The application of 3D bioprinting technology in liver manufacturing: Progress, challenges, and prospects
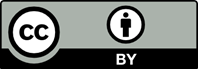
Recreating the complexity of liver structure and the diversity of cells and functions in vitro remains a significant focus in liver tissue engineering. 3D bioprinting technology features advantages in personalized manufacturing, replicating complex internal structures, and precise arrangement of multi-materials and cells, thus holding vast potential in tissue engineering, particularly for liver manufacturing. In this review, we discuss the complexity of the liver structure and function, including its composition and both development and regeneration processes. We then analyze the current limitations in liver transplantation, e.g., donor organ shortage, and the shortcomings of various liver transplantation alternatives, highlighting the importance of 3D bioprinting for liver fabrication. Furthermore, the composition and properties of the bioinks used in the liver 3D-printing process and the corresponding research outcomes are discussed. Notably, we summarize the latest advances and challenges in the field of liver fabrication and explore future development directions, namely vascular and bile duct structures. In summation, this review can provide guidance and inspiration for future research and applications related to liver reconstruction and manufacturing.
- Lemaigre FP. Mechanisms of liver development: concepts for understanding liver disorders and design of novel therapies. Gastroenterology. 2009;137(1):62-79. doi: 10.1053/j.gastro.2009.03.035
- Ober EA, Lemaigre FP. Development of the liver: Insights into organ and tissue morphogenesis. J Hepatol. 2018;68(5):1049-1062. doi: 10.1016/j.jhep.2018.01.005
- Wang X, Yang L, Wang Y-C, et al. Comparative analysis of cell lineage differentiation during hepatogenesis in humans and mice at the single-cell transcriptome level. Cell Res. 2020;30(12):1109-1126. doi: 10.1038/s41422-020-0378-6
- Macchi F, Sadler KC. Unraveling the epigenetic basis of liver development, regeneration and disease. Trends Genet. 2020;36(8):587-597. doi: 10.1016/j.tig.2020.05.002
- Matchett KP, Wilson-Kanamori JR, Portman JR, et al. Multimodal decoding of human liver regeneration. Nature. 2024;630(8015):158-165. doi: 10.1038/s41586-024-07376-2
- Sun T, Pikiolek M, Orsini V, et al. AXIN2<sup>+</sup> Pericentral hepatocytes have limited contributions to liver homeostasis and regeneration. Cell Stem Cell. 2020;26(1):97- 107.e6. doi: 10.1016/j.stem.2019.10.011
- Raasch M, Fritsche E, Kurtz A, Bauer M, Mosig AS. Microphysiological systems meet hiPSC technology - new tools for disease modeling of liver infections in basic research and drug development. Adv Drug Deliv Rev. 2019; 140:51-67. doi: 10.1016/j.addr.2018.06.008
- Heymann F, Tacke F. Immunology in the liver — from homeostasis to disease. Nat Rev Gastroenterol Hepatol. 2016;13(2):88-110. doi: 10.1038/nrgastro.2015.200
- Palakkan AA, Hay DC, PR Anil Kumar, TV Kumary, Ross JA. Liver tissue engineering and cell sources: issues and challenges. Liver Int. 2013;33(5):666-676. doi: 10.1111/liv.12134
- Prior N, Inacio P, Huch M. Liver organoids: from basic research to therapeutic applications. Gut. 2019;68(12): 2228-2237. doi: 10.1136/gutjnl-2019-319256
- Osonoi S, Takebe T. Organoid-guided precision hepatology for metabolic liver disease. J Hepatol. 2024;80(5): 805-821. doi: 10.1016/j.jhep.2024.01.002
- Panwar A, Das P, Tan LP. 3D hepatic organoid-based advancements in LIVER tissue engineering. Bioengineering (Basel). 2021;8(11):185. doi: 10.3390/bioengineering8110185
- Yang Z, Liu X, Cribbin EM, Kim AM, Li JJ, Yong KT. Liver-on-a-chip: considerations, advances, and beyond. Biomicrofluidics. 2022;16(6):061502. doi: 10.1063/5.0106855
- Dalsbecker P, Beck Adiels C, Goksör M. Liver-on-a-chip devices: the pros and cons of complexity. Am J Physiol Gastrointest Liver Physiol. 2022;323(3):G188-G204. doi: 10.1152/ajpgi.00346.2021
