Advances in 3D printing techniques for cartilage regeneration of temporomandibular joint disc and mandibular condyle
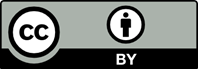
Temporomandibular joint (TMJ) osteoarthritis causes fibrocartilage damage to the TMJ disc and mandibular condyle, resulting in local pain and functional impairment that further reduces patients’ quality of life. Tissue engineering offers a potential treatment for fibrocartilage regeneration of the TMJ disc and mandibular condyle. However, the heterogeneous structure of TMJ fibrocartilage tissue poses significant challenges for the fabrication of biomimetic scaffolds. Over the past two decades, some researchers have attempted to adopt three-dimensional (3D) printing techniques to fabricate biomimetic scaffolds for TMJ fibrocartilage regeneration, but publications on such attempts are limited and rarely report satisfactory results, indicating an urgent need for further development. This review outlines several popular 3D printing techniques and the significant elements of tissue-engineered scaffolds: seed cells, scaffold materials, and bioactive factors. Current research progress on 3D-printed scaffolds for fibrocartilage regeneration of the TMJ disc and mandibular condyle is reviewed. The current challenges in TMJ tissue engineering are mentioned along with some emerging tissue-engineering strategies, such as machine learning, stimuli-responsive delivery systems, and extracellular vesicles, which are considered as potential approaches to improve the performance of 3D-printed scaffolds for TMJ fibrocartilage regeneration. This review is expected to inspire the further development of 3D printing techniques for TMJ fibrocartilage regeneration.
Alomar X, Medrano J, Cabratosa J, et al., 2007, Anatomy of the temporomandibular joint. Semin Ultrasound CT MR, 28(3): 170–183. http://doi.org/10.1053/j.sult.2007.02.002
de Souza RF, Lovato da Silva CH, Nasser M, et al., 2012, Interventions for the management of temporomandibular joint osteoarthritis. Cochrane Database Syst Rev, 2012(4): Cd007261. http://doi.org/10.1002/14651858.CD007261.pub2
Valesan LF, Da-Cas CD, Réus JC, et al., 2021, Prevalence of temporomandibular joint disorders: A systematic review and meta-analysis. Clin Oral Investig, 25(2): 441–53 http://doi.org/10.1007/s00784-020-03710-w
Tanaka E, Detamore MS, Mercuri LG, 2008, Degenerative disorders of the temporomandibular joint: Etiology, diagnosis, and treatment. J Dent Res, 87(4): 296–307 http://doi.org/10.1177/154405910808700406
Fang L, Ye Y, Tan X, et al., 2021, Overloading stress-induced progressive degeneration and self-repair in condylar cartilage. Ann N Y Acad Sci, 1503(1): 72–87 http://doi.org/10.1111/nyas.14606
Lee KS, Kwak HJ, Oh JM, et al., 2020, Automated detection of TMJ osteoarthritis based on artificial intelligence. J Dent Res, 99(12): 1363–7 http://doi.org/10.1177/0022034520936950
Wang XD, Zhang JN, Gan YH, et al., 2015, Current understanding of pathogenesis and treatment of TMJ osteoarthritis. J Dent Res, 94(5): 666–73 http://doi.org/10.1177/0022034515574770
Resnick CM, 2018, Temporomandibular joint reconstruction in the growing child. Oral Maxillofac Surg Clin North Am, 30(1): 109–21 http://doi.org/10.1016/j.coms.2017.08.006
Haene R, Qamirani E, Story RA, et al., 2012, Intermediate outcomes of fresh talar osteochondral allografts for treatment of large osteochondral lesions of the talus. J Bone Joint Surg Am, 94(12): 1105–10 http://doi.org/10.2106/jbjs.J.02010
