Flow performance of porous implants with different geometry: Line, surface, and volume structures
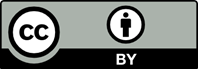
Additive manufacturing has enormous advantage of personalized adaptation. Particularly, porous implants have been widely used in clinical practice. Porous implant has the advantages and abilities to promote tissue growth and mass transfer, which are closely related to pore morphology. The purpose of this study is to investigate the effects of three porous structures, i.e., line structure, surface structure, and volume structure, on the flow properties of implants at different porosity. Therefore, a unit cell was selected from each type of structure (oct truss [OT], gyroid [G], and schwarz p [P]) as a typical cell, where OT is a line structure, G is a surface structure, and P is a volume structure. The scaffolds with different porosity of these cells were customized, and the shape parameters were measured and calculated. Then, the flow performance of three different scaffolds in Dulbecco’s modified Eagle’s medium was simulated by using computational fluid dynamics, and the flow velocity, permeability, and wall shear stress, which can reflect their biological properties, were calculated and compared. The results showed that the pore shape and porosity of porous implants greatly affected the physical parameters and performance of structures. These findings will contribute to unit cell selection and future performance optimization of porous implants.
1. Anselme K, 2000, Osteoblast adhesion on biomaterials. Biomaterials, 21:667–681. https://doi.org/10.1016/S0142-9612(99)00242-2
2. Peltola SM, Melchels FPW, Grijpma DW, et al., 2008, A review of rapid prototyping techniques for tissue engineering purposes. Ann Med, 40:268–280. https://doi.org/10.1080/07853890701881788
3. Bobbert FSL, Lietaert K, Eftekhari AA, et al., 2017, Additively manufactured metallic porous biomaterials based on minimal surfaces: A unique combination of topological, mechanical, and mass transport properties. Acta Biomater, 53:572–584. https://doi.org/10.1016/j.actbio.2017.02.024
4. Kumar A, Mandal S, Barui S, et al., 2016, Low temperature additive manufacturing of three dimensional scaffolds for bone-tissue engineering applications: Processing related challenges and property assessment. Mater Sci Eng R Rep, 103:1–39. https://doi.org/10.1016/j.mser.2016.01.001
5. Kelly CN, Miller AT, Hollister SJ, et al., 2018, Design and structure-function characterization of 3D printed synthetic porous biomaterials for tissue engineering. Adv Healthc Mater, 7:1701095. https://doi.org/10.1002/adhm.201701095
6. Školáková A, Körberová J, Málek J, et al., 2020, Microstructural, mechanical, corrosion and cytotoxicity characterization of porous Ti-Si alloys with pore-forming agent. Materials, 13:5607. https://doi.org/10.3390/ma13245607
7. Szklanny AA, Debbi L, Merdler U, et al., 2020, High‐throughput scaffold system for studying the effect of local geometry and topology on the development and orientation of sprouting blood vessels. Adv Funct Mater, 30:1901335. https://doi.org/10.1002/adfm.201901335
8. Rodríguez-Montaño ÓL, Cortés-Rodríguez CJ, Uva AE, et al., 2018, Comparison of the mechanobiological performance of bone tissue scaffolds based on different unit cell geometries. J Mech Behav Biomed Mater, 83:28–45. https://doi.org/10.1016/j.jmbbm.2018.04.008
9. Castro APG, Santos J, Pires T, et al., 2020, Micromechanical behavior of TPMS scaffolds for bone tissue engineering. Macromol Mater Eng, 305:2000487. https://doi.org/10.1002/mame.202000487