- Mirdamadi ES, Kalhori D, Zakeri N, Azarpira N, Solati- Hashjin M. Liver tissue engineering as an emerging alternative for liver disease treatment. Tissue Eng Part B Rev. 2020;26(2):145-163. doi: 10.1089/ten.TEB.2019.0233
- Zhang L, Guan Z, Ye JS, Yin YF, Stoltz JF, de Isla N. Research progress in liver tissue engineering. Biomed Mater Eng. 2017;28(s1):S113-s119. doi: 10.3233/bme-171632
- Agarwal T, Banerjee D, Konwarh R, et al. Recent advances in bioprinting technologies for engineering hepatic tissue. Mater Sci Eng C Mater Biol Appl. 2021;123:112013. doi: 10.1016/j.msec.2021.112013
- Zahra H, Paria P, Polina B, et al. Mimicking the liver function in micro-patterned units: challenges and perspectives in 3D bioprinting. Bioprinting. 2022;27:e00208. doi: 10.1016/j.bprint.2022.e00208
- Arka S, Sourabh G. 3D bioprinting strategies for recapitulation of hepatic structure and function in bioengineered liver: a State-of-the-art review. Curr Opin Biomed Eng. 2024;30:100526. doi: 10.1016/j.cobme.2024.100526
- Agarwal T, Subramanian B, Maiti TK. Liver tissue engineering: challenges and opportunities. ACS Biomater Sci Eng. 2019;5(9):4167-4182. doi: 10.1021/acsbiomaterials.9b00745
- Ordovás L, Park Y, Verfaillie CM. Stem cells and liver engineering. Biotechnol Adv. 2013;31(7):1094-1107. doi: 10.1016/j.biotechadv.2013.07.002
- Ali ASM, Wu D, Bannach-Brown A, et al. 3D bioprinting of liver models: a systematic scoping review of methods, bioinks, and reporting quality. Materials Today Bio. 2024;26:100991. doi: 10.1016/j.mtbio.2024.100991
- Thompson WL, Takebe T. Human liver model systems in a dish. Dev Growth Differ. 2021;63(1):47-58. doi: 10.1111/dgd.12708
- Collardeau-Frachon S, Scoazec J-Y. Vascular development and differentiation during human liver organogenesis. Anat Rec. (Hoboken). 2008;291(6):614-627. doi: 10.1002/ar.20679
- Swartley OM, Foley JF, Livingston DP, 3rd, Cullen JM, Elmore SA. Histology atlas of the developing mouse hepatobiliary hemolymphatic vascular system with emphasis on embryonic days 11.5-18.5 and early postnatal development. Toxicol Pathol. 2016;44(5): 705-725. doi: 10.1177/0192623316630836
- Zhang H, Pu W, Tian X, et al. Genetic lineage tracing identifies endocardial origin of liver vasculature. Nat Genet. 2016;48(5):537-543. doi: 10.1038/ng.3536
- Kang D, Hong G, An S, et al. Bioprinting of multiscaled hepatic lobules within a highly vascularized construct. Small. 2020;16(13):1905505. doi: 10.1002/smll.201905505
- Ben-Moshe S, Itzkovitz S. Spatial heterogeneity in the mammalian liver. Nat Rev Gastroenterol Hepatol. 2019;16(7):395-410. doi: 10.1038/s41575-019-0134-x
- Gissen P, Arias IM. Structural and functional hepatocyte polarity and liver disease. J Hepatol. 2015;63(4): 1023-1037. doi: 10.1016/j.jhep.2015.06.015
- Fu D, Wakabayashi Y, Ido Y, Lippincott-Schwartz J, Arias IM. Regulation of bile canalicular network formation and maintenance by AMP-activated protein kinase and LKB1. J Cell Sci. 2010;123(Pt 19):3294-3302. doi: 10.1242/jcs.068098
- Wang T, Yanger K, Stanger BZ, Cassio D, Bi E. Cytokinesis defines a spatial landmark for hepatocyte polarization and apical lumen formation. J Cell Sci. 2014;127(Pt 11): 2483-2492. doi: 10.1242/jcs.139923
- Zhang Y, De Mets R, Monzel C, et al. Biomimetic niches reveal the minimal cues to trigger apical lumen formation in single hepatocytes. Nat Mater. 2020;19(9):1026-1035. doi: 10.1038/s41563-020-0662-3
- He L, Pu W, Liu X, et al. Proliferation tracing reveals regional hepatocyte generation in liver homeostasis and repair. Science. 2021;371(6532):eabc4346. doi: 10.1126/science.abc4346
- Wei Y, Wang YG, Jia Y, et al. Liver homeostasis is maintained by midlobular zone 2 hepatocytes. Science. 2021;371(6532):eabb1625. doi: 10.1126/science.abb1625
