Replication of an intervertebral disc using an in-house bioprinter: A proof-of-concept study
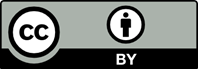
The intervertebral disc, a fibro-cartilaginous structure, contains a gelatinous core (nucleus pulposus) surrounded by collagen fiber lamellae (annulus fibrosus). Intervertebral disc degeneration leads to a debilitating disorder, and new treatments are currently being developed. Unfortunately, conventional monolayer in vitro models fail to predict the clinical efficacy of novel therapies accurately. Here, we report a bioprinted construct that mimics the macroscopic and microscopic architecture of the intervertebral disc. First, a 3D model was created from a histological section of a sheep lumbar intervertebral disc. The printability of the ink, gelatin (7% w/v), alginate (0.6% w/v), and hyaluronic acid (0.2% w/v) was optimized by varying the printing pressure (70–110 kPa), printing speed (2–10 mm/s), and nozzle type (needle or tip). Nucleus pulposus and annulus fibrosus cells, harvested from 4-month-old lambs, were bioprinted (5 × 105 cells/mL) using an in-house extrusion bioprinter. Cell viability (live/ dead assay), shape (actin immunostaining), distribution (confocal microscopy), and matrix synthesis (immunostaining) were evaluated after 21 days of culture. We used a parametric study to quantify and optimize the factors (pressure, printing speed, nozzle type) influencing the filament width. The 3D construct exhibited fidelity to the initial design and maintained stability in length, width, and height for 21 days. Fluorescent labeling confirmed the distribution of nucleus pulposus and annulus fibrosus cells in each tissue, replicating the native intervertebral disc structure. We also evidenced cell viability and collagen type 1 synthesis. This bioprinted construct offers a promising alternative to current in vitro models, potentially enabling more relevant preclinical evaluations.

- Wilke HJ, Galbusera F. Biomechanics of the healthy and diseased spine. In: Biological Approaches to Spinal Disc Repair and Regeneration for Clinicians (1st ed.). Stuttgart, Germany: Georg Thieme Verlag KG; 2017:30-36. doi: 10.1055/b-0037-145106
- Amin DB, Lawless IM, Sommerfeld D, Stanley RM, Ding B, Costi JJ. The effect of six degree of freedom loading sequence on the in-vitro compressive properties of human lumbar spine segments. J Biomech. 2016;49(14):3407-3414. doi: 10.1016/j.jbiomech.2016.09.009
- Gravel JT, Abduljabbar FH, Ouellet J, Haglund L. The human spinal disc: relevant anatomy and physiology. In: Biological Approaches to Spinal Disc Repair and Regeneration for Clinicians (1st ed.). Stuttgart, Germany: Georg Thieme Verlag KG; 2017:2-10. doi: 10.1055/b-0037-145103
- Lavignolle B. The intervertebral disc. In: Vital JM, Cawley DT, eds. Spinal Anatomy : Modern Concepts. Springer International Publishing; 2020:207-216. doi: 10.1007/978-3-030-20925-4_14
- Al-Abbasi M, Al-Rubai AJF, Assi MH. Quantification of the water content of human intervertebral discs in various regions and conditions. Al-Rafidain J Med Sci. 2024;6(2):43-47. doi: 10.54133/ajms.v6i2.715
- Johnstone B, Bayliss MT. The large proteoglycans of the human intervertebral disc. Changes in their biosynthesis and structure with age, topography, and pathology. Spine. 1995;20(6):674-684. doi: 10.1097/00007632-199503150-00008