Johnson NR, Roberts MJ, Doi SA, et al., 2017, Total temporomandibular joint replacement prostheses: A systematic review and bias-adjusted meta-analysis. Int J Oral Maxillofac Surg, 46(1): 86–92 http://doi.org/10.1016/j.ijom.2016.08.022
Armiento AR, Stoddart MJ, Alini M, et al., 2018, Biomaterials for articular cartilage tissue engineering: Learning from biology. Acta Biomater, 65: 1–20. http://doi.org/10.1016/j.actbio.2017.11.021
O’Shea DG, Curtin CM, O’Brien FJ, 2022, Articulation inspired by nature: A review of biomimetic and biologically active 3D printed scaffolds for cartilage tissue engineering. Biomater Sci, 10(10): 2462–83. http://doi.org/10.1039/d1bm01540k
Van Bellinghen X, Idoux-Gillet Y, Pugliano M, et al., 2018, Temporomandibular joint regenerative medicine. Int J Mol Sci, 19(2): 446. http://doi.org/10.3390/ijms19020446
Trindade D, Cordeiro R, José HC, et al., 2021, Biological treatments for temporomandibular joint disc disorders: Strategies in tissue engineering. Biomolecules, 11(7): 933. http://doi.org/10.3390/biom11070933
Perera K, Ivone R, Natekin E, et al., 2021, 3D bioprinted implants for cartilage repair in intervertebral discs and knee menisci. Front Bioeng Biotechnol, 9: 754113. http://doi.org/10.3389/fbioe.2021.754113
Doyle SE, Snow F, Duchi S, et al., 2021, 3D printed multiphasic scaffolds for osteochondral repair: Challenges and opportunities. Int J Mol Sci, 22(22): 12420. http://doi.org/10.3390/ijms222212420
Wang S, Zhao S, Yu J, et al., 2022, Advances in translational 3D printing for cartilage, bone, and osteochondral tissue engineering. Small, 18(36): e2201869. http://doi.org/10.1002/smll.202201869
McMillan A, McMillan N, Gupta N, et al., 2023, 3D bioprinting in otolaryngology: A review. Adv Healthc Mater, e2203268. http://doi.org/10.1002/adhm.202203268
Yang Z, Yi P, Liu Z, et al., 2022, Stem cell-laden hydrogel-based 3D bioprinting for bone and cartilage tissue engineering. Front Bioeng Biotechnol, 10: 865770. http://doi.org/10.3389/fbioe.2022.865770
Zub K, Hoeppener S, Schubert US, 2022, Inkjet printing and 3D printing strategies for biosensing, analytical, and diagnostic applications. Adv Mater, 34(31): e2105015. http://doi.org/10.1002/adma.202105015
Gudapati H, Dey M, Ozbolat I, 2016, A comprehensive review on droplet-based bioprinting: Past, present and future. Biomaterials, 102: 20–42. http://doi.org/10.1016/j.biomaterials.2016.06.012
Sohrabi S, Liu Y, 2018, Modeling thermal inkjet and cell printing process using modified pseudopotential and thermal lattice Boltzmann methods. Phys Rev E, 97(3–1): 033105. http://doi.org/10.1103/PhysRevE.97.033105
Mandrycky C, Wang Z, Kim K, et al., 2016, 3D bioprinting for engineering complex tissues. Biotechnol Adv, 34(4): 422–34. http://doi.org/10.1016/j.biotechadv.2015.12.011
Guo Q, Su X, Zhang X, et al., 2021, A review on acoustic droplet ejection technology and system. Soft Matter, 17(11): 3010–21. http://doi.org/10.1039/d0sm02193h
Derakhshanfar S, Mbeleck R, Xu K, et al., 2018, 3D bioprinting for biomedical devices and tissue engineering: A review of recent trends and advances. Bioact Mater, 3(2): 144–56. http://doi.org/10.1016/j.bioactmat.2017.11.008
Zhang YS, Haghiashtiani G, Hübscher T, et al., 2021, 3D extrusion bioprinting. Nat Rev Methods Primers, 1(1): 75. http://doi.org/10.1038/s43586-021-00073-8
Liu H, Zhou H, Lan H, et al., 2018, Multinozzle multichannel temperature deposition system for construction of a blood vessel. SLAS Technol, 23(1): 64–9. http://doi.org/10.1177/2472630317712221