- MacParland SA, Liu JC, Ma X-Z, et al. Single cell RNA sequencing of human liver reveals distinct intrahepatic macrophage populations. Nat Commun. 2018;” 9(1):4383. doi: 10.1038/s41467-018-06318-7
- He S, Luo Y, Ma W, et al. Endothelial POFUT1 controls injury-induced liver fibrosis by repressing fibrinogen synthesis. J Hepatol. 2024;81(1):135-148. doi: 10.1016/j.jhep.2024.02.032
- Shetty S, Lalor PF, Adams DH. Liver sinusoidal endothelial cells — gatekeepers of hepatic immunity. Nat Rev Gastroenterol Hepatol. 2018;15(9):555-567. doi: 10.1038/s41575-018-0020-y
- Gracia-Sancho J, Caparrós E, Fernández-Iglesias A, Francés R. Role of liver sinusoidal endothelial cells in liver diseases. Nat Rev Gastroenterol Hepatol. 2021;18(6):411-431. doi: 10.1038/s41575-020-00411-3
- Formes H, Bernardes JP, Mann A, et al. The gut microbiota instructs the hepatic endothelial cell transcriptome. iScience. 2021;24(10):103092. doi: 10.1016/j.isci.2021.103092
- Banales JM, Huebert RC, Karlsen T, Strazzabosco M, LaRusso NF, Gores GJ. Cholangiocyte pathobiology. Nat Rev Gastroenterol Hepatol. 2019;16(5):269-281. doi: 10.1038/s41575-019-0125-y
- Tabibian JH, Masyuk AI, Masyuk TV, O’Hara SP, LaRusso NF. Physiology of cholangiocytes. Compr Physiol. 2013;3(1): 541-565. doi: 10.1002/cphy.c120019
- Cervantes-Alvarez E, Wang Y, Collin de l’Hortet A, Guzman- Lepe J, Zhu J, Takeishi K. Current strategies to generate mature human induced pluripotent stem cells derived cholangiocytes and future applications. Organogenesis. 2017;13(1):1-15. doi: 10.1080/15476278.2016.1278133
- Sampaziotis F, Cardoso de Brito M, Madrigal P, et al. Cholangiocytes derived from human induced pluripotent stem cells for disease modeling and drug validation. Nat Biotechnol. 2015;33(8):845-852. doi: 10.1038/nbt.3275
- Ogawa M, Jiang J-X, Xia S, et al. Generation of functional ciliated cholangiocytes from human pluripotent stem cells. Nat Commun. 2021;12(1):6504. doi: 10.1038/s41467-021-26764-0
- Kamm DR, McCommis KS. Hepatic stellate cells in physiology and pathology. J Physiol. 2022;600(8): 1825-1837. doi: 10.1113/jp281061
- Tsuchida T, Friedman SL. Mechanisms of hepatic stellate cell activation. Nat Rev Gastroenterol Hepatol. 2017;14(7): 397-411. doi: 10.1038/nrgastro.2017.38
- Krenkel O, Tacke F. Liver macrophages in tissue homeostasis and disease. Nat Rev Immunol. 2017;17(5):306-321. doi: 10.1038/nri.2017.11
- Pollard JW. Trophic macrophages in development and disease. Nat Rev Immunol. 2009;9(4):259-270. doi: 10.1038/nri2528
- Zong Y, Stanger BZ. Molecular mechanisms of liver and bile duct development. Wiley Interdiscip Rev Dev Biol. 2012;1(5):643-655. doi: 10.1002/wdev.47
- Si-Tayeb K, Lemaigre FP, Duncan SA. Organogenesis and development of the liver. Dev Cell. 2010;18(2):175-189. doi: 10.1016/j.devcel.2010.01.011
- Gordillo M, Evans T, Gouon-Evans V. Orchestrating liver development. Development. 2015;142(12):2094-2108. doi: 10.1242/dev.114215
- Yang L, Li L-C, Lamaoqiezhong, et al. The contributions of mesoderm-derived cells in liver development. Semin Cell Dev Biol. 2019;92:63-76. doi: 10.1016/j.semcdb.2018.09.003
- Liang Y, Kaneko K, Xin B, et al. Temporal analyses of postnatal liver development and maturation by single-cell transcriptomics. Dev Cell. 2022;57(3):398-414.e5. doi: 10.1016/j.devcel.2022.01.004
- Alison M, Islam S, Lim S. Stem cells in liver regeneration, fibrosis and cancer: the good, the bad and the ugly. J Pathol. 2009;217(2):282-298. doi: 10.1002/path.2453
- Miyajima A, Tanaka M, Itoh T. Stem/Progenitor cells in liver development, homeostasis, regeneration, and reprogramming. Cell Stem Cell. 2014;14(5):561-574. doi: 10.1016/j.stem.2014.04.010
- Michalopoulos GK. Liver regeneration. J Cell Physiol. 2007;213(2):286-300. doi: 10.1002/jcp.21172
- Miyaoka Y, Ebato K, Kato H, Arakawa S, Shimizu S, Miyajima A. Hypertrophy and unconventional cell division of hepatocytes underlie liver regeneration. Curr Biol. 2012;22(13):1166-1175. doi: 10.1016/j.cub.2012.05.016
