Extrusion Printed Silk Fibroin Scaffolds with Post-mineralized Calcium Phosphate as a Bone Structural Material
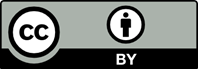
Artificial bone materials are of high demand due to the frequent occurrence of bone damage from trauma, disease, and ageing. Three-dimensional (3D) printing can tailor-make structures and implants based on biomaterial inks, rendering personalized bone medicine possible. Herein, we extrusion-printed 3D silk fibroin (SF) scaffolds using mixed inks from SF and sodium alginate (SA), and post-mineralized various calcium phosphates to make hybrid SF scaffolds. The effects of printing conditions and mineralization conditions on the mechanical properties of SF scaffolds were investigated. The SF scaffolds from ~10 wt% SF ink exhibited a compressive modulus of 240 kPa, which was elevated to ~1600 kPa after mineralization, showing a significant reinforcement effect. Importantly, the mineralized SF 3D scaffolds exhibited excellent MC3T3-E1 cell viability and promoted osteogenesis. The work demonstrates a convenient strategy to fabricate SF-based hybrid 3D scaffolds with bone-mimetic components and desirable mechanical properties for bone tissue engineering.
1. Wu CY, Lee HS, Tsai CF, et al., 2022, Secular Trends in the Incidence of Fracture Hospitalization between 2000 and 2015 among the Middle-aged and Elderly Persons in Taiwan: A Nationwide Register-based Cohort Study. Bone, 154:116250. https://doi.org/10.1016/j.bone.2021.116250
2. Behzadi S, Luther GA, Harris MB, et al., 2017, Nanomedicine for Safe Healing of Bone Trauma: Opportunities and Challenges. Biomaterials, 146:168–82. https://doi.org/10.1016/j.biomaterials.2017.09.005
3. Bharadwaz A, Jayasuriya AC, 2020, Recent Trends in the Application of Widely Used Natural and Synthetic Polymer Nanocomposites in Bone Tissue Regeneration. Mater Sci Eng C Mater Biol Appl, 110:110698. https://doi.org/10.1016/j.msec.2020.110698
4. Maia FR, Bastos AR, Oliveira JM, et al., 2022, Recent Approaches towards Bone Tissue Engineering. Bone, 154:116256. https://doi.org/10.1016/j.bone.2021.116256
5. Bose S, Ke D, Sahasrabudhe H, et al., 2018, Additive manufacturing of biomaterials. Prog Mater Sci, 93:45–111. https://doi.org/10.1016/j.pmatsci.2017.08.003
6. Yang Y, Wang G, Liang H, et al., 2019, Additive Manufacturing of Bone Scaffolds. Int J Bioprint, 5:148. https://doi.org/10.18063/IJB.v5i1.148
7. Sears NA, Seshadri DR, Dhavalikar PS, et al., 2016, A Review of Three-Dimensional Printing in Tissue Engineering. Tissue Eng Part B Rev, 22:298–310. https://doi.org/10.1089/ten.teb.2015.0464
8. Yan Q, Dong H, Su J, et al., 2018, A Review of 3D Printing Technology for Medical Applications. Engineering, 4:729–42. https://doi.org/10.1016/j.eng.2018.07.021
9. Gillispie G, Prim P, Copus J, et al., 2020, Assessment Methodologies for Extrusion-based Bioink Printability. Biofabrication, 12:022003. https://doi.org/10.1088/1758-5090/ab6f0d
10. Ozbolat IT, Hospodiuk M, 2016, Current Advances and Future Perspectives in Extrusion-based Bioprinting. Biomaterials, 76:321–43. https://doi.org/10.1016/j.biomaterials.2015.10.076