Hong S, Kim JS, Jung B, et al., 2019, Coaxial bioprinting of cell-laden vascular constructs using a gelatin-tyramine bioink. Biomater Sci, 7(11): 4578–87. http://doi.org/10.1039/c8bm00618k
Daly AC, Freeman FE, Gonzalez-Fernandez T, et al., 2017, 3D bioprinting for cartilage and osteochondral tissue engineering. Adv Healthc Mater, 6(22): 1700298. http://doi.org/10.1002/adhm.201700298
Li M, Sun D, Zhang J, et al., 2022, Application and development of 3D bioprinting in cartilage tissue engineering. Biomater Sci, 10(19):5430–58. http://doi.org/10.1039/d2bm00709f
Ozbolat IT, Hospodiuk M, 2016, Current advances and future perspectives in extrusion-based bioprinting. Biomaterials, 76: 321–43. http://doi.org/10.1016/j.biomaterials.2015.10.076
Heinrich MA, Liu W, Jimenez A, et al., 2019, 3D bioprinting: From benches to translational applications. Small, 15(23): e1805510. http://doi.org/10.1002/smll.201805510
Kačarević ŽP, Rider PM, Alkildani S, et al., 2018, An introduction to 3D bioprinting: Possibilities, challenges and future aspects. Materials (Basel), 11(11): 2199. http://doi.org/10.3390/ma11112199
Bohandy JB, Kim BF, Adrian FJ, 1986, Metal deposition from a supported metal film using an excimer laser. J Appl Phys, 60(4): 1538–9. http://doi.org/10.1063/1.337287
Li J, Chen M, Fan X, et al., 2016, Recent advances in bioprinting techniques: Approaches, applications and future prospects. J Transl Med, 14: 271. http://doi.org/10.1186/s12967-016-1028-0
Catros S, Guillotin B, BacÁková M, et al., 2011, Effect of laser energy, substrate film thickness and bioink viscosity on viability of endothelial cells printed by laser-assisted bioprinting. Appl Surf Sci, 257(12): 5142–7. http://doi.org/10.1016/j.apsusc.2010.11.049
Su X, Wang T, Guo S, 2021, Applications of 3D printed bone tissue engineering scaffolds in the stem cell field. Regen Ther, 16: 63–72. http://doi.org/10.1016/j.reth.2021.01.007
Zhang J, Hu Q, Wang S, et al., 2020, Digital light processing based three-dimensional printing for medical applications. Int J Bioprint, 6(1): 242. http://doi.org/10.18063/ijb.v6i1.242
Wu Y, Su H, Li M, et al., 2022, Digital light processing-based multi-material bioprinting: Processes, applications, and perspectives. J Biomed Mater Res A, 111(4): 527–42. http://doi.org/10.1002/jbm.a.37473
Kadry H, Wadnap S, Xu C, et al., 2019, Digital light processing (DLP) 3D-printing technology and photoreactive polymers in fabrication of modified-release tablets. Eur J Pharm Sci, 135: 60–7. http://doi.org/10.1016/j.ejps.2019.05.008
Ravanbakhsh H, Karamzadeh V, Bao G, et al., 2021, Emerging technologies in multi-material bioprinting. Adv Mater, 33(49): e2104730. http://doi.org/10.1002/adma.202104730
Godbey WT, Hindy SB, Sherman ME, et al., 2004, A novel use of centrifugal force for cell seeding into porous scaffolds. Biomaterials, 25(14): 2799–2805. http://doi.org/10.1016/j.biomaterials.2003.09.056
Griffon DJ, Abulencia JP, Ragetly GR, et al., 2011, A comparative study of seeding techniques and three-dimensional matrices for mesenchymal cell attachment. J Tissue Eng Regen Med, 5(3): 169–179. http://doi.org/10.1002/term.302
Collon K, Bell JA, Chang SW, et al., 2022, Effects of cell seeding technique and cell density on BMP-2 production in transduced human mesenchymal stem cells. J Biomed Mater Res A, 110(12): 1944–1952. http://doi.org/10.1002/jbm.a.37430
