Flexible, Wearable and Fully-printed Smart Patch for pH and Hydration Sensing in Wounds
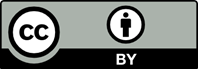
Wound healing is a complex and dynamic regeneration process, wherein the physical and chemical parameters are continuously changing. Its management and monitoring can provide immense benefits, especially for bed-ridden patients. This work reports a low-cost, flexible, and fully printed on-skin patch sensor to measure the change in pH and fluid content in a wound. Such a bendable sensor can also be easily incorporated in a wound dressing. The sensor consists of different electrodes printed on polydimethylsiloxane (PDMS) substrate for pH and moisture sensing. The fabricated sensor patch has a sensitivity of 7.1 ohm/pH for wound pH levels. The hydration sensor results showed that moisture levels on a semi-porous surface can be quantified through resistance change.
1. Kathawala MH, Ng WL, Liu D, et al., 2019, Healing of Chronic Wounds: An Update of Recent Developments and Future Possibilities. Tissue Engineering Part B: Reviews, 25:429–44. https://doi.org/10.1089/ten.TEB.2019.0019
2. Gianino E, Miller C, Gilmore J, 2018, Smart Wound Dressings for Diabetic Chronic Wounds. Bioengineering, 5:51. https://doi.org/10.3390/bioengineering5030051
3. Dubal DP, Chodankar NR, Kim DH, et al., 2018, Towards Flexible Solid-state Supercapacitors for Smart and Wearable Electronics. Chem Soc, 47:2065–129. https://doi.org/10.1039/C7CS00505A
4. Lv T, Liu M, Zhu D, et al., 2018, Nanocarbon‐based Materials for Flexible All‐solid‐State Supercapacitors. Adv Mater, 30:1705489. https://doi.org/10.1002/adma.201705489
5. Weng W, Yang J, Zhang Y, et al., 2020, A Route Toward Smart System Integration: From Fiber Design to Device Construction. Adv Mater, 32:1902301. https://doi.org/10.1002/adma.201902301
6. Chen Y, Pötschke P, Pionteck J, et al., 2018, Smart Cellulose/Graphene Composites Fabricated by In Situ Chemical Reduction of Graphene Oxide for Multiple Sensing Applications. J Mater Chem A, 6:7777–85. https://doi.org/10.1039/C8TA00618K
7. Li Q, Zhang J, Li Q, et al., 2019, Review of Printed Electrodes for Flexible Devices. Front Mater, 5:77. https://doi.org/10.3389/fmats.2018.00077
8. He T, Wang H, Wang J, et al., 2019, Self‐sustainable Wearable Textile Nano‐energy Nano‐system (NENS) for Next generation Healthcare Applications. Adv Sci, 6:1901437. https://doi.org/10.1002/advs.201901437
9. Jin Y, Chen G, Lao K, et al., 2020, Identifying Human Body States by Using a Flexible Integrated Sensor. npj Flex Electron, 4:1–8. https://doi.org/10.1038/s41528-020-00090-9
10. Schneider LA, Korber A, Grabbe S, et al., 2007, Influence of pH on Wound-healing: A New Perspective for Woundtherapy? Arch Dermatol, 298:413–20. https://doi.org/10.1007/s00403-006-0713-x
11. Goh GL, Agarwala S, Tan YJ, et al., 2018, A Low Cost and Flexible Carbon Nanotube pH Sensor Fabricated Using Aerosol Jet Technology for Live Cell Applications. Sens Actuators B Chem, 260:227–35. https://doi.org/10.1016/j.snb.2017.12.127
12. Armstrong DG, Lavery LA, Liswood PJ, et al., 1997, Infrared Dermal Thermometry for the High-risk Diabetic Foot. Phys Ther, 77:169–75. https://doi.org/10.1093/ptj/77.2.169
13. Bharara M, Schoess J, Nouvong A, et al., 2010, Wound Inflammatory Index: A “Proof of Concept” Study to Assess Wound Healing Trajectory. Thousand Oaks, California: SAGE Publications.
