Evaluation of mechanical and biological properties of akermanite/poly-ether-etherketone composite fabricated by hightemperature laser powder bed fusion
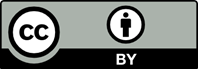
High-temperature laser bed powder fusion (HT-LPBF) technology is an ideal method for processing poly-ether-ether-ketone (PEEK) implants with personalized bionic structures, but the biological inertia of PEEK limits its medical applications. In this study, we evaluated the mechanical and biological properties of a novel akermanite (AKM)/PEEK composite for HT-LPBF. The results showed that tiny AKM particles are evenly attached to the surface of the PEEK particle. The delayed peak crystallization temperature and stable sintering window ensure the processing feasibility of the AKM/PEEK composites. The tensile strength and Young’s modulus are in the range of 30.83–98.73 MPa and 2.27–3.71 GPa, respectively, which can match the properties of cancellous bones and meet their implanting requirement. The CCK-8 experiments demonstrated the biocompatibility of the composites and the good proliferation of bone marrow stromal cells. The dense hydroxyapatite network layer and petal-like hydroxyapatite demonstrates biological activity, indicating that the composite has a good potential in the orthopedics fields.
1. Chen P, Wang H, Su J, et al., 2022, Recent advances on high-performance polyaryletherketone materials for additive manufacturing. Adv Mater, 34, 2200750:1–26.
2. Chen J, Liu X, Tian Y, et al., 2022, 3D-printed anisotropic polymer materials for functional applications. Adv Mater, 34(5):2102877.
3. Wu H, Wang O, Tian Y, et al., 2021, Selective laser sintering-based 4D printing of magnetism-responsive grippers. ACS Appl Mater Interfaces, 13(11):12679–12688.
4. Wu H, Zhang X, Ma Z, et al., 2020, A material combination concept to realize 4D printed products with newly emerging property/functionality. Adv Sci, 7(9):1903208.
5. Feng P, Wu P, Gao C, et al., 2018, A multimaterial scaffold with tunable properties: Toward bone tissue repair, Adv Sci, 5(6):1700817.
6. Berretta S, Evans K, Ghita O, 2018, Additive manufacture of PEEK cranial implants: Manufacturing considerations versus accuracy and mechanical performance. Mater Des, 139:141–152.
7. Wu H, Wang Q, Wu Z, et al., 2022, Multi-material additively manufactured magnetoelectric architectures with a structure-dependent mechanical-to-electrical conversion capability. Small Methods, 6(12):e2201127.
8. Chen P, Su J, Wang H, et al., 2022, Mechanical properties and microstructure characteristics of lattice-surfaced PEEK cage fabricated by high-temperature laser powder bed fusion. J Mater Sci Technol, 125:105–117.
9. Chen B, Yazdani B, Benedetti L, et al., 2019, Fabrication of nanocomposite powders with a core-shell structure. Compos Sci Technol, 170:116–127.
10. Shirazi SF, Gharehkhani S, Mehrali M, et al., 2015, A review on powder-based additive manufacturing for tissue engineering: Selective laser sintering and inkjet 3D printing. Sci Technol Adv Mater, 16(3):033502.
11. Wang H, Chen P, Wu H, et al., 2022, Comparative evaluation of printability and compression properties of poly-ether-ether-ketone triply periodic minimal surface scaffolds fabricated by laser powder bed fusion. Addit Manuf, 57:102961.
12. Yang L, Yan C, Han C, et al., 2018, Mechanical response of a triply periodic minimal surface cellular structures manufactured by selective laser melting. Int J Mech Sci, 148:149–157.
13. Zhang C, Zheng H, Yang L, et al., 2022, Mechanical responses of sheet-based gyroid-type triply periodic minimal surface lattice structures fabricated using selective laser melting. Mater Des, 214:110407.
14. Haleem A, Javaid M, 2019, Polyether ether ketone (PEEK) and its 3D printed implants applications in medical field: An overview. Clin Epidemiol Global Health, 7(4):571–577.
15. Oladapo BI, Zahedi SA, Ismail SO, et al., 2021, 3D printing of PEEK and its composite to increase biointerfaces as a biomedical material: A review. Colloids Surf B Biointerfaces, 203:111726.
16. Chen B, Berretta S, Evans K, et al., 2018, A primary study into graphene/polyether ether ketone (PEEK) nanocomposite for laser sintering. Appl Surf Sci, 428:1018–1028.
17. Zhang S, Feng Z, Hu Y, et al., 2022, Endowing polyetheretherketone implants with osseointegration Properties: In situ construction of patterned nanorod arrays. Small, 18(5):2105589.
18. Yuan X, Ouyang L, Luo Y, et al., 2019, Multifunctional sulfonated polyetheretherketone coating with beta-defensin-14 for yielding durable and broad-spectrum antibacterial activity and osseointegration. Acta Biomater, 86:323–337.
19. Tan LJ, Zhu W, Zhou K, 2020, Recent progress on polymer materials for additive manufacturing. Adv Funct Mater, 30(43):2003062.
20. Najeeb S, Zafar MS, Khurshid Z, et al., 2016, Applications of polyetheretherketone (PEEK) in oral implantology and prosthodontics. J Prosthodont Res, 60(1):12–19.
21. Brizuela A, Herrero-Climent M, Rios-Carrasco E, et al., 2019, Influence of the elastic modulus on the osseointegration of dental implants. Materials, 12(6):980.