Anderson DE, Athanasiou KA, 2009, A comparison of primary and passaged chondrocytes for use in engineering the temporomandibular joint. Arch Oral Biol, 54(2): 138–145. http://doi.org/10.1016/j.archoralbio.2008.09.018
Anderson DE, Athanasiou KA, 2008, Passaged goat costal chondrocytes provide a feasible cell source for temporomandibular joint tissue engineering. Ann Biomed Eng, 36(12): 1992–2001. http://doi.org/10.1007/s10439-008-9572-2
Vapniarsky N, Huwe LW, Arzi B, et al., 2018, Tissue engineering toward temporomandibular joint disc regeneration. Sci Transl Med, 10(446): eaaq1802. http://doi.org/10.1126/scitranslmed.aaq1802
Kalpakci KN, Kim EJ, Athanasiou KA, 2011, Assessment of growth factor treatment on fibrochondrocyte and chondrocyte co-cultures for TMJ fibrocartilage engineering. Acta Biomater, 7(4): 1710–1718. http://doi.org/10.1016/j.actbio.2010.12.015
Cui D, Li H, Xu X, et al., 2017, Mesenchymal stem cells for cartilage regeneration of TMJ osteoarthritis. Stem Cells Int, 2017: 5979741. http://doi.org/10.1155/2017/5979741
Bousnaki M, Bakopoulou A, Papadogianni D, et al., 2018, Fibro/chondrogenic differentiation of dental stem cells into chitosan/alginate scaffolds towards temporomandibular joint disc regeneration. J Mater Sci Mater Med, 29(7): 97.http://doi.org/10.1007/s10856-018-6109-6
Park YB, Ha CW, Lee CH, et al., 2017, Restoration of a large osteochondral defect of the knee using a composite of umbilical cord blood-derived mesenchymal stem cells and hyaluronic acid hydrogel: A case report with a 5-year follow-up. BMC Musculoskelet Disord, 18(1): 59. http://doi.org/10.1186/s12891-017-1422-7
Liu J, Nie H, Xu Z, et al., 2014, The effect of 3D nanofibrous scaffolds on the chondrogenesis of induced pluripotent stem cells and their application in restoration of cartilage defects. PLoS One, 9(11): e111566. http://doi.org/10.1371/journal.pone.0111566
Zhang J, Guo F, Mi J, et al., 2014, Periodontal ligament mesenchymal stromal cells increase proliferation and glycosaminoglycans formation of temporomandibular joint derived fibrochondrocytes. Biomed Res Int, 2014: 410167. http://doi.org/10.1155/2014/410167
Ogasawara N, Kano F, Hashimoto N, et al., 2020, Factors secreted from dental pulp stem cells show multifaceted benefits for treating experimental temporomandibular joint osteoarthritis. Osteoarthr Cartil, 28(6): 831–841. http://doi.org/10.1016/j.joca.2020.03.010
Sun AX, Lin H, Fritch MR, et al., 2017, Chondrogenesis of human bone marrow mesenchymal stem cells in 3-dimensional, photocrosslinked hydrogel constructs: Effect of cell seeding density and material stiffness. Acta Biomater, 58: 302–311. http://doi.org/10.1016/j.actbio.2017.06.016
Sophia Fox AJ, Bedi A, Rodeo SA, 2009, The basic science of articular cartilage: Structure, composition, and function. Sports Health, 1(6): 461–468. http://doi.org/10.1177/1941738109350438
Hunziker EB, Quinn TM, Häuselmann HJ, 2002, Quantitative structural organization of normal adult human articular cartilage. Osteoarthr Cartil, 10(7): 564–572. http://doi.org/10.1053/joca.2002.0814
Ren X, Wang F, Chen C, et al., 2016, Engineering zonal cartilage through bioprinting collagen type II hydrogel constructs with biomimetic chondrocyte density gradient. BMC Musculoskelet Disord, 17: 301. http://doi.org/10.1186/s12891-016-1130-8
Talukdar S, Nguyen QT, Chen AC, et al., 2011, Effect of initial cell seeding density on 3D-engineered silk fibroin scaffolds for articular cartilage tissue engineering. Biomaterials, 32(34): 8927–8937. http://doi.org/10.1016/j.biomaterials.2011.08.027
