Three-dimensional printing of microfiberreinforced hydrogel loaded with oxymatrine for treating spinal cord injury
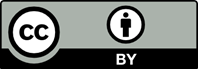
Spinal cord injury (SCI) causes severe neural tissue damage and motor/sensory dysfunction. Since the injured spinal cord tissue has limited self-regeneration ability, several strategies, including cell therapy, drug delivery, and tissue engineering scaffold implantation, have been employed to treat SCI. However, each of these strategies fails to obtain desirable outcomes due to their respective limitations. In comparison, advanced tissue engineering scaffolds with appropriate topographical features, favorable composition, and sustained drug delivery capability can be employed to recruit endogenous neural stem cells (NSCs), induce neuronal differentiation, and facilitate neuron maturation. This can lead to the regeneration of injured spinal cord tissue and the recovery of motor function. In this study, fiber bundle-reinforced spinal cord extracellular matrix hydrogel scaffolds loaded with oxymatrine (OMT) were produced through nearfield direct write electrospinning. The spinal cord extracellular matrix-based hydrogel was then coated with OMT. The physical/chemical properties and in vitro degradation behavior of the composite scaffolds were investigated. The in vitro cell culture results showed that composite scaffolds loaded with OMT promoted the differentiation of NSCs into neurons and inhibited differentiation into astrocytes. The in vivo results showed that the composite scaffolds loaded with OMT recruited NSCs from the host tissue, promoted neuronal differentiation and axon extension at the lesion site, inhibited glial scar formation at/around the lesion site, and improved the recovery of motor function in rats with SCI. To sum up, 3D-printed microfiber-reinforced spinal cord extracellular matrix hydrogel scaffolds loaded with OMT are promising biomaterials for the treatment of SCI.
1. Wyndaele M, 2006, Incidence, prevalence and epidemiology of spinal cord injury: What learns a worldwide literature survey? Spinal Cord, 44(9):523–529. https://doi.org/10.1038/sj.sc.3101893
2. Scivoletto G, Miscusi M, Forcato S, et al., 2017, The rehabilitation of spinal cord injury patients in Europe. Acta Neurochir Suppl, 124:203–210. https://doi.org/10.1007/978-3-319-39546-3_31
3. Rupp R, 2020, Spinal cord lesions. Handb Clin Neurol, 168:51–65. https://doi.org/10.1016/B978-0-444-63934-9.00006-8
4. Guo S, 2021, Spinal cord repair: From cells and tissue engineering to extracellular vesicles. Cells, 10(8):1872. https://doi.org/10.3390/cells10081872
5. Shi Z, Yuan S, Shi L, et al., 2021, Programmed cell death in spinal cord injury pathogenesis and therapy. Cell Prolif, 54(3):e12992. https://doi.org/10.1111/cpr.12992
6. Chen X, Wang Y, Zhou G, et al., 2021, The combination of nanoscaffolds and stem cell transplantation: Paving a promising road for spinal cord injury regeneration. Biomed Pharmacother, 143:112233. https://doi.org/10.1016/j.biopha.2021.112233
7. Zawadzka M, Kwasniewska A, Miazga K, et al., 2021, Perspectives in the cell-based therapies of various aspects of the spinal cord injury-associated pathologies: Lessons from the animal models. Cells, 10(11):2995. https://doi.org/10.3390/cells10112995
8. Xiao Z, Tang F, Zhao Y, et al., 2018, Significant improvement of acute complete spinal cord injury patients diagnosed by a combined criteria implanted with neuroregen scaffolds and mesenchymal stem cells. Cell Transplant, 27(6): 907–915. https://doi.org/10.1177/0963689718766279
9. Zhang Y, Al MA, Yuan Y, et al., 2021, Acute spinal cord injury: Pathophysiology and pharmacological intervention (Review). Mol Med Rep, 23(6):417. https://doi.org/10.3892/mmr.2021.12056
