Combination of 3D printing and electrospinning to develop chitin/gelatin/PVA scaffolds
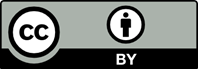
In this study, novel scaffolds based on natural polymers were developed by combining 3D printing (3DP) and electrospinning (ES) techniques. ES ink was prepared with gelatin and poly(vinyl alcohol) (PVA), while 3DP ink was prepared with gelatin and chitin. Different biopolymers were used to confer unique properties to each ink and obtain a multilayered scaffold suitable for tissue regeneration. First, gelatin is able to exhibit the characteristics needed for both inks since gelatin chains contain arginineglycine-aspartic (RGD) motifs, an important sequence in the promotion of cell adhesion, which gives gelatin an improved biological behavior in comparison to other polymers. Additionally, PVA was selected for ES ink to facilitate gelatin spinnability, and chitin was incorporated into 3DP ink as reinforcement to provide mechanical support and protection to the overall design. In this work, chitin was extracted from fruit fly pupae. The high extraction yield and purity of the chitin obtained from the fruit fly pupae confirmed that this pupa is an alternative source to produce chitin. Once the chitin was characterized, both inks were prepared and rheological analysis was carried out in order to confirm the shear thinning behavior required for additive manufacturing processes. The combination of 3DP and ES processes resulted in porous scaffolds, which were proven biocompatible, highlighting their potential for biomedical applications.
1. Omran BA, Baek K-H, 2022, Valorization of agro-industrial biowaste to green nanomaterials for wastewater treatment: Approaching green chemistry and circular economy principles. J Environ Manage, 311:114806.
2. Águila-Almanza E, Low SS, Hernández-Cocoletzi H, et al., 2021, Facile and green approach in managing sand crab carapace biowaste for obtention of high deacetylation percentage chitosan. J Environ Chem Eng, 9:105229.
3. Ren B, Weitzel KA, Duan X, et al., 2022, A comprehensive review on algae removal and control by coagulation-based processes: mechanism, material, and application. Sep Purif Technol, 293:121106.
4. Tuão Gava CA, da Silva JC, Simões WL, et al., 2021, Impact of soil texture on conidia movement and residual effect of entomopathogenic fungi applied through irrigation to control fruit-fly pupae in mango orchards. Biol Control, 163:104559.
5. Wappner P, Kramer KJ, Hopkins TL, et al., 1995, White pupa: a Ceratitis capitata mutant lacking catecholamines for tanning the puparium. Insect Biochem Mol Biol, 25:365.
6. Tsurkan MV, Voronkina A, Khrunyk Y, et al., 2021, Progress in chitin analytics. Carbohydr Polym, 252:117204.
7. Chen C, Deng S, Yang Y, et al., 2018, Highly transparent chitin nanofiber/gelatin nanocomposite with enhanced mechanical properties. Cellulose, 25:5063.
8. Zheng Y, Li X, Huang Y, et al., 2022, Two colorimetric films based on chitin whiskers and sodium alginate/gelatin incorporated with anthocyanins for monitoring food freshness. Food Hydrocolloid, 127:107517.
9. Orooji Y, Han N, Nezafat Z, et al., 2022, Valorisation of nuts biowaste: Prospects in sustainable bio(nano)catalysts and environmental applications. J Clean Prod, 347:131220.
10. Álvarez-Castillo E, Felix M, Bengoechea C, et al., 2021, Proteins from Agri-Food industrial biowastes or co-products and Their applications as green materials. Foods, 10:981.
11. Rodriguez Colon R, Nayak VV, Parente PEL, et al., 2022, The presence of 3D printing in orthopedics: A clinical and material review. J Orthop Res, 40:1–13.
https://doi.org/10.1002/jor.25388
12. Smith ML, Jones JFX, 2018, Dual-extrusion 3D printing of anatomical models for education. Anat Sci Educ, 11:65.
13. Dey D, Srinivas D, Panda B, et al., 2022, Use of industrial waste materials for 3D printing of sustainable concrete: A review. J Clean Prod, 340:130749.
14. Mustapha KB, Metwalli KM, 2021, A review of fused deposition modelling for 3D printing of smart polymeric materials and composites. Eur Polym J, 156:110591.
15. Dong Q, Zhang M, Zhou X, et al., 2021, 3D-printed Mg-incorporated PCL-based scaffolds: A promising approach for bone healing. Mater Sci Eng C, 129:112372.