Lam T, Dehne T, Krüger JP, et al., 2019, Photopolymerizable gelatin and hyaluronic acid for stereolithographic 3D bioprinting of tissue-engineered cartilage. J Biomed Mater Res B Appl Biomater, 107(8): 2649–2657. http://doi.org/10.1002/jbm.b.34354
Li X, Teng Y, Liu J, et al., 2017, Chondrogenic differentiation of BMSCs encapsulated in chondroinductive polysaccharide/ collagen hybrid hydrogels. J Mater Chem B, 5(26): 5109–5119. http://doi.org/10.1039/c7tb01020f
Kim M, Erickson IE, Huang AH, et al., 2018, Donor variation and optimization of human mesenchymal stem cell chondrogenesis in hyaluronic acid. Tissue Eng Part A, 24(21-22): 1693–1703. http://doi.org/10.1089/ten.TEA.2017.0520
Wang D, Qi Y, Wang Z, et al., 2022, Recent advances in animal models, diagnosis, and treatment of temporomandibular joint osteoarthritis. Tissue Eng Part B Rev, 29(1): 62–77. http://doi.org/10.1089/ten.TEB.2022.0065
Yang X, Lu Z, Wu H, et al., 2018, Collagen-alginate as bioink for three-dimensional (3D) cell printing based cartilage tissue engineering. Mater Sci Eng C Mater Biol Appl, 83: 195–201. http://doi.org/10.1016/j.msec.2017.09.002
Huber F, Vollmer D, Vinke J, et al., 2022, Influence of 3D printing parameters on the mechanical stability of PCL scaffolds and the proliferation behavior of bone cells. Materials (Basel), 15(6): 2091. http://doi.org/10.3390/ma15062091
Lu J, Huang J, Jin J, et al., 2022, The design and characterization of a strong bio-ink for meniscus regeneration. Int J Bioprint, 8(4): 600. http://doi.org/10.18063/ijb.v8i4.600
Li P, Fu L, Liao Z, et al., 2021, Chitosan hydrogel/3D-printed poly(ε-caprolactone) hybrid scaffold containing synovial mesenchymal stem cells for cartilage regeneration based on tetrahedral framework nucleic acid recruitment. Biomaterials, 278: 121131. http://doi.org/10.1016/j.biomaterials.2021.121131
Shen H, Hu X, 2021, Growth factor loading on aliphatic polyester scaffolds. RSC Adv, 11(12): 6735–6747. http://doi.org/10.1039/d0ra10232f
Acri TM, Shin K, Seol D, et al., 2019, Tissue engineering for the temporomandibular joint. Adv Healthc Mater, 8(2): e1801236. http://doi.org/10.1002/adhm.201801236
Luo C, Xie R, Zhang J, et al., 2020, Low-temperature three-dimensional printing of tissue cartilage engineered with gelatin methacrylamide. Tissue Eng Part C Methods, 26(6): 306–316. http://doi.org/10.1089/ten.TEC.2020.0053
Tarafder S, Koch A, Jun Y, et al., 2016, Micro-precise spatiotemporal delivery system embedded in 3D printing for complex tissue regeneration. Biofabrication, 8(2): 025003. http://doi.org/10.1088/1758-5090/8/2/025003
Dormer NH, Busaidy K, Berkland CJ, et al., 2011, Osteochondral interface regeneration of rabbit mandibular condyle with bioactive signal gradients. J Oral Maxillofac Surg, 69(6): e50–57. http://doi.org/10.1016/j.joms.2010.12.049
Kalpakci KN, Willard VP, Wong ME, et al., 2011, An interspecies comparison of the temporomandibular joint disc. J Dent Res, 90(2): 193–198. http://doi.org/10.1177/0022034510381501
Kuo J, Zhang L, Bacro T, et al., 2010, The region-dependent biphasic viscoelastic properties of human temporomandibular joint discs under confined compression. J Biomech, 43(7): 1316–1321. http://doi.org/10.1016/j.jbiomech.2010.01.020
Legemate K, Tarafder S, Jun Y, et al., 2016, Engineering human TMJ discs with protein-releasing 3D-printed scaffolds. J Dent Res, 95(7): 800–807. http://doi.org/10.1177/0022034516642404