10. Bružauskaitė I, Bironaitė D, Bagdonas E, et al., 2016, Scaffolds and cells for tissue regeneration: Different scaffold pore sizes—Different cell effects. Cytotechnology, 68: 355–369. https://doi.org/10.1007/s10616-015-9895-4
11. Zhao F, Vaughan TJ, McNamara LM, 2016, Quantification of fluid shear stress in bone tissue engineering scaffolds with spherical and cubical pore architectures. Biomech Model Mechanobiol, 15:561–577. https://doi.org/10.1007/s10237-015-0710-0
12. Ali D, Ozalp M, Blanquer SBG, et al., 2020, Permeability and fluid flow-induced wall shear stress in bone scaffolds with TPMS and lattice architectures: A CFD analysis. Eur J Mech B Fluids, 79:376–385. https://doi.org/10.1016/j.euromechflu.2019.09.015
13. Zhao F, Rietbergen B, Ito K, et al., 2020, Fluid flow‐induced cell stimulation in bone tissue engineering changes due to interstitial tissue formation in vitro. Int J Numer Meth Biomed Engng, 36:e3342. https://doi.org/10.1002/cnm.3342
14. Zhao F, Lacroix D, Ito K, et al., 2020, Changes in scaffold porosity during bone tissue engineering in perfusion bioreactors considerably affect cellular mechanical stimulation for mineralization. Bone Rep, 12:100265. https://doi.org/10.1016/j.bonr.2020.100265
15. Cartmell SH, Porter BD, García AJ, et al., 2003, Effects of medium perfusion rate on cell-seeded three-dimensional bone constructs in vitro. Tissue Eng, 9:1197–1203. https://doi.org/10.1089/10763270360728107
16. Castro APG, Lacroix D, 2018, Micromechanical study of the load transfer in a polycaprolactone–collagen hybrid scaffold when subjected to unconfined and confined compression. Biomech Model Mechanobiol, 17:531–541. https://doi.org/10.1007/s10237-017-0976-5
17. Castro APG, Pires T, Santos JE, et al., 2019, Permeability versus design in TPMS scaffolds. Materials, 12:1313. https://doi.org/10.3390/ma12081313
18. Zhao S, Hou WT, Xu QS, et al., 2018, Ti-6Al-4V lattice structures fabricated by electron beam melting for biomedical applications. In Titanium in Medical and Dental Applications, Elsevier, 277–301. https://doi.org/10.1016/B978-0-12-812456-7.00013-5
19. Li J, Chen D, Luan H, et al., 2017, Mechanical performance of porous implant with different unit cells. J Mech Med Biol, 17:1750101. https://doi.org/10.1142/S0219519417501019
20. Ma S, Song K, Lan J, et al., 2020, Biological and mechanical property analysis for designed heterogeneous porous scaffolds based on the refined TPMS. J Mech Behav Biomed Mater, 107:103727. https://doi.org/10.1016/j.jmbbm.2020.103727
21. Shi J, Zhu L, Li L, et al., 2018, A TPMS-based method for modeling porous scaffolds for bionic bone tissue engineering. Sci Rep, 8:7395. https://doi.org/10.1038/s41598-018-25750-9
22. Santos J, Pires T, Gouveia BP, et al., 2020, On the permeability of TPMS scaffolds. J Mech Behav Biomed Mater, 110:103932. https://doi.org/10.1016/j.jmbbm.2020.103932
23. Ahmadi SM, Hedayati R, Li Y, et al., 2018, Fatigue performance of additively manufactured meta-biomaterials: The effects of topology and material type. Acta Biomater, 65:292–304. https://doi.org/10.1016/j.actbio.2017.11.014
24. Wu J, Sigmund O, Groen JP, 2021, Topology optimization of multi-scale structures: A review. Struct Multidisc Optim, 63:1455–1480. https://doi.org/10.1007/s00158-021-02881-8
25. Ali D, Sen S, 2018, Computational fluid dynamics study of the effects of surface roughness on permeability and fluid flow-induced wall shear stress in scaffolds. Ann Biomed Eng, 46:2023–2035. https://doi.org/10.1007/s10439-018-2101-z
26. Pires T, Santos J, Ruben RB, et al., 2021, Numerical-experimental analysis of the permeability-porosity relationship in triply periodic minimal surfaces scaffolds. J Biomech, 117:110263. https://doi.org/10.1016/j.jbiomech.2021.110263