- Devarbhavi H, Asrani SK, Arab JP, Nartey YA, Pose E, Kamath PS. Global burden of liver disease: 2023 update. J Hepatol. 2023;79(2):516-537. doi: 10.1016/j.jhep.2023.03.017
- Bhatia SN, Underhill GH, Zaret KS, Fox IJ. Cell and tissue engineering for liver disease. Sci Transl Med. 2014;6(245):245sr2. doi: 10.1126/scitranslmed.3005975
- Sun Y, Chang J, Liu X, Liu C. Mortality trends of liver diseases in mainland China over three decades: an age-period-cohort analysis. BMJ Open. 2019;9(11):e029793. doi: 10.1136/bmjopen-2019-029793
- Mohiuddin MM, Singh AK, Scobie L, et al. Graft dysfunction in compassionate use of genetically engineered pig-to-human cardiac xenotransplantation: a case report. Lancet. 2023;402(10399):397-410. doi: 10.1016/S0140-6736(23)00775-4
- Mallapaty S, Kozlov M. First pig kidney transplant in a person: what it means for the future. Nature. 2024;628(8006):13-14. doi: 10.1038/d41586-024-00879-y
- Sun Z, Yuan X, Wu J, et al. Hepatocyte transplantation: the progress and the challenges. Hepatol Commun. 2023;7(10):e0266. doi: 10.1097/hc9.0000000000000266
- Struecker B, Raschzok N, Sauer IM. Liver support strategies: cutting-edge technologies. Nat Rev Gastroenterol Hepatol. 2014;11(3):166-176. doi: 10.1038/nrgastro.2013.204
- Millis JM, Losanoff JE. Technology Insight: liver support systems. Nat Clin Pract Gastroenterol Hepatol. 2005;2(9): 398-405. doi: 10.1038/ncpgasthep0254
- Krisper P, Stauber RE. Technology insight: artificial extracorporeal liver support—how does Prometheus® compare with MARS®? Nat Clin Pract Nephrol. 2007;3(5): 267-276. doi: 10.1038/ncpneph0466
- Strain AJ, Neuberger JM. A bioartificial liver--state of the art. Science. 2002;295(5557):1005-1009. doi: 10.1126/science.1068660
- Glorioso JM, Mao SA, Rodysill B, et al. Pivotal preclinical trial of the spheroid reservoir bioartificial liver. J Hepatol. 2015;63(2):388-398. doi: 10.1016/j.jhep.2015.03.021
- Li Y, Wu Q, Wang Y, et al. Novel spheroid reservoir bioartificial liver improves survival of nonhuman primates in a toxin-induced model of acute liver failure. Theranostics. 2018;8(20):5562-5574. doi: 10.7150/thno.26540
- Thompson J, Jones N, Al‐Khafaji A, et al. Extracorporeal cellular therapy (ELAD) in severe alcoholic hepatitis: a multinational, prospective, controlled, randomized trial. Liver Transpl. 2018;24(3):380-393. doi: 10.1002/lt.24986
- Chen S, Wang J, Ren H, et al. Hepatic spheroids derived from human induced pluripotent stem cells in bio-artificial liver rescue porcine acute liver failure. Cell Res. 2020; 30(1):95-97. doi: 10.1038/s41422-019-0261-5
- Li W-J, Zhu X-J, Yuan T-J, et al. An extracorporeal bioartificial liver embedded with 3D-layered human liver progenitor-like cells relieves acute liver failure in pigs. Sci Transl Med. 2020;12(551):eaba5146. doi: 10.1126/scitranslmed.aba5146
- Wang Y, Zheng Q, Sun Z, et al. Reversal of liver failure using a bioartificial liver device implanted with clinical-grade human-induced hepatocytes. Cell Stem Cell. 2023;30(5):617- 631.e8. doi: 10.1016/j.stem.2023.03.013
- Shi X-L, Gao Y, Yan Y, et al. Improved survival of porcine acute liver failure by a bioartificial liver device implanted with induced human functional hepatocytes. Cell Res. 2016;26(2):206-216. doi: 10.1038/cr.2016.6
- Hofer M, Lutolf MP. Engineering organoids. Nat Rev Mater. 2021;6(5):402-420. doi: 10.1038/s41578-021-00279-y
- Zhao Z, Chen X, Dowbaj AM, et al. Organoids. Nat Rev Methods Primers. 2022;2(1):94. doi: 10.1038/s43586-022-00174-y
- Rossi G, Manfrin A, Lutolf MP. Progress and potential in organoid research. Nat Rev Genet. 2018;19(11):671-687. doi: 10.1038/s41576-018-0051-9
- Harrison SP, Baumgarten SF, Verma R, Lunov O, Dejneka A, Sullivan GJ. Liver organoids: recent developments, limitations and potential. Front Med (Lausanne). 2021;8:574047. doi: 10.3389/fmed.2021.574047