10. Gedde MH, Lilleberg HS, Assmus J, et al., 2019, Traumatic vs non-traumatic spinal cord injury: A comparison of primary rehabilitation outcomes and complications during hospitalization. J Spinal Cord Med, 42(6):695–701. https://doi.org/10.1080/10790268.2019.1598698
11. Haddad AF, 2021, The natural history of spinal cord injury. Neurosurg Clin N Am, 32(3):315–321. https://doi.org/10.1016/j.nec.2021.03.003
12. Fan B, Wei Z, Yao X, et al., 2018, Microenvironment imbalance of spinal cord injury. Cell Transplant, 27(6): 853–866. https://doi.org/10.1177/0963689718755778
13. Vijayavenkataraman S, Yan WC, Lu WF, et al., 2018, 3D bioprinting of tissues and organs for regenerative medicine. Adv Drug Deliv Rev, 132:296–332. https://doi.org/10.1016/j.addr.2018.07.004
14. Luo Y, 2018, 3D bioprinting of artificial tissues: Construction of biomimetic microstructures. Macromol Biosci, 18(6):e1800034. https://doi.org/10.1002/mabi.201800034
15. Junka R, 2020, Acellular polycaprolactone scaffolds laden with fibroblast/endothelial cell-derived extracellular matrix for bone regeneration. J Biomed Mater Res A, 108(2): 351–364. https://doi.org/10.1002/jbm.a.36821
16. He J, Li Z, Yu T, et al., 2020, Preparation and evaluation of acellular sheep periostea for guided bone regeneration. J Biomed Mater Res A, 108(1):19–29. https://doi.org/10.1002/jbm.a.36787
17. Gong M, Sun J, Liu G, et al., 2021, Graphene oxide-modified 3D acellular cartilage extracellular matrix scaffold for cartilage regeneration. Mater Sci Eng C Mater Biol Appl, 119:111603. https://doi.org/10.1016/j.msec.2020.111603
18. Zhao Y, Zhao X, Zhang R, et al., 2020, Cartilage extracellular matrix scaffold with kartogenin-encapsulated PLGA microspheres for cartilage regeneration. Front Bioeng Biotechnol, 8:600103. https://doi.org/10.3389/fbioe.2020.600103
19. Urciuolo A, 2018, Decellularized tissue for muscle regeneration. Int J Mol Sci, 19(8):2392. https://doi.org/10.3390/ijms19082392
20. Dhasmana A, Singh L, Roy P, et al., 2019, Silk fibroin protein modified acellular dermal matrix for tissue repairing and regeneration. Mater Sci Eng C Mater Biol Appl, 97:313–324. https://doi.org/10.1016/j.msec.2018.12.038
21. Perveen S, Rossin D, Vitale E, et al., 2021, Therapeutic acellular scaffolds for limiting left ventricular remodelling-current status and future directions. Int J Mol Sci,
22(23):13054. https://doi.org/10.3390/ijms222313054
22. Eitan Y, Sarig U, Dahan N, et al., 2010, Acellular cardiac extracellular matrix as a scaffold for tissue engineering: In vitro cell support, remodeling, and biocompatibility. Tissue Eng Part C Methods, 16(4):671–683. https://doi.org/10.1089/ten.TEC.2009.0111
23. Esmaeili PK, Mashayekhan S, Asl SG, et al., 2018, Construction of scaffolds composed of acellular cardiac extracellular matrix for myocardial tissue engineering. Biologicals, 53:10–18. https://doi.org/10.1016/j.biologicals.2018.03.005
24. Ilanlou S, Khakbiz M, Amoabediny G, et al., 2019, Preclinical studies of acellular extracellular matrices as small-caliber vascular grafts. Tissue Cell, 60:25–32. https://doi.org/10.1016/j.tice.2019.07.008
25. Horst M, Milleret V, Noetzli S, et al., 2017, Polyesterurethane and acellular matrix based hybrid biomaterial for bladder engineering. J Biomed Mater Res B Appl Biomater, 105(3):658–667. https://doi.org/10.1002/jbm.b.33591
26. Guan Y, Liu S, Liu Y, et al., 2015, Porcine kidneys as a source of ECM scaffold for kidney regeneration. Mater Sci Eng C Mater Biol Appl, 56:451–456. https://doi.org/10.1016/j.msec.2015.07.007
27. Huang D, 2016, Cogels of hyaluronic acid and acellular matrix for cultivation of adipose-derived stem cells: Potential application for vocal fold tissue engineering. Biomed Res Int, 2016:6584054. https://doi.org/10.1155/2016/6584054
28. Ge L, Arul K, Ikpeze T, et al., 2018, Traumatic and nontraumatic spinal cord injuries. World Neurosurg, 111:e142–e148. https://doi.org/10.1016/j.wneu.2017.12.008