22. Basgul C, Yu T, MacDonald DW, et al., 2020, Does annealing improve the interlayer adhesion and structural integrity of FFF 3D printed PEEK lumbar spinal cages? J Mech Behav Biomed Mater, 102:103455.
23. Velasco-Hogan A, Xu J, Meyers MA, 2018, Additive manufacturing as a method to design and optimize bioinspired structures. Adv Mater, 30(52):1800940.
24. Chen B, Wang Y, Berretta S, et al., 2017, Poly aryl ether ketones (PAEKs) and carbon-reinforced PAEK powders for laser sintering. J Mater Sci, 52(10):6004–6019.
25. Berretta S, Evans KE, Ghita O, 2015, Processability of PEEK, a new polymer for high temperature laser sintering (HT-LS). Eur Polym J, 68(Suppl C):243–266.
26. Berretta S, Evans KE, Ghita OR, 2016, Predicting processing parameters in high temperature laser sintering (HT-LS) from powder properties. Mater Des, 105:301–314.
27. Chen P, Cai H, Li Z, et al., 2020, Crystallization kinetics of polyetheretherketone during high temperature-selective laser sintering. Addit Manuf, 36:101615.
28. Torstrick FB, Lin ASP, Potter D, et al., 2018, Porous PEEK improves the bone-implant interface compared to plasma-sprayed titanium coating on PEEK. Biomaterials 185:106–116.
29. Almasi D, Iqbal N, Sadeghi M, et al., 2016, Preparation methods for improving PEEK’s bioactivity for orthopedic and dental application: A review. Int J Biomater, 2016:8202653.
30. Ma R, Tang T, 2014, Current strategies to improve the bioactivity of PEEK. Int J Mol Sci, 15(4):5426–5445.
31. Vaezi M, Black C, Gibbs DMR, et al., 2016, Characterization of new PEEK/HA composites with 3D HA network fabricated by extrusion freeforming. Molecules 21(6):687.
32. Von Wilmonsky C, Lutz R, Meisel U, et al., 2009, In vivo evaluation of ß-TCP containing 3D laser sintered poly(ether ether ketone) composites in pigs. J Bioact Compat Polym, 24(2):169–184.
33. Ma R, Yu Z, Tang S, et al., 2016, Osseointegration of nanohydroxyapatite- or nano-calcium silicate-incorporated polyetheretherketone bioactive composites in vivo. Int J Nanomed, 11:6023–6033.
34. Wang H, Chen P, Shu Z, et al., 2023, Laser powder bed fusion of poly-ether-ether-ketone/bioactive glass composites: Processability, mechanical properties, and bioactivity. Compos Sci Technol, 231:109805.
35. Yuan S, Shen F, Chua CK, et al., 2019, Polymeric composites for powder-based additive manufacturing: Materials and applications. Progr Polym Sci, 91:141–168.
36. Mohammadi H, Baba Ismail YM, Bin Shariff KA, et al., 2018, Synthesis and characterization of Akermanite by mechanical milling and subsequent heat treatment. J Phys Conf Series, 1082:012021.
37. Xu S, Lin K, Wang Z, et al., 2008, Reconstruction of calvarial defect of rabbits using porous calcium silicate bioactive ceramics. Biomaterials, 29(17):2588–2596.
38. Huang Y, Jin X, Zhang X, et al., 2009, In vitro and in vivo evaluation of akermanite bioceramics for bone regeneration. Biomaterials, 30(28):5041–5048.
39. Wu C, Chang J, Zhai W, et al., 2006, Porous akermanite scaffolds for bone tissue engineering: Preparation, characterization, and in vitro studies. J Biomed Mater Res Part B Appl Biomater, 78B(1):47–55.
40. Duman Ş, Bulut B, 2021, Effect of akermanite powders on mechanical properties and bioactivity of chitosan-based scaffolds produced by 3D-bioprinting. Ceram Int, 47(10):13912–13921.
41. Zhai W, Lu H, Chen L, et al., 2012, Silicate bioceramics induce angiogenesis during bone regeneration. Acta Biomater, 8(1):341–349.
42. Chen P, Cai H, Li Z, et al., 2018, Crystallization kinetics of polyetheretherketone during high temperature-selective laser sintering. Addit Manuf, 36:101615.
43. Chen P, Wu H, Zhu W, et al., 2018, Investigation into the processability, recyclability and crystalline structure of selective laser sintered Polyamide 6 in comparison with Polyamide 12. Polym Test, 69:366–374.
44. Chen P, Su J, Wang H, et al., 2022, Aging mechanism of polyetheretherketone powder during layer-wise infrared radiation of high-temperature laser powder bed fusion. Mater Des, 213:110348.
45. Zhu W, Yan C, Shi Y, et al., 2015, Investigation into mechanical and microstructural properties of polypropylene manufactured by selective laser sintering in comparison with injection molding counterparts. Mater Des, 82:37–45.
46. Li Y, Liu C, 2017, Nanomaterial-based bone regeneration. Nanoscale, 9(15):4862–4874.
47. Geetha M, Singh AK, Asokamani R, et al., 2009, Ti based biomaterials, the ultimate choice for orthopaedic implants—A review. Progr Mater Sci, 54(3):397–425.
48. Su J, Hua S, Chen A, et al., 2022, Three-dimensional printing of gyroid-structured composite bioceramic scaffolds with tuneable degradability. Biomater Adv, 133:112595.