16. Xie Z, Gao M, Lobo AO, et al., 2020, 3D bioprinting in tissue engineering for medical applications: The classic and the hybrid. Polymers, 12:1717. https://doi.org/10.3390/polym12081717
17. Nam S, Mooney D, 2021, Polymeric Tissue Adhesives. Chem Rev, 121:11336.
18. Afewerki S, Sheikhi A, Kannan S, et al., 2019, Gelatin-polysaccharide composite scaffolds for 3D cell culture and tissue engineering: Towards natural therapeutics. Bioeng Transl Med, 4:96.
19. Nhu CN, Thi NV, Hoang NN, et al., 2021, Characterization of gelatin and PVA nanofibers fabricated using electrospinning process. in Advances in Engineering Research and application, Sattler KU, Nguyen DC, Vu NP, Long BT, Puta H (Eds.), Springer International Publishing, Cham, 216–222.
20. Li T, Sun M, Wu S, 2022, State-of-the-Art review of electrospun gelatin-based nanofiber dressings for wound healing applications. Nanomaterials, 12:784.
21. Thangprasert A, Tansakul C, Thuaksubun N, et al., 2019, Mimicked hybrid hydrogel based on gelatin/PVA for tissue engineering in subchondral bone interface for osteoarthritis surgery. Mater Design, 183:108113.
22. Abel SB, Ballarin FM, Abraham GA, 2020, Combination of electrospinning with other techniques for the fabrication of 3D polymeric and composite nanofibrous scaffolds with improved cellular interactions. Nanotechnology, 31:172002.
23. Bidgoli MR, Alemzadeh I, Tamjid E, et al., 2019, Fabrication of hierarchically porous silk fibroin-bioactive glass composite scaffold via indirect 3D printing: Effect of particle size on physico-mechanical properties and in vitro cellular behavior. Mater Sci Eng C, 103:109688.
24. Hernandez JL, Woodrow KA, 2022, Medical applications of porous biomaterials: Features of porosity and tissue-specific implications for biocompatibility. Adv Healthc Mater, 11:2102087.
25. Ma P, Wu W, Wei Y, et al., 2021, Biomimetic gelatin/chitosan/ polyvinyl alcohol/nano-hydroxyapatite scaffolds for bone tissue engineering. Mater Design, 207:109865.
26. Sánchez-Cardona Y, Echeverri-Cuartas CE, López MEL, et al., 2021, Chitosan/Gelatin/PVA scaffolds for beta pancreatic cell culture. Polymers, 13:2372.
27. Chen Y-M, Pekdemir S, Bilican I, et al., 2021, Production of natural chitin film from pupal shell of moth: Fabrication of plasmonic surfaces for SERS-based sensing applications. Carbohydr Polym, 262:117909.
28. Kucukgulmez A, Celik M, Yanar Y, et al., 2011, Physicochemical characterization of chitosan extracted from metapenaeus stebbingi shells. Food Chem, 126:1144.
29. Pössl A, Hartzke D, Schmidts TM, et al., 2021, A targeted rheological bioink development guideline and its systematic correlation with printing behavior. Biofabrication, 13:035021.
30. Schneider CA, Rasband WS, Eliceiri KW, 2012, NIH Image to ImageJ: 25 years of image análisis. Nat Methods, 9:671.
31. International Organization for Standardization, 2009, Biological evaluation of medical devices - Part 5: Tests for in vitro cytotoxicity. ISO 10993-5:2009, https://www. iso.org/cms/render/live/en/sites/isoorg/contents/data/ standard/03/64/36406.html
32. Liao J, Huang H, 2020, Extraction of a novel fungal chitin from hericium erinaceus residue using multistep mild procedures. Int J Biol Macromol, 156:1279.
33. Antonov A, Ivanov G, Pastukhova N, et al., 2019, Production of chitin from dead hermetia illucens. IOP Conf Ser Earth Environ Sci, 315:042003.
34. Mohan K, Ganesan AR, Muralisankar T, et al., 2020, Recent insights into the extraction, characterization, and bioactivities of chitin and chitosan from insects. Trends Food Sci Technol, 105:17.
35. Joseph SM, Krishnamoorthy S, Paranthaman R, et al., 2021, A review on source-specific chemistry, functionality, and applications of chitin and chitosan. Carbohydr Polym Technol Appl, 2:100036.
36. Wei A, Fu J, Guo F, 2021, Mechanical properties of chitin polymorphs: A computational study. J Mater Sci, 56:12048.
37. Złotko K, Waśko A, Kamiński DM, et al., 2021, Isolation of Chitin from Black Soldier Fly (Hermetia illucens) and Its usage to metal sorption. Polymers, 13:818.