29. Miyazaki K, 2014, Partial regeneration and reconstruction of the rat uterus through recellularization of a decellularized uterine matrix. Biomaterials, 35(31):8791–8800. https://doi.org/10.1016/j.biomaterials.2014.06.052
30. Wang F, Maeda Y, Zachar V, et al., 2018, Regeneration of the oesophageal muscle layer from oesophagus acellular matrix scaffold using adipose-derived stem cells. Biochem Biophys Res Commun, 503(1):271–277. https://doi.org/10.1016/j.bbrc.2018.06.014
31. Xu Y, Zhou J, Liu C, et al., 2021, Understanding the role of tissue-specific decellularized spinal cord matrix hydrogel for neural stem/progenitor cell microenvironment reconstruction and spinal cord injury. Biomaterials, 268:120596. https://doi.org/10.1016/j.biomaterials.2020.120596
32. Haggerty AE, 2017, Extracellular matrix components as therapeutics for spinal cord injury. Neurosci Lett, 652:50–55. https://doi.org/10.1016/j.neulet.2016.09.053
33. Liu J, Chen J, Liu B, et al., 2013, Acellular spinal cord scaffold seeded with mesenchymal stem cells promotes long-distance axon regeneration and functional recovery in spinal cord injured rats. J Neurol Sci, 325(1-2):127–136. The original reference was retracted by the journal office. https://doi.org/10.1016/j.jns.2012.11.022
34. Lan X, Zhao J, Zhang Y, et al., 2020, Oxymatrine exerts organ-and tissue-protective effects by regulating inflammation, oxidative stress, apoptosis, and fibrosis: From bench to bedside. Pharmacol Res, 151:104541. https://doi.org/10.1016/j.phrs.2019.104541
35. Deng X, Zhao F, Zhao D, et al., 2021, Oxymatrine promotes hypertrophic scar repair through reduced human scar fibroblast viability, collagen and induced apoptosis via autophagy inhibition. Int Wound J, 19(5):1221–1231. https://doi.org/10.1111/iwj.13717
36. Guan B, Chen R, Zhong M, et al., 2020, Protective effect of oxymatrine against acute spinal cord injury in rats via modulating oxidative stress, inflammation and apoptosis. Metab Brain Dis, 35(1):149–157. https://doi.org/10.1007/s11011-019-00528-8
37. Tanabe N, Kuboyama T, Kazuma K, et al., 2015, The extract of roots of Sophora flavescens enhances the recovery of motor function by axonal growth in mice with a spinal cord injury. Front Pharmacol, 6:326. https://doi.org/10.3389/fphar.2015.00326
38. Wang Q, Zhang H, Xu H, et al., 2018, Novel multi-drug delivery hydrogel using scar-homing liposomes improves spinal cord injury repair. Theranostics, 8(16):4429–4446. https://doi.org/10.7150/thno.26717
39. Fuhrmann T, 2017, Combinatorial therapies after spinal cord injury: How can biomaterials help? Adv Healthc Mater, 6(10):1601130. https://doi.org/10.1002/adhm.201601130
40. Usmani S, Franceschi Biagioni A, Medelin M, et al., 2020, Functional rewiring across spinal injuries via biomimetic nanofiber scaffolds. Proc Natl Acad Sci, 117(41):25212– 25218. https://doi.org/10.1073/pnas.2005708117
41. Kourgiantaki A, Tzeranis DS, Karali K, et al., 2020, Neural stem cell delivery via porous collagen scaffolds promotes neuronal differentiation and locomotion recovery in spinal cord injury. NPJ Regen Med, 5(1):12. https://doi.org/10.1038/s41536-020-0097-0
42. Sever Bahcekapili M, Yilmaz C, Demirel A, et al., 2021, Neuroactive peptide nanofibers for regeneration of spinal cord after injury. Macromol Biosci, 21(1):2000234. https://doi.org/10.1002/mabi.202000234
43. Sajadi E, Aliaghaei A, Farahni RM, et al., 2021, Tissue plasminogen activator loaded PCL nanofibrous scaffold promoted nerve regeneration after sciatic nerve transection in male rats. Neurotox Res, 39(2):413–428. https://doi.org/10.1007/s12640-020-00276-z
44. Babaloo H, Ebrahimi Barough S, Derakhshan MA, et al., 2018, PCL/gelatin nanofibrous scaffolds with human endometrial stem cells/schwann cells facilitate axon regeneration in spinal cord injury. J Cell Physiol, 234(7):11060–11069. https://doi.org/10.1002/jcp.27936