11. Jang TS, Jung HD, Pan HM, et al., 2018, 3D Printing of Hydrogel Composite Systems: Recent Advances in Technology for Tissue Engineering. Int J Bioprint, 4:126. https://doi.org/10.18063/IJB.v4i1.126
12. Jo YY, Kim SG, Kwon KJ, et al., 2017, Silk Fibroin-Alginate-Hydroxyapatite Composite Particles in Bone Tissue Engineering Applications In Vivo. Int J Mol Sci, 18:858. https://doi.org/10.3390/ijms18040858
13. Mu X, Agostinacchio F, Xiang N, et al., 2021, Recent Advances in 3D Printing with Protein-based Inks. Prog Polym Sci, 115:101375. https://doi.org/10.1016/j.progpolymsci.2021.101375
14. Demirtas TT, Irmak G, Gumusderelioglu M, 2017, A Bioprintable Form of Chitosan Hydrogel for Bone Tissue Engineering. Biofabrication, 9:035003. https://doi.org/10.1088/1758-5090/aa7b1d
15. Salati MA, Khazai J, Tahmuri AM, et al., 2020, Agarose-Based Biomaterials: Opportunities and Challenges in Cartilage Tissue Engineering. Polymers, 12:1150. https://doi.org/10.3390/polym12051150
16. Agostinacchio F, Mu X, Dire S, et al., 2021, In Situ 3D Printing: Opportunities with Silk Inks. Trends Biotechnol, 39:719–30. https://doi.org/10.1016/j.tibtech.2020.11.003
17. Perrone GS, Leisk GG, Lo TJ, et al., 2014, The Use of Silk based Devices for Fracture Fixation. Nat Commun, 5:3385. https://doi.org/10.1038/ncomms4385
18. Zhao Y, Zhu ZS, Guan J, et al., 2021, Processing, Mechanical Properties and Bio-applications of Silk Fibroin-based High strength Hydrogels. Acta Biomater, 125:57–71. https://doi.org/10.1016/j.actbio.2021.02.018
19. Farokhi M, Mottaghitalab F, Samani S, et al., 2018, Silk Fibroin/Hydroxyapatite Composites for Bone Tissue Engineering. Biotechnol Adv, 36:68–91. https://doi.org/10.1016/j.biotechadv.2017.10.001
20. Coelho F, Cavicchioli M, Specian SS, et al., 2020, Silk Fibroin/Hydroxyapatite Composite Membranes: Production, Characterization and Toxicity Evaluation. Toxicology In Vitro, 62:104670. https://doi.org/10.1016/j.tiv.2019.104670
21. Cheng W, Ding Z, Zheng X, et al., 2020, Injectable Hydrogel Systems with Multiple Biophysical and Biochemical Cues for Bone Regeneration. Biomater Sci, 8:2537–48. https://doi.org/10.1039/d0bm00104j
22. Huang T, Fan C, Zhu M, et al., 2019, 3D-printed Scaffolds of Biomineralized Hydroxyapatite Nanocomposite on Silk Fibroin for Improving Bone Regeneration. Appl Surface Sci, 467:345–53. https://doi.org/10.1016/j.apsusc.2018.10.166
23. Kundu B, Brancato V, Oliveira JM, et al., 2020, Silk Fibroin Promotes Mineralization of Gellan Gum Hydrogels. Int J Biol Macromol, 153:1328–34. https://doi.org/10.1016/j.ijbiomac.2019.10.269
24. Zhang H, You R, Yan K, et al., 2020, Silk as Templates for Hydroxyapatite Biomineralization: A Comparative Study of Bombyx mori and Antheraea pernyi Silkworm Silks. Int J Biol Macromol, 164:2842–50. https://doi.org/10.1016/j.ijbiomac.2020.08.142
