK, Shen YF, 2019, Print me an organ! Why we are not there yet. Prog Polym Sci, 97(October 1): 101145. https://doi.org/10.1016/j.progpolymsci.2019.101145
- Ozbolat IT, Hospodiuk M, 2016, Current advances and future perspectives in extrusion-based bioprinting. Biomaterials, 76 (January 1): 321–343. http://dx.doi.org/10.1016/j.biomaterials.2015.10.076
- Suntornnond R, Ng WL, Huang X, et al., 2022, Improving printability of hydrogel-based bio-inks for thermal inkjet bioprinting applications via saponification and heat treatment processes. J Mater Chem B, 10(31): 5989–6000.
- Ng WL, Lee JM, Zhou M, et al., 2020, Vat polymerization-based bioprinting - process, materials, applications and regulatory challenges. Biofabrication, 12(2): 022001.
- Sekar MP, Budharaju H, Zennifer A, et al., 2021, Current standards and ethical landscape of engineered tissues- 3D bioprinting perspective. J Tissue Eng, 12 (July 1): 20417314211027676.
- Costantini M, Idaszek J, Szöke K, et al., 2016, 3D bioprinting of BM-MSCs-loaded ECM biomimetic hydrogels for in vitro neocartilage formation. Biofabrication, 8(3): 035002. https://iopscience.iop.org/article/10.1088/1758-5090/8/3/035002
- Daly AC, Critchley SE, Rencsok EM, et al., 2016, A comparison of different bioinks for 3D bioprinting of fibrocartilage and hyaline cartilage. Biofabrication, 8(4): 045002. https://iopscience.iop.org/article/10.1088/1758-5090/8/4/045002
- Duchi S, Onofrillo C, O’Connell CD, et al., 2017, Handheld co-axial bioprinting: Application to in situ surgical cartilage repair. Sci Rep, 7(1): 5837.
- Gao G, Schilling AF, Hubbell K, et al., 2015, Improved properties of bone and cartilage tissue from 3D inkjet-bioprinted human mesenchymal stem cells by simultaneous deposition and photocrosslinking in PEG-GelMA. Biotechnol Lett, 37(11): 2349–2355.
- Levato R, Visser J, Planell JA, et al., 2014, Biofabrication of tissue constructs by 3D bioprinting of cell-laden microcarriers. Biofabrication, 6(3): 035020. https://iopscience.iop.org/article/10.1088/1758-5082/6/3/035020
- Levato R, Webb WR, Otto IA, et al., 2017, The bio in the ink: Cartilage regeneration with bioprintable hydrogels and articular cartilage-derived progenitor cells. Acta Biomater, 61 (October 1): 41–53.
- Mouser VHM, Melchels FPW, Visser J, et al., 2016, Yield stress determines bioprintability of hydrogels based on gelatin-methacryloyl and gellan gum for cartilage bioprinting. Biofabrication, 8(3): 35003.
- O’Connell CD, Di Bella C, Thompson F, et al., 2016, Development of the Biopen: A handheld device for surgical printing of adipose stem cells at a chondral wound site. Biofabrication, 8(1): 15019.
- Zhu W, Cui H, Boualam B, et al., 2018, 3D bioprinting mesenchymal stem cell-laden construct with core-shell nanospheres for cartilage tissue engineering. Nanotechnology, 29(18): 185101.
- Abbadessa A, Mouser VHM, Blokzijl MM, et al., 2016, A synthetic thermosensitive hydrogel for cartilage bioprinting and its biofunctionalization with polysaccharides. Biomacromolecules, 17(6): 2137–2147. https://pubs.acs.org/doi/10.1021/acs.biomac.6b00366
- Wasyłeczko M, Sikorska W, Chwojnowski A, 2020, Review of synthetic and hybrid scaffolds in cartilage tissue engineering. Membranes, 10(11): 348. https://pubmed.ncbi.nlm.nih.gov/33212901
- Popov A, Malferrari S, Kalaskar DM, 2017, 3D bioprinting for musculoskeletal applications. J 3D Print Med, 1(3): 191–211. https://www.futuremedicine.com/doi/10.2217/3dp-2017-0004
- OECD, 2018, Guidance Document on Good In Vitro Method Practices (GIVIMP), Vol. 1, OECD Publishing, Paris, 1–264. https://www.oecd-ilibrary.org/docserver/9789264304796- en.pdf?expires=1621462493&id=id&accname=guest&checksum=4EA871101E844045CE8D833D9DFADC25%0A https://www.oecd-ilibrary.org/environment/guidance-document-on-good-in-vitro-method-practices-givimp_9789264304
- Ligorio C, Hoyland JA, Saiani A, 2022, Self-assembling peptide hydrogels as functional tools to tackle intervertebral disc degeneration. Gels, 8(4). https://www.mdpi.com/2310-2861/8/4/211
- Mujeeb A, Miller AF, Saiani A, Gough JE. 2013; Self-assembled octapeptide scaffolds for in vitro chondrocyte culture. 9(1):4609–17. 2013. Acta Biomater [Internet]. Available from: http://dx.doi.org/10.1016/j.actbio.2012.08.044
- Wan S, Borland S, Richardson SM, et al., 2016, Self-assembling peptide hydrogel for intervertebral disc tissue engineering. Acta Biomater, 46(December 1): 29–40. https://www.sciencedirect.com/science/article/pii/ S1742706116305062
- Raphael B, Khalil T, Workman VL, et al., 2017, 3D cell bioprinting of self-assembling peptide-based hydrogels. Mater Lett, 190: 103–6.