Lee CH, Rodeo SA, Fortier LA, et al., 2014, Protein-releasing polymeric scaffolds induce fibrochondrocytic differentiation of endogenous cells for knee meniscus regeneration in sheep. Sci Transl Med, 6(266): 266ra171. http://doi.org/10.1126/scitranslmed.3009696
Moura C, Trindade D, Vieira M, et al., 2020, Multi-material implants for temporomandibular joint disc repair: Tailored additive manufacturing production. Front Bioeng Biotechnol, 8: 342. http://doi.org/10.3389/fbioe.2020.00342
Ângelo DF, Wang Y, Morouço P, et al., 2021, A randomized controlled preclinical trial on 3 interposal temporomandibular joint disc implants: TEMPOJIMS-Phase 2. J Tissue Eng Regen Med, 15(10): 852–868. http://doi.org/10.1002/term.3230
Jiang N, Yang Y, Zhang L, et al., 2021, 3D-printed polycaprolactone reinforced hydrogel as an artificial TMJ disc. J Dent Res, 100(8): 839–846. http://doi.org/10.1177/00220345211000629
Yi P, Liang J, Huang F, et al., 2021, Composite system of 3D-printed polymer and acellular matrix hydrogel to repair temporomandibular joint disc. Front Mater, 8: 621416. http://doi.org/10.3389/fmats.2021.621416
Wang L, Lazebnik M, Detamore MS, 2009, Hyaline cartilage cells outperform mandibular condylar cartilage cells in a TMJ fibrocartilage tissue engineering application. Osteoarthr Cartil, 17(3): 346–353. http://doi.org/10.1016/j.joca.2008.07.004
Stocum DL, Roberts WE, 2018, Part I: Development and physiology of the temporomandibular joint. Curr Osteoporos Rep, 16(4): 360–368. http://doi.org/10.1007/s11914-018-0447-7
Singh M, Detamore MS, 2009, Biomechanical properties of the mandibular condylar cartilage and their relevance to the TMJ disc. J Biomech, 42(4): 405–417. http://doi.org/10.1016/j.jbiomech.2008.12.012
Smith MH, Flanagan CL, Kemppainen JM, et al., 2007, Computed tomography-based tissue-engineered scaffolds in craniomaxillofacial surgery. Int J Med Robot, 3(3): 207–216. http://doi.org/10.1002/rcs.143
Abramowicz S, Crotts SJ, Hollister SJ, et al., 2021, Tissue-engineered vascularized patient-specific temporomandibular joint reconstruction in a Yucatan pig model. Oral Surg Oral Med Oral Pathol Oral Radiol, 132(2): 145–152. http://doi.org/10.1016/j.oooo.2021.02.002
Williams JM, Adewunmi A, Schek RM, et al., 2005, Bone tissue engineering using polycaprolactone scaffolds fabricated via selective laser sintering. Biomaterials, 26(23): 4817–4827. http://doi.org/10.1016/j.biomaterials.2004.11.057
Ciocca L, Donati D, Fantini M, et al., 2013, CAD-CAM-generated hydroxyapatite scaffold to replace the mandibular condyle in sheep: Preliminary results. J Biomater Appl, 28(2): 207–218. http://doi.org/10.1177/0885328212443296
Schek R, Taboas J, Hollister S, et al., 2005, Tissue engineering osteochondral implants for temporomandibular joint repair. Orthod Craniofac Res, 8(4): 313–319. http://doi. org/ht tps://doi. org/10.1111/j.1601- 6343.2005.00354.x
Wang F, Hu Y, He D, et al., 2017, Regeneration of subcutaneous tissue-engineered mandibular condyle in nude mice. J Craniomaxillofac Surg, 45(6): 855–861. http://doi.org/10.1016/j.jcms.2017.03.017
Helgeland E, Rashad A, Campodoni E, et al., 2021, Dual-crosslinked 3D printed gelatin scaffolds with potential for temporomandibular joint cartilage regeneration. Biomed Mater, 16(3): 035026. http://doi.org/10.1088/1748-605X/abe6d9
Helgeland E, Mohamed-Ahmed S, Shanbhag S, et al., 2021, 3D printed gelatin-genipin scaffolds for temporomandibular joint cartilage regeneration. Biomed Phys Eng Express, 7(5): 055025. http://doi.org/10.1088/2057-1976/ac1e68