- Hu H, Gehart H, Artegiani B, et al. Long-term expansion of functional mouse and human hepatocytes as 3D organoids. Cell. 2018;175(6):1591-1606.e19. doi: 10.1016/j.cell.2018.11.013
- Huch M, Dorrell C, Boj SF, et al. In vitro expansion of single Lgr5+ liver stem cells induced by Wnt-driven regeneration. Nature. 2013;494(7436):247-250. doi: 10.1038/nature11826
- Huch M, Gehart H, van Boxtel R, et al. Long-term culture of genome-stable bipotent stem cells from adult human liver. Cell. 2015;160(1):299-312. doi: 10.1016/j.cell.2014.11.050
- Vyas D, Baptista PM, Brovold M, et al. Self‐assembled liver organoids recapitulate hepatobiliary organogenesis in vitro. Hepatology. 2018;67(2):750-761. doi: 10.1002/hep.29483
- Takebe T, Sekine K, Enomura M, et al. Vascularized and functional human liver from an iPSC-derived organ bud transplant. Nature. 2013;499(7459):481-484. doi: 10.1038/nature12271
- Wu F, Wu D, Ren Y, et al. Generation of hepatobiliary organoids from human induced pluripotent stem cells. J Hepatol. 2019;70(6):1145-1158. doi: 10.1016/j.jhep.2018.12.028
- Sorrentino G, Rezakhani S, Yildiz E, et al. Mechano-modulatory synthetic niches for liver organoid derivation. Nat Commun. 2020;11(1):3416. doi: 10.1038/s41467-020-17161-0
- Zhang S, Xu G, Wu J, et al. Microphysiological constructs and systems: biofabrication tactics, biomimetic evaluation approaches, and biomedical applications. Small Methods. 2024;8(1):2300685. doi: 10.1002/smtd.202300685
- Zhang B, Korolj A, Lai BFL, Radisic M. Advances in organ-on-a-chip engineering. Nat Rev Mater. 2018;3(8):257-278. doi: 10.1038/s41578-018-0034-7
- Leung CM, de Haan P, Ronaldson-Bouchard K, et al. A guide to the organ-on-a-chip. Nat Rev Methods Primers. 2022;2(1):33. doi: 10.1038/s43586-022-00118-6
- Ingber DE. Human organs-on-chips for disease modelling, drug development and personalized medicine. Nat Rev Genet. 2022;23(8):467-491. doi: 10.1038/s41576-022-00466-9
- Low LA, Mummery C, Berridge BR, Austin CP, Tagle DA. Organs-on-chips: into the next decade. Nat Rev Drug Discov. 2021;20(5):345-361. doi: 10.1038/s41573-020-0079-3
- Bhatia SN, Ingber DE. Microfluidic organs-on-chips. Nat Biotechnol. 2014;32(8):760-772. doi: 10.1038/nbt.2989
- Moradi E, Jalili-Firoozinezhad S, Solati-Hashjin M. Microfluidic organ-on-a-chip models of human liver tissue. Acta Biomater. 2020;116:67-83. doi: 10.1016/j.actbio.2020.08.041
- Rennert K, Steinborn S, Gröger M, et al. A microfluidically perfused three dimensional human liver model. Biomaterials. 2015;71:119-131. doi: 10.1016/j.biomaterials.2015.08.043
- Siwczak F, Cseresnyes Z, Hassan MIA, et al. Human macrophage polarization determines bacterial persistence of Staphylococcus aureus in a liver-on-chip-based infection model. Biomaterials. 2022;287:121632. doi: 10.1016/j.biomaterials.2022.121632
- Jang K-J, Otieno MA, Ronxhi J, et al. Reproducing human and cross-species drug toxicities using a Liver-Chip. Sci Transl Med. 2019;11(517):eaax5516. doi: 10.1126/scitranslmed.aax5516
- Nawroth JC, Petropolis DB, Manatakis DV, et al. Modeling alcohol-associated liver disease in a human Liver-Chip. Cell Rep. 2021;36(3):109393. doi: 10.1016/j.celrep.2021.109393
- Ewart L, Apostolou A, Briggs SA, et al. Performance assessment and economic analysis of a human Liver-Chip for predictive toxicology. Commun Med. 2022;2(1):154. doi: 10.1038/s43856-022-00209-1
- Du K, Li S, Li C, et al. Modeling nonalcoholic fatty liver disease on a liver lobule chip with dual blood supply. Acta Biomater. 2021;134:228-239. doi: 10.1016/j.actbio.2021.07.013
- Hong G, Kim J, Oh H, et al. Production of multiple cell-laden microtissue spheroids with a biomimetic hepatic-lobule-like structure. Adv Mater. 2021;33(36):2102624. doi: 10.1002/adma.202102624
- Vunjak-Novakovic G, Ronaldson-Bouchard K, Radisic M. Organs-on-a-chip models for biological research. Cell. 2021;184(18):4597-4611. doi: 10.1016/j.cell.2021.08.005