14. Liu X, Zweier JL, 2001, A Real-Time Electrochemical Technique for Measurement of Cellular Hydrogen Peroxide Generation and Consumption: Evaluation in Human Polymorphonuclear Leukocytes. Free Radic Biol Med, 31:894–901. https://doi.org/10.1016/s0891-5849(01)00665-7
15. Test ST, Weiss S, 1984, Quantitative and Temporal Characterization of the Extracellular H2O2 Pool Generated by Human Neutrophils. J Biol Chem, 259:399–405. https://doi.org/10.1016/s0021-9258(17)43674-x
16. McColl D, Cartlidge B, Connolly P, 2007, Real-Time Monitoring of Moisture Levels in Wound Dressings In Vitro: An Experimental Study. Int J Surg, 5:316–22. https://doi.org/10.1016/j.ijsu.2007.02.008
17. Cho M, Hunt TK, Hussain MZ, 2001, Hydrogen Peroxide Stimulates Macrophage Vascular Endothelial Growth Factor Release. Am J Physiol Heart Circ Physiol, 280:H2357–63. https://doi.org/10.1152/ajpheart.2001.280.5.H2357
18. Trabold O, Wagner S, Wicke C, et al., 2003, Lactate and Oxygen Constitute a Fundamental Regulatory Mechanism in Wound Healing. Wound Repair Regen, 11:504–9. https://doi.org/10.1046/j.1524-475X.2003.11621.x
19. Lee H, Song C, Hong YS, et al., 2017, Wearable/Disposable Sweat-based Glucose Monitoring Device with Multistage Transdermal Drug Delivery Module. Sci Adv, 3:e1601314. https://doi.org/10.1126/sciadv.1601314
20. Yu Y, Li P, Zhu C, et al., 2019, Multifunctional and Recyclable Photothermally Responsive Cryogels as Efficient Platforms for Wound Healing. Adv Funct Mater, 29:1904402. https://doi.org/10.1002/adfm.201904402
21. Ninan N, Forget A, Shastri VP, et al., 2016, Antibacterial and Anti-inflammatory pH-Responsive Tannic Acid-carboxylated Agarose Composite Hydrogels for Wound Healing. ACS Appl Mater Interfaces, 8:28511–21. https://doi.org/10.1021/acsami.6b10491
22. Piva RH, Rocha MC, Piva DH, et al., 2018, Acidic Dressing Based on Agarose/CS2. 5H0. 5PW12O40 Nanocomposite for Infection Control in Wound Care. ACS Appl Mater Interfaces, 10:30963–72. https://doi.org/10.1021/acsami.8b09066
23. Zlotogorski A, 1987, Distribution of Skin Surface pH on the Forehead and Cheek of Adults. Arch Dermatol, 279:398–401. https://doi.org/10.1007/BF00412626
24. Dargaville TR, Farrugia BL, Broadbent JA, et al., 2013, Sensors and Imaging for Wound Healing: A Review. Biosens Bioelectron, 41:30–42. https://doi.org/10.1016/j.bios.2012.09.029
25. Schmid-Wendtner MH, Korting HC, 2006, The pH of the Skin Surface and its Impact on the Barrier Function. Skin Pharmacol Physiol, 19:296–302. https://doi.org/10.1159/000094670
26. Ehlers C, Ivens U, Møller M, et al., 2001, Comparison of Two pH Meters Used for Skin Surface pH Measurement: The pH Meter “pH900” from Courage and Khazaka Versus the pH Meter “1140” from Mettler Toledo. Skin Res Technol, 7:84–9. https://doi.org/10.1034/j.1600-0846.2001.70205.x
27. Wang M, Wang C, Chen M, et al., 2019, Efficient Angiogenesis-based Diabetic Wound Healing/Skin Reconstruction through Bioactive Antibacterial Adhesive Ultraviolet Shielding Nanodressing with Exosome Release. ACS Nano, 13:10279–93. https://doi.org/10.1021/acsnano.9b03656
28. Jones EM, Cochrane CA, Percival SL, 2015, The Effect of pH on the Extracellular Matrix and Biofilms. Adv Wound Care, 4:431–9. https://doi.org/10.1089/wound.2014.0538
29. Baldini F, Bechi P, Bracci S, et al., 1995, In Vivo Optical-Fibre pH Sensor for Gastro-Oesophageal Measurements. Sens Actuators B Chem, 29:164–8. https://doi.org/10.1016/0925-4005(95)01678-3
30. Sudakov-Boreysha L, Dinnar U, Nemirovsky Y, 2004, New ISFET Catheters Encapsulation Techniques for Brain PH In-Vivo Monitoring, Proceedings of the 2004 11th IEEE International Conference on Electronics, Circuits and Systems. ICECS, p424-426.