- Pattappa G, Li Z, Peroglio M, Wismer N, Alini M, Grad S. Diversity of intervertebral disc cells: phenotype and function: diversity of intervertebral disc cells. J Anat. 2012;221(6):480-496. doi: 10.1111/j.1469-7580.2012.01521.x
- Sivan SS, Hayes AJ, Wachtel E, et al. Biochemical composition and turnover of the extracellular matrix of the normal and degenerate intervertebral disc. Eur Spine J. 2014;23 (Suppl. 3):S344-S353. doi: 10.1007/s00586-013-2767-8
- Zhang S, Liu W, Chen S, et al. Extracellular matrix in intervertebral disc: basic and translational implications. Cell Tissue Res. 2022;390(1):1-22. doi: 10.1007/s00441-022-03662-5
- Vogel A, Pioletti DP. Damping properties of the nucleus pulposus. Clin Biomech. 2012;27(9):861-865. doi: 10.1016/j.clinbiomech.2012.06.005
- Krull C, Lutton A, Olesik J, Walter B. A method for measuring intra-tissue swelling pressure using a needle micro-osmometer. Eur Cell Mater. 2020;40:146-159. doi: 10.22203/eCM.v040a09
- Urban JPG, McMullin JF. Swelling pressure of the inervertebral disc: influence of proteoglycan and collagen contents. Biorheology. 1985;22(2):145-157. doi: 10.3233/BIR-1985-22205
- Pazzaglia UE, Salisbury JR, Byers PD. Development and involution of the Notochord in the human spine. J R Soc Med. 1989;82(7):413-415. doi: 10.1177/014107688908200714
- Tan Z, Chen P, Dong X, et al. Progenitor-like cells contributing to cellular heterogeneity in the nucleus pulposus are lost in intervertebral disc degeneration. Cell Rep. 2024;43(6):114342. doi: 10.1016/j.celrep.2024.114342
- Risbud MV, Schoepflin ZR, Mwale F, et al. Defining the phenotype of young healthy nucleus pulposus cells: recommendations of the Spine Research Interest Group at the 2014 annual ORS meeting. J Orthop Res. 2015;33(3):283-293. doi: 10.1002/jor.22789
- Mwale F, Roughley P, Antoniou J. Distinction between the extracellular matrix of the nucleus pulposus and hyaline cartilage: a requisite for tissue engineering of intervertebral disc. Eur Cells A¨nd Mater. 2004;8:58-64. doi: 10.22203/eCM.v008a06
- Tomaszewski KA, Walocha JA, Mizia E, Gładysz T, Głowacki R, Tomaszewska R. Age- and degeneration-related variations in cell density and glycosaminoglycan content in the human cervical intervertebral disc and its endplates. Pol J Pathol. 2015;66(3):296-300. doi: 10.5114/pjp.2015.54964
- Urban JPG, Roberts S, Ralphs JR. The nucleus of the intervertebral disc from development to degeneration. Am Zool. 2000;40(1):53-061. doi: 10.1093/icb/40.1.53
- Marchand F, Ahmed AM. Investigation of the laminate structure of lumbar disc anulus fibrosus. Spine. 1990;15(5):402-410. doi: 10.1097/00007632-199005000-00011
- Liang H, Luo R, Li G, Zhang W, Song Y, Yang C. The proteolysis of ECM in intervertebral disc degeneration. Int J Mol Sci. 2022;23(3):1715. doi: 10.3390/ijms23031715
- Costi JJ, Stokes IA, Gardner-Morse M, Laible JP, Scoffone HM, Iatridis JC. Direct measurement of intervertebral disc maximum shear strain in six degrees of freedom: motions that place disc tissue at risk of injury. J Biomech. 2007;40(11):2457-2466. doi: 10.1016/j.jbiomech.2006.11.006
- Guerin HAL, Elliott DM. Structure and properties of soft tissues in the spine. In: Spine Technology Handbook. Academic Press; 2006:35-62. doi: 10.1016/B978-012369390-7/50004-6
- Torre OM, Mroz V, Bartelstein MK, Huang AH, Iatridis JC. Annulus fibrosus cell phenotypes in homeostasis and injury: implications for regenerative strategies. Ann N Y Acad Sci. 2019;1442(1):61-78. doi: 10.1111/nyas.13964