- Ehrlich A, Duche D, Ouedraogo G, Nahmias Y. Challenges and opportunities in the design of liver-on-chip microdevices. Annu Rev Biomed Eng. 2019;21:219-239. doi: 10.1146/annurev-bioeng-060418-052305
- Moroni L, Burdick JA, Highley C, et al. Biofabrication strategies for 3D in vitro models and regenerative medicine. Nat Rev Mater. 2018;3(5):21-37. doi: 10.1038/s41578-018-0006-y
- Daly AC, Prendergast ME, Hughes AJ, Burdick JA. Bioprinting for the biologist. Cell. 2021;184(1):18-32. doi: 10.1016/j.cell.2020.12.002
- Morgan FLC, Moroni L, Baker MB. Dynamic bioinks to advance bioprinting. Adv Healthcare Mater. 2020;9(15):1901798. doi: 10.1002/adhm.201901798
- Chimene D, Lennox KK, Kaunas RR, Gaharwar AK. Advanced bioinks for 3D printing: a materials science perspective. Ann Biomed Eng. 2016;44(6):2090-2102. doi: 10.1007/s10439-016-1638-y
- Heinrich MA, Liu W, Jimenez A, et al. 3D bioprinting: from benches to translational applications. Small. 2019;15(23):1805510. doi: 10.1002/smll.201805510
- Malda J, Visser J, Melchels FP, et al. 25th anniversary article: engineering hydrogels for biofabrication. Adv Mater. 2013;25(36):5011-5028. doi: 10.1002/adma.201302042
- Lou J, Mooney DJ. Chemical strategies to engineer hydrogels for cell culture. Nat Rev Chem. 2022;6(10):726-744. doi: 10.1038/s41570-022-00420-7
- Chaudhuri O, Cooper-White J, Janmey PA, Mooney DJ, Shenoy VB. Effects of extracellular matrix viscoelasticity on cellular behaviour. Nature. 2020;584(7822): 535-546. doi: 10.1038/s41586-020-2612-2
- Rizwan M, Ling C, Guo C, et al. Viscoelastic notch signaling hydrogel induces liver bile duct organoid growth and morphogenesis. Adv Healthc Mater. 2022; 11(23):2200880. doi: 10.1002/adhm.202200880
- Murphy SV, Atala A. 3D bioprinting of tissues and organs. Nat Biotechnol. 2014;32(8):773-785. doi: 10.1038/nbt.2958
- Vasconcelos DP, Águas AP, Barbosa MA, Pelegrín P, Barbosa JN. The inflammasome in host response to biomaterials: bridging inflammation and tissue regeneration. Acta Biomater. 2019;83:1-12. doi: 10.1016/j.actbio.2018.09.056
- Levato R, Jungst T, Scheuring RG, Blunk T, Groll J, Malda J. From shape to function: the next step in bioprinting. Adv Mater. 2020;32(12):1906423. doi: 10.1002/adma.201906423
- Wang Y, Cui C-B, Yamauchi M, et al. Lineage restriction of human hepatic stem cells to mature fates is made efficient by tissue-specific biomatrix scaffolds. Hepatology. 2011;53(1):293-305. doi: 10.1002/hep.24012
- Nichol JW, Koshy ST, Bae H, Hwang CM, Yamanlar S, Khademhosseini A. Cell-laden microengineered gelatin methacrylate hydrogels. Biomaterials. 2010;31(21): 5536-5544. doi: 10.1016/j.biomaterials.2010.03.064
- Mouw JK, Ou G, Weaver VM. Extracellular matrix assembly: a multiscale deconstruction. Nat Rev Mol Cell Biol. 2014;15(12):771-785. doi: 10.1038/nrm3902
- Donderwinkel I, van Hest JCM, Cameron NR. Bio-inks for 3D bioprinting: recent advances and future prospects. Polymer Chem. 2017;8(31):4451-4471. doi: 10.1039/C7PY00826K
- Lee JW, Choi Y-J, Yong W-J, et al. Development of a 3D cell printed construct considering angiogenesis for liver tissue engineering. Biofabrication. 2016;8(1):015007. doi: 10.1088/1758-5090/8/1/015007
- Reizabal A, Costa CM, Pérez-Álvarez L, Vilas-Vilela JL, Lanceros-Méndez S. Silk fibroin as sustainable advanced material: material properties and characteristics, processing, and applications. Adv Funct Mater. 2023;33(3):2210764. doi: 10.1002/adfm.202210764
- Maity C, Das N. Alginate-based smart materials and their application: recent advances and perspectives. Top Curr Chem (Cham). 2021;380(1):3. doi: 10.1007/s41061-021-00360-8
- Spearman BS, Agrawal NK, Rubiano A, Simmons CS, Mobini S, Schmidt CE. Tunable methacrylated hyaluronic acid-based hydrogels as scaffolds for soft tissue engineering applications. J Biomed Mater Res A. 2020;108(2):279-291. doi: 10.1002/jbm.a.36814
- Ma X, Qu X, Zhu W, et al. Deterministically patterned biomimetic human iPSC-derived hepatic model via rapid 3D bioprinting. Proc Natl Acad Sci U S A. 2016;113(8): 2206-2211. doi: 10.1073/pnas.1524510113