31. Yoshioka Y, Oikawa H, Ehara S, et al., 2002, Noninvasive Estimation of Temperature and pH in Human Lower Leg Muscles Using 1H Nuclear Magnetic Resonance Spectroscopy. Spectroscopy, 16:183–90.https://doi.org/10.1155/2002/392987
32. Pietsch C, Hoogenboom R, Schubert US, 2009, Soluble Polymeric Dual Sensor for Temperature and pH Value. Angew Chem, 121:5763–6. https://doi.org/10.1002/anie.200901071
33. Safavi A, Abdollahi H, 1998, Optical Sensor for High pH Values. Anal Chim Acta, 367:167–73. https://doi.org/10.1016/S0003-2670(98)00079-8
34. Soyemi O, Shear M, Landry M, et al., 2005, In Vivo Non-invasive Measurement of Muscle pH during Exercise Using Near-infrared Spectroscopy, Smart Medical and Biomedical Sensor Technology III, International Society for Optics and Photonics, 60070N.
35. Van der Schueren L, De Clerck K, 2010, The Use of pH Indicator Dyes for pH-Sensitive Textile Materials. Text Res J,80:590–603. https://doi.org/10.1177/0040517509346443
36. Shoghi F, Mal S, Tie M, et al., 2021, pH Responsive Platinum-Coated Single-Walled Carbon Nanotube Optical Sensor with Internal Reference. Carbon, 184:659–68. https://doi.org/10.1016/j.carbon.2021.08.065
37. Guinovart T, Valdés‐Ramírez G, Windmiller JR, et al., 2014, Bandage‐based Wearable Potentiometric Sensor for Monitoring Wound pH. Electroanalysis, 26:1345–53. https://doi.org/10.1002/elan.201300558
38. Arafat MT, Mahmud MM, Wong SY, et al., 2021, PVA/PAA Based Electrospun Nanofibers with pH-responsive Color Change Using Bromothymol Blue and on-Demand Ciprofloxacin Release Properties. J Drug Deliv Sci Technol, 61:102297. https://doi.org/10.1016/j.jddst.2020.102297
39. Bazbouz MB, Tronci G, 2019, Two-layer Electrospun System Enabling Wound Exudate Management and Visual Infection Response. Sensors, 19:991. https://doi.org/10.3390/s19050991
40. Tamayol A, Akbari M, Zilberman Y, et al., 2016, pH‐Sensing Hydrogel Fibers: Flexible pH‐Sensing Hydrogel Fibers for Epidermal Applications. Adv Healthc Mater, 5:711–9. https://doi.org/10.1002/adhm.201500553
41. Mirani B, Pagan E, Currie B, et al., 2017, An Advanced Multifunctional Hydrogel‐Based Dressing for Wound Monitoring and Drug Delivery. Adv Healthc Mater, 6:1700718. https://doi.org/10.1002/adhm.201700718
42. Yang P, Zhu Z, Zhang T, et al., 2019, Orange‐Emissive Carbon Quantum Dots: Toward Application in Wound pH Monitoring Based on Colorimetric and Fluorescent Changing. Small, 15:1902823. https://doi.org/10.1002/smll.201902823
43. Begu S, Mordon SR, Desmettre T, et al., 2005, Fluorescence Imaging Method for In Vivo pH Monitoring during Liposomes Uptake in Rat Liver Using a pH-Sensitive Fluorescent Dye. J Biomed Opt, 10:024008. https://doi.org/10.1117/1.1899685
44. Malkaj P, Dalas E, Vitoratos E, et al., 2006, pH Electrodes Constructed from Polyaniline/Zeolite and Polypyrrole/Zeolite Conductive Blends. J Appl Polym Sci, 101:1853–6. https://doi.org/10.1002/app.23590
45. Trupp S, Alberti M, Carofiglio T, et al., 2010, Development of pH Sensitive Indicator Dyes for the Preparation of Micro-patterned Optical Sensor Layers. Sens Actuators B Chem, 150:206–10. https://doi.org/10.1016/j.snb.2010.07.015
46. Mohr GJ, Müller H, Bussemer B, et al., 2008, Design of Acidochromic Dyes for Facile Preparation of pH Sensor Layers. Anal Bioanal Chem, 392:1411–8. https://doi.org/10.1007/s00216-008-2428-7