- Gatenholm B, Lindahl C, Brittberg M, et al., 2020, Collagen 2A type B induction after 3D bioprinting chondrocytes in situ into osteoarthritic chondral tibial lesion. Cartilage, 13(December 1): 1–15. http://journals.sagepub.com/doi/10.1177/1947603520903788
- Yang IH, Kim SH, Kim YH, et al., 2004, Comparison of phenotypic characterization between “alginate bead” and “pellet” culture systems as chondrogenic differentiation models for human mesenchymal stem cells. Yonsei Med J, 45: 891–900. http://www.ncbi.nlm.nih.gov/pubmed/15515201
- Yeung P, Cheng KH, Yan CH, et al., 2019, Collagen microsphere based 3D culture system for human osteoarthritis chondrocytes (hOACs). Sci Rep, 9(1): 1–14. http://dx.doi.org/10.1038/s41598-019-47946-3
- Ligorio C, Zhou M, Wychowaniec JK, et al., 2019, Graphene oxide containing self-assembling peptide hybrid hydrogels as a potential 3D injectable cell delivery platform for intervertebral disc repair applications. Acta Biomater, 92: 92–103. https://doi.org/10.1016/j.actbio.2019.05.004
- Lemarié L, Anandan A, Petiot E, et al., 2021, Rheology, simulation and data analysis toward bioprinting cell viability awareness. Bioprinting, 21(December 2020): e00119. ht tps : / / l inkinghub. el s evi e r. com/ re t r i eve /pi i / S2405886620300464
- Schmitz C, Potekhina E, Belousov VV, et al., 2021, Hypoxia onset in mesenchymal stem cell spheroids: monitoring with hypoxia reporter cells. Front Bioeng Biotechnol, 9(February).
- Caron MMJ, Emans PJ, Coolsen MME, et al., 2012, Redifferentiation of dedifferentiated human articular chondrocytes: Comparison of 2D and 3D cultures. Osteoarthr Cartil, 20(10): 1170–1178. http://dx.doi.org/10.1016/j.joca.2012.06.016
- Haseeb A, Kc R, Angelozzi M, et al., 2021, SOX9 keeps growth plates and articular cartilage healthy by inhibiting chondrocyte dedifferentiation/osteoblastic redifferentiation. Proc Natl Acad Sci, 118(8): e2019152118. https://www.pnas.org/doi/abs/10.1073/pnas.2019152118
- Schon BS, Schrobback K, Van Der Ven M, et al., 2012, Validation of a high-throughput microtissue fabrication process for 3D assembly of tissue engineered cartilage constructs. Cell Tissue Res, 347(3): 629–642.
- Bosnakovski D, Mizuno M, Kim G, et al., 2004, Chondrogenic differentiation of bovine bone marrow mesenchymal stem cells in pellet cultural system. Exp Hematol, 32(5): 502–509.
- Griffin MF, Ibrahim A, Seifalian AM, et al., 2017, Chemical group-dependent plasma polymerisation preferentially directs adipose stem cell differentiation towards osteogenic or chondrogenic lineages. Acta Biomater, 50: 450–61. http://dx.doi.org/10.1016/j.actbio.2016.12.016
- Zhang Z, McCaffery JM, Spencer RGS, et al., 2004, Hyaline cartilage engineered by chondrocytes in pellet culture: Histological, immunohistochemical and ultrastructural analysis in comparison with cartilage explants. J Anat, 205(3): 229–237.
- Faroni A, Workman VL, Saiani A, et al., 2019, Self-assembling peptide hydrogel matrices improve the neurotrophic potential of human adipose-derived stem cells. Adv Healthc Mater, 8(17).
- Benya P, 1982, Dedifferentiated chondrocytes reexpress the differentiated collagen phenotype when cultured in agarose gels. Cell, 30(1): 215–224. https://doi.org/10.1016%2F0092-8674%2882%2990027-7
- Lemare F, Steimberg N, Le Griel C, et al., 1998, Dedifferentiated chondrocytes cultured in alginate beads: Restoration of the differentiated phenotype and of the metabolic responses to interleukin- 1β. J Cell Physiol, 176(2): 303–313.