- Baier Leach J, Bivens KA, Patrick CW, Jr., Schmidt CE. Photocrosslinked hyaluronic acid hydrogels: natural, biodegradable tissue engineering scaffolds. Biotechnol Bioeng. 2003;82(5):578-589. doi: 10.1002/bit.10605
- Zarrintaj P, Manouchehri S, Ahmadi Z, et al. Agarose-based biomaterials for tissue engineering. Carbohydr Polym. 2018;187:66-84. doi: 10.1016/j.carbpol.2018.01.060
- López-Marcial GR, Zeng AY, Osuna C, Dennis J, García JM, O’Connell GD. Agarose-based hydrogels as suitable bioprinting materials for tissue engineering. ACS Biomater Sci Eng. 2018;4(10):3610-3616. doi: 10.1021/acsbiomaterials.8b00903
- Hasebe Y, Okumura N, Koh T, et al. Formation of rat hepatocyte spheroids on agarose. Hepatol Res. 2005;32(2): 89-95. doi: 10.1016/j.hepres.2005.03.012
- Passaniti A, Kleinman HK, Martin GR. Matrigel: history/ background, uses, and future applications. J Cell Commun Signal. 2022;16(4):621-626. doi: 10.1007/s12079-021-00643-1
- Jiang S, Zhuang Y, Cai M, Wang X, Lin K. Decellularized extracellular matrix: a promising strategy for skin repair and regeneration. Eng Regenerat. 2023;4(4):357-374. doi: 10.1016/j.engreg.2023.05.001
- Deng B, Ma Y, Huang J, et al. Revitalizing liver function in mice with liver failure through transplantation of 3D-bioprinted liver with expanded primary hepatocytes. Sci Adv. 2024;10(23):eado1550. doi: 10.1126/sciadv.ado1550
- Lutolf MP, Lauer-Fields JL, Schmoekel HG, et al. Synthetic matrix metalloproteinase-sensitive hydrogels for the conduction of tissue regeneration: engineering cell-invasion characteristics. Proc Natl Acad Sci U S A. 2003;100(9): 5413-5418. doi: 10.1073/pnas.0737381100
- Kolesky DB, Homan KA, Skylar-Scott MA, Lewis JA. Three-dimensional bioprinting of thick vascularized tissues. Proc Natl Acad Sci U S A. 2016;113(12):3179-3184. doi: 10.1073/pnas.1521342113
- Gu Q, Tomaskovic-Crook E, Wallace GG, Crook JM. 3D bioprinting human induced pluripotent stem cell constructs for in situ cell proliferation and successive multilineage differentiation. Adv Healthc Mater. 2017;6(17):1700175. doi: 10.1002/adhm.201700175
- Andersson TB, Kanebratt KP, Kenna JG. The HepaRG cell line: a unique in vitro tool for understanding drug metabolism and toxicology in human. Expert Opin Drug Metab Toxicol. 2012;8(7):909-920. doi: 10.1517/17425255.2012.685159
- Cerec V, Glaise D, Garnier D, et al. Transdifferentiation of hepatocyte-like cells from the human hepatoma HepaRG cell line through bipotent progenitor. Hepatology. 2007;45(4):957-967. doi: 10.1002/hep.21536
- Gripon P, Rumin S, Urban S, et al. Infection of a human hepatoma cell line by hepatitis B virus. Proc Natl Acad Sci U S A. 2002;99(24):15655-15660. doi: 10.1073/pnas.232137699
- Higuchi Y, Kawai K, Yamazaki H, et al. The human hepatic cell line HepaRG as a possible cell source for the generation of humanized liver TK-NOG mice. Xenobiotica. 2014;44(2):146-153. doi: 10.3109/00498254.2013.836257
- Laurent V, Glaise D, Nübel T, Gilot D, Corlu A, Loyer P. Highly efficient SiRNA and gene transfer into hepatocyte-like HepaRG cells and primary human hepatocytes: new means for drug metabolism and toxicity studies. Methods Mol Biol. 2013;987:295-314. doi: 10.1007/978-1-62703-321-3_25
- Koike H, Iwasawa K, Ouchi R, et al. Engineering human hepato-biliary-pancreatic organoids from pluripotent stem cells. Nat Protoc. 2021;16(2):919-936. doi: 10.1038/s41596-020-00441-w
- Koike H, Iwasawa K, Ouchi R, et al. Modelling human hepato-biliary-pancreatic organogenesis from the foregut-midgut boundary. Nature. 2019;574(7776):112-116. doi: 10.1038/s41586-019-1598-0
- Hannan NR, Segeritz CP, Touboul T, Vallier L. Production of hepatocyte-like cells from human pluripotent stem cells. Nat Protoc. 2013;8(2):430-437. doi: 10.1038/nprot.2012.153
- Dao Thi VL, Wu X, Belote RL, et al. Stem cell-derived polarized hepatocytes. Nat Commun. 2020;11(1):1677. doi: 10.1038/s41467-020-15337-2