38. Uranga J, Etxabide A, Cabezudo S, et al., 2020, Valorization of marine-derived biowaste to develop chitin/fish gelatin products as bioactive carriers and moisture scavengers. Sci Total Environ, 706:135747.
39. Jantzen da Silva Lucas A, Quadro Oreste E, Leão Gouveia Costa H, et al., 2021, Extraction, physicochemical characterization, and morphological properties of chitin and chitosan from cuticles of edible insects. Food Chem, 343:128550.
40. Kaya M, Sofi K, Sargin I, et al., 2016, Changes in physicochemical properties of chitin at developmental stages (larvae, pupa and adult) of Vespa crabro (wasp). Carbohydr Polym, 145:64.
41. Dhanabalan V, Xavier KAM, Eppen S, et al., 2021, Characterization of chitin extracted from enzymatically deproteinized acetes shell residue with varying degree of hydrolysis. Carbohydr Polym, 253:117203.
42. Soetemans L, Uyttebroek M, Bastiaens L, 2020, Characteristics of chitin extracted from black soldier fly in different life stages. Int J Biol Macromol, 165:3206.
43. Wardhono EY, Pinem MP, Kustiningsih I, et al., 2021, Heterogeneous deacetylation reaction of chitin under low-frequency ultrasonic irradiation. Carbohydr Polym, 267:118180.
44. Ilyas HN, Zia KM, Rehman S, et al., 2021, Utilization of shellfish industrial waste for isolation, purification, and characterizations of Chitin from Crustacean’s sources in Pakistan. J Polym Environ, 29:2337.
45. Agulló E, Mato R, Peniche C, et al., 2004, Quitina y quitosano: obtención, caracterización y aplicaciones, Pastor de Abram A (Eds.), Pontifica universidad católica del peru, fondo editorial, 105-154.
46. Sabab A, Liu S, Javadiyan S, et al., 2021, The effect of chemical and structural modifiers on the haemostatic process and cytotoxicity of the beta-chitin patch. Sci Rep, 11:18577.
47. Aranaz I, Mengíbar M, Harris R, et al., 2009, Functional characterization of chitin and chitosan. Curr Chem Biol, 3:203.
48. Fernández-Marín R, Hernández-Ramos F, Salaberria AM, et al., 2021, Eco-friendly isolation and characterization of nanochitin from different origins by microwave irradiation: Optimization using response surface methodology. Int J Biol Macromol, 186:218.
49. Tan YN, Chin YL, Chen WN, 2021, Comparison of sustainable lipid and protein removal methods for the isolation of insect chitin from Black Soldier Fly exoskeleton. ACS Food Sci Technol, 1:698.
50. Ssekatawa K, Byarugaba DK, Wampande EM, et al., 2021, Isolation and characterization of chitosan from ugandan edible mushrooms, Nile perch scales and banana weevils for biomedical applications. Sci Rep, 11:4116.
51. Lee SC, Gillispie G, Prim P, et al., 2020, Physical and chemical factors influencing the printability of hydrogel-based extrusion bioinks. Chem Rev, 120:10834.
52. Tian S, Zhao H, Lewinski N, 2021, Key parameters and applications of extrusion-based bioprinting. Bioprinting, 23:e00156.
53. Ahmady A, Abu Samah NH, 2021, A review: Gelatine as a bioadhesive material for medical and pharmaceutical applications. Int J Pharm, 608:121037.
54. Spence Leung SH, Robinson JR, 1987, The contribution of anionic polymer structural features to mucoadhesion. J Control Release, 5:223.
55. Bassi da Silva J, de S. Ferreira SB, Reis AV, et al., 2018, Assessing mucoadhesion in polymer gels: The effect of method type and instrument variables. Polymers, 10:254.
56. Jovanović M, Tomić N, Cvijić S, et al., 2021, Mucoadhesive gelatin buccal films with propranolol hydrochloride: Evaluation of mechanical. Pharmaceutics, 13:273.
57. Sakaguchi K, Tobe Y, Yang J, et al., 2021, Bioengineering of a scaffold-less three-dimensional tissue using net mould, Biofabrication. Biofabrication, 13:045019.
58. Kuo C-C, Qin H, Cheng Y, et al., 2021, An integrated manufacturing strategy to fabricate delivery system using gelatin/alginate hybrid hydrogels: 3D printing and freeze-drying. Food Hydrocolloid, 111:106262.
59. Zhang C, Wang C-S, Therriault D, et al., 2022, Development of aqueous protein/polysaccharide mixture-based inks for 3D printing towards food applications. Food Hydrocolloid, 107742.
60. Breene WM, 1975, Application of texture profile analysis to instrumental food texture evaluation. J Texture Stud, 6:53.