27. Ali D, Sen S, 2018, Permeability and fluid flow-induced wall shear stress of bone tissue scaffolds: Computational fluid dynamic analysis using Newtonian and non-Newtonian blood flow models. Comput Biol Med, 99:201–208. https://doi.org/10.1016/j.compbiomed.2018.06.017
28. Zhao F, Melke J, Ito K, et al., 2019, A multiscale computational fluid dynamics approach to simulate the micro-fluidic environment within a tissue engineering scaffold with highly irregular pore geometry. Biomech Model Mechanobiol, 18:1965–1977. https://doi.org/10.1007/s10237-019-01188-4
29. Poon C, 2022, Measuring the density and viscosity of culture media for optimized computational fluid dynamics analysis of in vitro devices. J Mech Behav Biomed Mater, 126:105024. https://doi.org/10.1016/j.jmbbm.2021.105024
30. Baroud G, Falk R, Crookshank M, et al., 2004, Experimental and theoretical investigation of directional permeability of human vertebral cancellous bone for cement infiltration. J Biomech, 37:189–196. https://doi.org/10.1016/S0021-9290(03)00246-X
31. Nauman EA, Fong KE, Keaveny TM, 1999, Dependence of intertrabecular permeability on flow direction and anatomic site. Ann Biomed Eng, 27:517–524. https://doi.org/10.1114/1.195
32. Kohles SS, Roberts JB, Upton ML, et al., 2001, Direct perfusion measurements of cancellous bone anisotropic permeability. J Biomech, 34:1197–1202. https://doi.org/10.1016/S0021-9290(01)00082-3
33. Sander EA, Shimko DA, Dee KC, et al., 2003, Examination of continuum and micro-structural properties of human vertebral cancellous bone using combined cellular solid models. Biomech Model Mechanobiol, 2:97–107. https://doi.org/10.1007/s10237-003-0031-6
34. Syahrom A, Abdul Kadir MR, Abdullah J, et al., 2013, Permeability studies of artificial and natural cancellous bone structures. Med Eng Phys, 35:792–799. https://doi.org/10.1016/j.medengphy.2012.08.011
35. Chappard D, Kün-Darbois J-D, Guillaume B, 2020, Computational fluid dynamics simulation from microCT stacks of commercial biomaterials usable for bone grafting. Micron, 133:102861. https://doi.org/10.1016/j.micron.2020.102861
36. Ouyang P, Dong H, He X, et al., 2019, Hydromechanical mechanism behind the effect of pore size of porous titanium scaffolds on osteoblast response and bone ingrowth. Mater Design, 183:108151. https://doi.org/10.1016/j.matdes.2019.108151
37. Singh SP, Shukla M, Srivastava RK, 2018, Lattice modeling and CFD simulation for prediction of permeability in porous scaffolds. Mater Today Proc, 5:18879–18886. https://doi.org/10.1016/j.matpr.2018.06.236
38. Du Y, Liang H, Xie D, et al., 2019, Finite element analysis of mechanical behavior, permeability of irregular porous scaffolds and lattice-based porous scaffolds. Mater Res Express, 6:105407. https://doi.org/10.1088/2053-1591/ab3ac1
39. Yu G, Li Z, Li S, et al., 2020, The select of internal architecture for porous Ti alloy scaffold: A compromise between mechanical properties and permeability. Mater Design, 192:108754. https://doi.org/10.1016/j.matdes.2020.108754
40. Timercan A, Sheremetyev V, Brailovski V, 2021, Mechanical properties and fluid permeability of gyroid and diamond lattice structures for intervertebral devices: Functional requirements and comparative analysis. Sci Technol Adv Mater, 22:285–300. https://doi.org/10.1080/14686996.2021.1907222
41. Foroughi AH, Razavi MJ, 2022, Multi-objective shape optimization of bone scaffolds: Enhancement of mechanical properties and permeability. Acta Biomater, 146:317–340. https://doi.org/10.1016/j.actbio.2022.04.051
42. Foroughi AH, Razavi MJ, 2022, Shape optimization of orthopedic porous scaffolds to enhance mechanical performance. J Mech Behav Biomed Mater, 128:105098. https://doi.org/10.1016/j.jmbbm.2022.105098