- Liebscher T, Haefeli M, Wuertz K, Nerlich AG, Boos N. Age-related variation in cell density of human lumbar intervertebral disc. Spine. 2011;36(2):153-159. doi: 10.1097/brs.0b013e3181cd588c
- Samanta A, Lufkin T, Kraus P. Intervertebral disc degeneration—current therapeutic options and challenges. Front Public Health. 2023;11:1156749. doi: 10.3389/fpubh.2023.1156749
- Casiano VE, Sarwan G, Dydyk AM, Varacallo M. Back pain. In: StatPearls. StatPearls Publishing; 2023. Accessed March 29, 2023. http://www.ncbi.nlm.nih.gov/books/NBK538173/
- Hay SI, Abajobir AA, Abate KH, et al. Global, regional, and national disability-adjusted life-years (DALYs) for 333 diseases and injuries and healthy life expectancy (HALE) for 195 countries and territories, 1990–2016: a systematic analysis for the Global Burden of Disease Study 2016. Lancet. 2017;390(10100):1260-1344. doi: 10.1016/S0140-6736(17)32130-X
- Meddeba M, Hatirab F, Habboubia K, Mestiria. Biomechanical Study of the Behavior of the Intervertebral Disc: Intact Lumbar Spine Versus Arthrodesis. J Orthop Bone Disord. 2024;8(2):1-4. doi: 10.23880/jobd-16000265
- Sampara P, Banala RR, Vemuri SK, Av GR, Gpv S. Understanding the molecular biology of intervertebral disc degeneration and potential gene therapy strategies for regeneration: a review. Gene Ther. 2018;25(2):67-82. doi: 10.1038/s41434-018-0004-0
- Tamagawa S, Schol J, Sakai D. Cell therapy for intervertebral disc regeneration: progress and hurdles in clinical translation. Indian Spine J. 2024;7(2):131-141. doi: 10.4103/isj.isj_17_24
- Liu Y, Zhao Z, Guo C, et al. Application and development of hydrogel biomaterials for the treatment of intervertebral disc degeneration: a literature review. Front Cell Dev Biol. 2023;11:1286223. doi: 10.3389/fcell.2023.1286223
- Doench I, Tran TA, David L, et al. Cellulose nanofiber-reinforced chitosan hydrogel composites for intervertebral disc tissue repair. Biomimetics (Basel). 2019;4(1):19. doi: 10.3390/biomimetics4010019
- Hingert D, Barreto Henriksson H, Baranto A, Brisby H. BMP-3 promotes matrix production in co-cultured stem cells and disc cells from low back pain patients. Tissue Eng Part A. 2020;26(1-2):47-56. doi: 10.1089/ten.tea.2019.0125
- Tang SN, Salazar-Puerta AI, Heimann MK, et al. Engineered extracellular vesicle-based gene therapy for the treatment of discogenic back pain. Biomaterials. 2024;308:122562. doi: 10.1016/j.biomaterials.2024.122562
- Barcellona MN, Ní Néill T, O’Brien FJ, Dixon JE, Curtin CM, Buckley CT. Modulation of inflammation and regeneration in the intervertebral disc using enhanced cell‐penetrating peptides for MicroRNA delivery. Adv NanoBiomed Res. 2024;4(7):2300112. doi: 10.1002/anbr.202300112
- Yuan B, Rudeen K, Li J, et al. Biodegradable microspheres and hydrogel drug delivery system of tumor necrosis factor (TNF) inhibitor and growth differentiation factor 5 (GDF5) reduces disk inflammation in the rabbit model. Spine (Phila Pa 1976). 2023;48(15):E257-E265. doi: 10.1097/BRS.0000000000004686
- Rivera Tapia ED, Meakin JR, Holsgrove TP. In-vitro models of disc degeneration – a review of methods and clinical relevance. J Biomech. 2022;142:111260. doi: 10.1016/j.jbiomech.2022.111260
- Yung Lee J, Hall R, Pelinkovic D, et al. New use of a three-dimensional pellet culture system for human intervertebral disc cells: initial characterization and potential use for tissue engineering. Spine (Phila Pa 1976). 2001;26(21):2316-2322. doi: 10.1097/00007632-200111010-00005