47. Yuan F, Ding L, Li Y, et al., 2015, Multicolor Fluorescent Graphene Quantum Dots Colorimetrically Responsive to All pH and a Wide Temperature Range. Nanoscale, 7:11727–33. https://doi.org/10.1039/C5NR02007G
48. Wang L, Li M, Li W, et al., 2018, Rationally Designed Efficient Dual-Mode Colorimetric/Fluorescence Sensor Based on Carbon Dots for Detection of pH and Cu2+ Ions. ACS Sustain Chem Eng, 6:12668–74. https://doi.org/10.1021/acssuschemeng.8b01625
49. Ferrer‐Anglada N, Kaempgen M, Roth S, 2006, Transparent and Flexible Carbon Nanotube/Polypyrrole and Carbon Nanotube/Polyaniline pH Sensors. Phys Status Solidi B, 243:3519–23. https://doi.org/10.1002/pssb.200669220
50. Liao Y, Zhang C, Zhang Y, et al., 2011, Carbon Nanotube/Polyaniline Composite Nanofibers: Facile Synthesis and Chemosensors. Nano Lett, 11:954–9. https://doi.org/10.1021/nl103322b
51. Gou P, Kraut ND, Feigel IM, et al., 2014, Carbon Nanotube Chemiresistor for Wireless pH Sensing. Sci Rep, 4:1–6. https://doi.org/10.1038/srep04468
52. Qin Y, Kwon HJ, Subrahmanyam A, et al., 2016, Inkjet printed Bifunctional Carbon Nanotubes for pH Sensing. Mater Lett, 176:68–70. https://doi.org/10.1016/j.matlet.2016.04.048
53. Jiang T, Munguia-Lopez JG, Flores-Torres S, et al., 2019, Extrusion Bioprinting of Soft Materials: An Emerging Technique for Biological Model Fabrication. Appl Phys Rev, 6:011310. https://doi.org/10.1063/1.5059393
54. Li X, Liu B, Pei B, et al., 2020, Inkjet Bioprinting of Biomaterials. Chem Rev, 120:10793–833. doi.org/10.1021/acs.chemrev.0c00008
55. Ng WL, Lee JM, Zhou M, et al., 2020, Vat Polymerization based Bioprinting Process, Materials, Applications and Regulatory Challenges. Biofabrication, 12:022001. doi.org/10.1088/1758-5090/ab6034
56. Cremers NA, Suttorp M, Gerritsen MM, et al., 2015, Mechanical Stress Changes the Complex Interplay between HO-1, Inflammation and Fibrosis, during Excisional Wound Repair. Front Med, 2:86. https://doi.org/10.3389/fmed.2015.00086
57. Barnes LA, Marshall CD, Leavitt T, et al., 2018, Mechanical Forces in Cutaneous Wound Healing: Emerging Therapies to Minimize Scar Formation. Adv Wound Care, 7:47–56. https://doi.org/10.1089/wound.2016.0709
58. Urschel J, Scott P, Williams H, 1988, The Effect of Mechanical Stress on Soft and Hard Tissue Repair; a Review. Br J Plast Surg, 41:182–6. https://doi.org/10.1016/0007-1226(88)90049-5
59. Liang FC, Chang YW, Kuo CC, et al., 2019, A Mechanically Robust Silver Nanowire Polydimethylsiloxane Electrode Based on Facile Transfer Printing Techniques for Wearable Displays. Nanoscale, 11:1520–30. https://doi.org/10.1039/C8NR08819E
60. Boateng J, 2020, Therapeutic Dressings and Wound Healing Applications. New York: John Wiley and Sons.
61. Lee D, Cui T, 2009, pH-dependent Conductance Behaviors of Layer-by-layer Self-Assembled Carboxylated Carbon Nanotube Multilayer Thin-film Sensors. J Vac Sci Technol B, 27:842–8. https://doi.org/10.1116/1.3002386
62. Li P, Martin CM, Yeung KK, et al., 2011, Dielectrophoresis Aligned Single-walled Carbon Nanotubes as pH Sensors. Biosensors, 1:23–35. https://doi.org/10.3390/bios1010023
63. Cutting KF, 2003, Wound Exudate: Composition and Functions. Br J Community Nurs, 8 Suppl 3:S4–9. https://doi.org/10.12968/bjcn.2003.8.sup3.11577