Donahue RP, Hu JC, Athanasiou KA, 2019, Remaining hurdles for tissue-engineering the temporomandibular joint disc. Trends Mol Med, 25(3): 241–256. http://doi.org/10.1016/j.molmed.2018.12.007
Murphy SV, De Coppi P, Atala A, 2020, Opportunities and challenges of translational 3D bioprinting. Nat Biomed Eng, 4(4): 370–380. http://doi.org/10.1038/s41551-019-0471-7
Chuong R, Piper MA, 1992, Cerebrospinal fluid leak associated with proplast implant removal from the temporomandibular joint. Oral Surg Oral Med Oral Pathol, 74(4): 422–425. http://doi.org/10.1016/0030-4220(92)90286-y
Bielajew BJ, Donahue RP, Espinosa MG, et al., 2021, Knee orthopedics as a template for the temporomandibular joint. Cell Rep Med, 2(5): 100241. http://doi.org/10.1016/j.xcrm.2021.100241
She Y, Tang S, Zhu Z, et al., 2022, Comparison of temporomandibular joint disc, meniscus, and intervertebral disc in fundamental characteristics and tissue engineering. J Biomed Mater Res B Appl Biomater, 111(3): 717–729. http://doi.org/10.1002/jbm.b.35178
Malekpour A, Chen X, 2022, Printability and cell viability in extrusion-based bioprinting from experimental, computational, and machine learning views. J Funct Biomater, 13(2): 40. http://doi.org/10.3390/jfb13020040
Conev A, Litsa EE, Perez MR, et al., 2020, Machine learning-guided three-dimensional printing of tissue engineering scaffolds. Tissue Eng Part A, 26(23–24): 1359–1368. http://doi.org/10.1089/ten.TEA.2020.0191
Ruberu K, Senadeera M, Rana S, et al., 2021, Coupling machine learning with 3D bioprinting to fast track optimisation of extrusion printing. Appl Mater Today, 22: 100914. http://doi.org/10.1016/j.apmt.2020.100914
Reina-Romo E, Mandal S, Amorim P, et al., 2021, Towards the experimentally-informed in silico nozzle design optimization for extrusion-based bioprinting of shear-thinning hydrogels. Front Bioeng Biotechnol, 9: 701778. http://doi.org/10.3389/fbioe.2021.701778
Caballero Aguilar LM, Silva SM, Moulton SE, 2019, Growth factor delivery: Defining the next generation platforms for tissue engineering. J Control Release, 306: 40–58. http://doi.org/10.1016/j.jconrel.2019.05.028
Zhang M, Hu W, Cai C, et al., 2022, Advanced application of stimuli-responsive drug delivery system for inflammatory arthritis treatment. Materials Today Bio, 14: 100223. http://doi.org/10.1016/j.mtbio.2022.100223
Azagarsamy MA, Anseth KS, 2013, Wavelength-controlled photocleavage for the orthogonal and sequential release of multiple proteins. Angew Chem Int Ed Engl, 52(51): 13803–13807. http://doi.org/10.1002/anie.201308174
Chen P, Zheng L, Wang Y, et al., 2019, Desktop-stereolithography 3D printing of a radially oriented extracellular matrix/mesenchymal stem cell exosome bioink for osteochondral defect regeneration. Theranostics, 9(9): 2439–2459. http://doi.org/10.7150/thno.31017
Ng CY, Chai JY, Foo JB, et al., 2021, Potential of exosomes as cell-free therapy in articular cartilage regeneration: A review. Int J Nanomed, 16: 6749–6781. http://doi.org/10.2147/ijn.S327059
Feng ZY, Zhang QY, Tan J, et al., 2022, Techniques for increasing the yield of stem cell-derived exosomes: What factors may be involved? Sci China Life Sci, 65(7): 1325–1341. http://doi.org/10.1007/s11427-021-1997-2
Yuan W, Wu Y, Huang M, et al., 2022, A new frontier in temporomandibular joint osteoarthritis treatment: Exosome-based therapeutic strategy. Front Bioeng Biotechnol, 10: 1074536. http://doi.org/10.3389/fbioe.2022.1074536