- Le Maitre CL, Freemont AJ, Hoyland JA. The role of interleukin-1 in the pathogenesis of human intervertebral disc degeneration. Arthritis Res Ther. 2005;7(4):R732. doi: 10.1186/ar1732
- McDonnell EE, Buckley CT. Two- and three-dimensional in vitro nucleus pulposus cultures: an in silico analysis of local nutrient microenvironments. JOR Spine. 2022;5(3):e1222. doi: 10.1002/jsp2.1222
- Zhang A, Cheng Z, Chen Y, Shi P, Gan W, Zhang Y. Emerging tissue engineering strategies for annulus fibrosus therapy. Acta Biomater. 2023;167:1-15. doi: 10.1016/j.actbio.2023.06.012
- Liu Y, Peng L, Li L, et al. 3D-bioprinted BMSC-laden biomimetic multiphasic scaffolds for efficient repair of osteochondral defects in an osteoarthritic rat model. Biomaterials. 2021;279:121216. doi: 10.1016/j.biomaterials.2021.121216
- Yuan D, Chen Z, Xiang X, et al. The establishment and biological assessment of a whole tissue-engineered intervertebral disc with PBST fibers and a chitosan hydrogel in vitro and in vivo. J Biomed Mater Res B Appl Biomater. 2019;107(7):2305-2316. doi: 10.1002/jbm.b.34323
- Fusellier M, Clouet J, Gauthier O, Tryfonidou M, Le Visage C, Guicheux J. Degenerative lumbar disc disease: in vivo data support the rationale for the selection of appropriate animal models. Eur Cell Mater. 2020;39:17-48. doi: 10.22203/eCM.v039a02
- Pourchet LJ, Thepot A, Albouy M, et al. Human skin 3D bioprinting using scaffold-free approach. Adv Healthc Mater. 2017;6(4):1601101. doi: 10.1002/adhm.201601101
- Wang J, Cui Z, Maniruzzaman M. Bioprinting: a focus on improving bioink printability and cell performance based on different process parameters. Int J Pharm. 2023;640:123020. doi: 10.1016/j.ijpharm.2023.123020
- Abedi K, Keshvari H, Solati-Hashjin M. Extrusion-based bioprinting: considerations toward gelatin-alginate bioink. Rapid Prototyp J. 2024;30(6):1094-1104. doi: 10.1108/RPJ-06-2023-0207
- Seok JM, Ahn M, Kim D, et al. Decellularized matrix bioink with gelatin methacrylate for simultaneous improvements in printability and biofunctionality. Int J Biol Macromol. 2024;262:130194. doi: 10.1016/j.ijbiomac.2024.130194
- Rajasekaran S, Soundararajan DCR, Nayagam SM, et al. Novel biomarkers of health and degeneration in human intervertebral discs: in-depth proteomic analysis of collagen framework of fetal, healthy, scoliotic, degenerate, and herniated discs. Asian Spine J. 2023;17(1):17-29. doi: 10.31616/asj.2021.0535
- Melrose J, Smith S, Ghosh P, Taylor TKF. Differential expression of proteoglycan epitopes and growth characteristics of intervertebral disc cells grown in alginate bead culture. Cells Tissues Organs. 2001;168(3):137-146. doi: 10.1159/000047829
- Thonar E, An H, Masuda K. Compartmentalization of the matrix formed by nucleus pulposus and annulus fibrosus cells in alginate gel. Biochem Soc Trans. 2002;30(6):874-878. doi: 10.1042/bst0300874
- Ouyang L, Yao R, Zhao Y, Sun W. Effect of bioink properties on printability and cell viability for 3D bioplotting of embryonic stem cells. Biofabrication. 2016;8(3):035020. doi: 10.1088/1758-5090/8/3/035020
- Habib MA, Khoda B. Rheological analysis of bio-ink for 3D bio-printing processes. J Manuf Process. 2022;76:708-718. doi: 10.1016/j.jmapro.2022.02.048
- Magalhães IP, Oliveira PMD, Dernowsek J, Casas EBL, Casas MSL. Investigation of the effect of nozzle design on rheological bioprinting properties using computational fluid dynamics. Matéria (Rio J.) 2019;24(3):e12401. doi: 10.1590/s1517-707620190003.0714