25. Li H, Li N, Zhang H, et al., 2020, Three-Dimensional Bioprinting of Perfusable Hierarchical Microchannels with Alginate and Silk Fibroin Double Cross-linked Network. 3d Print Addit Manuf, 7:78–84. https://doi.org/10.1089/3dp.2019.0115
26. Meng ZY, Wang L, Shen LY, et al., 2021, Supercritical Carbon Dioxide Assisted Fabrication of Biomimetic Sodium Alginate/Silk Fibroin Nanofibrous Scaffolds. J Appl Polym Sci, 138:51421. https://doi.org/10.1002/app.51421
27. Beck EC, Barragan M, Tadros MH, et al., 2016, Approaching the Compressive Modulus of Articular Cartilage with a Decellularized Cartilage-based Hydrogel. Acta Biomater, 38:94–105. https://doi.org/10.1016/j.actbio.2016.04.019
28. Loh QL, Choong C, 2013, Three-Dimensional Scaffolds for Tissue Engineering Applications: Role of Porosity and Pore Size. Tissue Eng Part B Rev, 19:485–502. https://doi.org/10.1089/ten.teb.2012.0437
29. Le BQ, Nurcombe V, Cool SM, et al., 2018, The Components of Bone and What They Can Teach Us about Regeneration. Materials, 11:14. https://doi.org/10.3390/ma11010014
30. Fitzpatrick V, Martin-Moldes Z, Deck A, et al., 2021, Functionalized 3D-printed Silk-hydroxyapatite Scaffolds for Enhanced Bone Regeneration with Innervation and Vascularization. Biomaterials, 276:120995. https://doi.org/10.1016/j.biomaterials.2021.120995
31. Arahira T, Todo M, 2019, Development of Novel Collagen Scaffolds with Different Bioceramic Particles for Bone Tissue Engineering. Compos Commun, 16:30–2. https://doi.org/10.1016/j.coco.2019.08.012
32. Zhou H, Yang L, Gbureck U, et al., 2021, Monetite, an Important Calcium Phosphate Compound-its Synthesis, Properties and Applications in Orthopedics. Acta Biomater, 127:41–55. https://doi.org/10.1016/j.actbio.2021.03.050
33. Bohner M, Gbureck U, 2008, Thermal Reactions of Brushite Cements. J Biomed Mater Res Part B Appl Biomater, 84B:375–85. https://doi.org/375-385,10.1002/jbm.b.30881.
34. Tamimi F, Sheikh Z, Barralet J, 2012, Dicalcium Phosphate Cements: Brushite and Monetite. Acta Biomater, 8:474–87. https://doi.org/10.1016/j.actbio.2011.08.005
35. Idowu B, Cama G, Deb S, et al., 2014, In Vitro Osteoinductive Potential of Porous Monetite for Bone Tissue Engineering. J Tissue Eng, 5:2041731414536572. https://doi.org/2041731414536572-2041731414536572
36. Torres J, Tamimi I, Cabrejos-Azama J, et al., 2015, Monetite Granules Versus Particulate Autologous Bone in Bone Regeneration. Ann Anat, 200:126–33. https://doi.org/10.1016/j.aanat.2015.03.008
37. Motameni A, Alshemary AZ, Evis Z, 2021, A Review of Synthesis Methods, Properties and Use of Monetite Cements as Filler for Bone Defects. Ceram Int, 47:13245–56. https://doi.org/10.1016/j.ceramint.2021.01.240
38. Jin Y, Kundu B, Cai Y, et al., 2015, Bio-inspired Mineralization of Hydroxyapatite in 3D Silk Fibroin Hydrogel for BoneTissue Engineering. Colloids Sur B Biointerf, 134:339–45. https://doi.org/10.1016/j.colsurfb.2015.07.015
39. Chen J, Wang H, Wu Y, et al., 2022, Biocompatible Octacalcium Phosphate/Sodium Alginate/Silk Fibroin Composite Scaffolds for Bone Regeneration. Mater Today Commun, 31:103312. https://doi.org/10.1016/j.mtcomm.2022.103312