45. Rahimi-Sherbaf F, Nadri S, Rahmani A, et al., 2020, Placenta mesenchymal stem cells differentiation toward neuronal-like cells on nanofibrous scaffold. BioImpacts, 10(2):117–122. https://doi.org/10.34172/bi.2020.14
46. Tukmachev D, Forostyak S, Koci Z, et al., 2016, Injectable extracellular matrix hydrogels as scaffolds for spinal cord injury repair. Tissue Eng Part A, 22(3-4):306–317. https://doi.org/10.1089/ten.TEA.2015.0422
47. Ozudogru E, Isik M, Eylem CC, et al., 2021, Decellularized spinal cord meninges extracellular matrix hydrogel that supports neurogenic differentiation and vascular structure formation. J Tissue Eng Regen Med, 15(11):948–963. https://doi.org/10.1002/term.3240
48. Zhang S, Wang XJ, Li WS, et al., 2018, Polycaprolactone/ polysialic acid hybrid, multifunctional nanofiber scaffolds for treatment of spinal cord injury. Acta Biomater, 77:15–27. https://doi.org/10.1016/j.actbio.2018.06.038
49. Wang P, Wang H, Ma K, et al., 2020, Novel cytokine-loaded PCL-PEG scaffold composites for spinal cord injury repair. RSC Adv, 10(11):6306–6314. https://doi.org/10.1039/c9ra10385f
50. Xing H, Ren X, Yin H, et al., 2019, Construction of a NT-3 sustained-release system cross-linked with an acellular spinal cord scaffold and its effects on differentiation of cultured bone marrow mesenchymal stem cells. Mater Sci Eng C, 104:109902. https://doi.org/10.1016/j.msec.2019.109902
51. Yang Y, Fan Y, Zhang H, et al., 2021, Small molecules combined with collagen hydrogel direct neurogenesis and migration of neural stem cells after spinal cord injury. Biomaterials, 269:120479. https://doi.org/10.1016/j.biomaterials.2020.120479
52. Li X, Fan C, Xiao Z, et al., 2018, A collagen microchannel scaffold carrying paclitaxel-liposomes induces neuronal differentiation of neural stem cells through Wnt/beta-catenin signaling for spinal cord injury repair. Biomaterials, 183:114–127. https://doi.org/10.1016/j.biomaterials.2018.08.037
53. Okada S, Hara M, Kobayakawa K, et al., 2018, Astrocyte reactivity and astrogliosis after spinal cord injury. Neurosci Res, 126:39–43. https://doi.org/10.1016/j.neures.2017.10.004
54. Bai Y, Lai B, Han W, et al., 2021, Decellularized optic nerve functional scaffold transplant facilitates directional axon regeneration and remyelination in the injured white matter of the rat spinal cord. Neural Regen Res, 16(11):2276. https://doi.org/10.4103/1673-5374.310696
55. Sadik ME, Ozturk AK, Albayar A, et al., 2020, A strategy toward bridging a complete spinal cord lesion using stretch-grown axons. Tissue Eng Part A, 26(11-12):623–635. https://doi.org/10.1089/ten.TEA.2019.0230
56. Lan X, Zhao J, Zhang Y, et al., 2020, Oxymatrine exerts organ-and tissue-protective effects by regulating inflammation, oxidative stress, apoptosis, and fibrosis: From bench to bedside. Pharmacol Res, 151:104541. https://doi.org/10.1016/j.phrs.2019.104541
57. Nathan FM, Li S, 2017, Environmental cues determine the fate of astrocytes after spinal cord injury. Neural Regen Res, 12(12):1964–1970. https://doi.org/10.4103/1673-5374.221144
58. Gomes FC, Sousa VO, Romao L, 2005, Emerging roles for TGF-beta1 in nervous system development. Int J Dev Neurosci, 23(5):413–424. https://doi.org/10.1016/j.ijdevneu.2005.04.001
59. Liu L, Lu W, Ma Z, et al., 2012, Oxymatrine attenuates bleomycin-induced pulmonary fibrosis in mice via the inhibition of inducible nitric oxide synthase expression and the TGF-beta/Smad signaling pathway. Int J Mol Med, 29(5):815–822. https://doi.org/10.3892/ijmm.2012.923
60. Zhao HW, Zhang ZF, Chai X, et al., 2016, Oxymatrine attenuates CCl4-induced hepatic fibrosis via modulation of TLR4-dependent inflammatory and TGF-beta1 signaling pathways. Int Immunopharmacol, 36:249–255. https://doi.org/10.1016/j.intimp.2016.04.040