- Chand R, Muhire BS, Vijayavenkataraman S. Computational fluid dynamics assessment of the effect of bioprinting parameters in extrusion bioprinting. Int J Bioprint. 2022; 8(2):545. doi: 10.18063/ijb.v8i2.545
- Reitmaier S, Schmidt H. Review article on spine kinematics of quadrupeds and bipeds during walking. J Biomech. 2020;102:109631. doi: 10.1016/j.jbiomech.2020.109631
- Yoshizato H, Morimoto T, Nonaka T, et al. Animal model for anterior lumbar interbody fusion: a literature review. Spine Surg Relat Res. 2024;8(4):373-382. doi: 10.22603/ssrr.2023-0262
- Duan B, Hockaday LA, Kang KH, Butcher JT. 3D bioprinting of heterogeneous aortic valve conduits with alginate/gelatin hydrogels. J Biomed Mater Res A. 2013;101A(5):1255-1264. doi: 10.1002/jbm.a.34420
- Fedorovich NE, De Wijn JR, Verbout AJ, Alblas J, Dhert WJA. Three-dimensional fiber deposition of cell-laden, viable, patterned constructs for bone tissue printing. Tissue Eng Part A. 2008;14(1):127-133. doi: 10.1089/ten.a.2007.0158
- Wang X, Yan Y, Pan Y, et al. Generation of three-dimensional hepatocyte/gelatin structures with rapid prototyping system. Tissue Eng. 2006;12(1):83-90. doi: 10.1089/ten.2006.12.83
- Gironi P, Petraro L, Santoni S, Dedé L, Colosimo BM. A computational model of cell viability and proliferation of extrusion-based 3D-bioprinted constructs during tissue maturation process. Int J Bioprinting. 2023;9(4):741. doi: 10.18063/ijb.741
- Bruehlmann SB, Rattner JB, Matyas JR, Duncan NA. Regional variations in the cellular matrix of the annulus fibrosus of the intervertebral disc. J Anat. 2002;201(2):159-171. doi: 10.1046/j.1469-7580.2002.00080.x.
- Rastogi P, Kandasubramanian B. Review of alginate-based hydrogel bioprinting for application in tissue engineering. Biofabrication. 2019;11(4):042001. doi: 10.1088/1758-5090/ab331e
- Li QQ, Xu D, Dong QW, Song XJ, Chen YB, Cui YL. Biomedical potentials of alginate via physical, chemical, and biological modifications. Int J Biol Macromol. 2024; 277:134409. doi: 10.1016/j.ijbiomac.2024.134409
- Sandvig I, Karstensen K, Rokstad AM, et al. RGD-peptide modified alginate by a chemoenzymatic strategy for tissue engineering applications. J Biomed Mater Res A. 2015; 103(3):896-906. doi: 10.1002/jbm.a.35230
- Yang J, Yang X, Wang L, et al. Biomimetic nanofibers can construct effective tissue-engineered intervertebral discs for therapeutic implantation. Nanoscale. 2017;9(35):13095-13103. doi: 10.1039/c7nr03944a
- Zhou P, Chu G, Yuan Z, et al. Regulation of differentiation of annulus fibrosus-derived stem cells using heterogeneous electrospun fibrous scaffolds. J Orthop Transl. 2021;26: 171-180. doi: 10.1016/j.jot.2020.02.003
- Gluais M, Clouet J, Fusellier M, et al. In vitro and in vivo evaluation of an electrospun-aligned microfibrous implant for Annulus fibrosus repair. Biomaterials. 2019;205:81-93. doi: 10.1016/j.biomaterials.2019.03.010
- Mordechai HS, Aharonov A, Sharon SE, et al. Toward a mechanically biocompatible intervertebral disc: engineering of combined biomimetic annulus fibrosus and nucleus pulposus analogs. J Biomed Mater Res A. 2023;111(5):618-633. doi: 10.1002/jbm.a.37519
- Moxon SR, McMurran Z, Kibble MJ, Domingos M, Gough JE, Richardson SM. 3D bioprinting of an intervertebral disc tissue analogue with a highly aligned annulus fibrosus via suspended layer additive manufacture. Biofabrication. 2024;17(1):015005. doi: 10.1088/1758-5090/ad8379