40. Mo C, Holland C, Porter D, et al., 2009, Concentration State Dependence of the Rheological and Structural Properties of Reconstituted Silk. Biomacromolecules, 10:2724–8. https://doi.org/10.1021/bm900452u
41. Hu X, Kaplan D, Cebe P, 2006, Determining Beta-sheet Crystallinity in Fibrous Proteins by Thermal Analysis and Infrared Spectroscopy. Macromolecules, 39:6161–70. https://doi.org/10.1021/ma0610109
42. Horan RL, Antle K, Collette AL, et al., 2005, In Vitro Degradation of Silk Fibroin. Biomaterials, 26:3385–93. https://doi.org/10.1016/j.biomaterials.2004.09.020
43. He GP, Pan XY, Liu X, et al., 2020, HIF-1 Alpha-Mediated Mitophagy Determines ZnO Nanoparticle-Induced Human Osteosarcoma Cell Death both In Vitro and In Vivo. ACS Appl Mater Interf, 12:48296–309. https://doi.org/10.1021/acsami.0c12139
44. Mao Z, Bi X, Ye F, et al., 2020, Controlled Cryogelation and Catalytic Cross-Linking Yields Highly Elastic and Robust Silk Fibroin Scaffolds. ACS Biomater Sci Eng, 6:4512–22. https://doi.org/10.1021/acsbiomaterials.0c0075
45. Kasoju N, Hawkins N, Pop-Georgievski O, et al., 2016, Silk Fibroin Gelation via Non-solvent Induced Phase Separation. Biomater Sci, 4:460–73. https://doi.org/10.1039/c5bm00471c
46. Bi X, Li L, Mao Z, et al., 2020, The Effects of Silk Layer-by-layer Surface Modification on the Mechanical and Structural Retention of Extracellular Matrix Scaffolds. Biomater Sci, 8:4026–38. https://doi.org/10.1039/d0bm00448k
47. Yan LP, Silva-Correia J, Correia C, et al., 2013, Bioactive Macro/Micro Porous Silk Fibroin/Nano-Sized Calcium Phosphate Scaffolds with Potential for Bone-Tissue-Engineering Applications. Nanomedicine, 8:359–78. https://doi.org/10.2217/nnm.12.118
48. Zhang Y, Wu C, Friis T, et al., 2010, The Osteogenic Properties of CaP/Silk Composite Scaffolds. Biomaterials, 31:2848–56. https://doi.org/10.1016/j.biomaterials.2009.12.049
49. Marsh RE, Corey RB, Pauling L, 1955, An Investigation of the Structure of Silk Fibroin. Biochim Biophys Acta, 16:1–34. https://doi.org/10.1016/0006-3002(55)90178-5
50. Tamada Y, 2005, New Process to Form a Silk Fibroin Porous 3-D Structure. Biomacromolecules, 6:3100–6. https://doi.org/.1021/bm050431f
51. Bertassoni LE, Cardoso JC, Manoharan V, et al., 2014, Direct-write Bioprinting of Cell-laden Methacrylated Gelatin Hydrogels. Biofabrication, 6:024105. https://doi.org/10.1088/1758-5082/6/2/024105
52. Morris ER, Rees DA, Thom D, et al., 1978, Chiroptical and Stoichiometric Evidence of a Specific, Primary Dimerisation Process in Alginate Gelation. Carbohydr Res, 66:145–54. https://doi.org/10.1016/S0008-6215(00)83247-4
53. Ming J, Jiang Z, Wang P, et al., 2015, Silk Fibroin/Sodium Alginate Fibrous Hydrogels Regulated Hydroxyapatite Crystal Growth. Mater Sci Eng C Mater Biol Appl, 51:287–93. https://doi.org/10.1016/j.msec.2015.03.014
54. Wu JW, Liu MJ, Wang L, et al., 2020, Influence of Silk Fibroin/Sodium Alginate Coatings on the Mineralization of Silk Fibroin Fiber Artificial Ligament Prototypes. Text Res J, 90:1590–601. https://doi.org/10.1177/0040517519898156