- Lee H, Chae S, Kim JY, et al. Cell-printed 3D liver-on-a-chip possessing a liver microenvironment and biliary system. Biofabrication. 2019;11(2):025001. doi: 10.1088/1758-5090/aaf9fa
- Liu Q, Mille LS, Villalobos C, et al. 3D-bioprinted cholangiocarcinoma-on-a-chip model for evaluating drug responses. Bio-Design Manuf. 2023;6(4):373-389. doi: 10.1007/s42242-022-00229-9
- Hafiz EOA, Bulutoglu B, Mansy SS, et al. Development of liver microtissues with functional biliary ductular network. Biotechnol Bioeng. 2021;118(1):17-29. doi: 10.1002/bit.27546
- Stevens KR, Scull MA, Ramanan V, et al. In situ expansion of engineered human liver tissue in a mouse model of chronic liver disease. Sci Transl Med. 2017;9(399):eaah5505. doi: 10.1126/scitranslmed.aah5505
- Grigoryan B, Paulsen SJ, Corbett DC, et al. Multivascular networks and functional intravascular topologies within biocompatible hydrogels. Science. 2019;364(6439): 458-464. doi: 10.1126/science.aav9750
- Peng X, Janićijević Ž, Lemm S, et al. Impact of viscosity on human hepatoma spheroids in soft core–shell microcapsules. Adv Healthc Mater. 2024;13(11):2302609. doi: 10.1002/adhm.202302609
- Foyt DA, Norman MDA, Yu TTL, Gentleman E. Exploiting advanced hydrogel technologies to address key challenges in regenerative medicine. Adv Healthc Mater. 2018;7(8):1700939. doi: 10.1002/adhm.201700939
- Bishop ES, Mostafa S, Pakvasa M, et al. 3-D bioprinting technologies in tissue engineering and regenerative medicine: current and future trends. Genes Dis. 2017;4(4):185-195. doi: 10.1016/j.gendis.2017.10.002
- Xu C, Zhang M, Huang Y, Ogale A, Fu J, Markwald RR. Study of droplet formation process during drop-on-demand inkjetting of living cell-Laden Bioink. Langmuir. 2014;30(30):9130-9138. doi: 10.1021/la501430x
- Matsusaki M, Sakaue K, Kadowaki K, Akashi M. Three-dimensional human tissue chips fabricated by rapid and automatic inkjet cell printing. Adv Healthc Mater. 2013;2(4):534-539. doi: 10.1002/adhm.201200299
- Jian H, Li X, Dong Q, Tian S, Bai S. In vitro construction of liver organoids with biomimetic lobule structure by a multicellular 3D bioprinting strategy. Cell Prolif. 2023;56(5):e13465. doi: 10.1111/cpr.13465
- Gu Z, Fu J, Lin H, He Y. Development of 3D bioprinting: from printing methods to biomedical applications. Asian J Pharm Sci. 2020;15(5):529-557. doi: 10.1016/j.ajps.2019.11.003
- Dou C, Perez V, Qu J, Tsin A, Xu B, Li J. A State-of-the-Art review of laser-assisted bioprinting and its future research trends. ChemBioEng Rev. 2021;8(5):517-534. doi: 10.1002/cben.202000037
- Zhu W, Qu X, Zhu J, et al. Direct 3D bioprinting of prevascularized tissue constructs with complex microarchitecture. Biomaterials. 2017;124:106-115. doi: 10.1016/j.biomaterials.2017.01.042
- Wang M, Li W, Hao J, et al. Molecularly cleavable bioinks facilitate high-performance digital light processing-based bioprinting of functional volumetric soft tissues. Nat Commun. 2022;13(1):3317. doi: 10.1038/s41467-022-31002-2
- Jing S, Lian L, Hou Y, et al. Advances in volumetric bioprinting. Biofabrication. 2024;16(1):012004. doi: 10.1088/1758-5090/ad0978
- Bernal PN, Bouwmeester M, Madrid-Wolff J, et al. Volumetric bioprinting of organoids and optically tuned hydrogels to build liver-like metabolic biofactories. Adv Mater. 2022;34(15):2110054. doi: 10.1002/adma.202110054
- Miller JS, Stevens KR, Yang MT, et al. Rapid casting of patterned vascular networks for perfusable engineered three-dimensional tissues. Nat Mater. 2012;11(9):768-774. doi: 10.1038/nmat3357
- Liu X, Wang X, Zhang L, et al. 3D liver tissue model with branched vascular networks by multimaterial bioprinting. Adv Healthc Mater. 2021;10(23):2101405. doi: 10.1002/adhm.202101405
- Yang H, Sun L, Pang Y, et al. Three-dimensional bioprinted hepatorganoids prolong survival of mice with liver failure. Gut. 2021;70(3):567-574. doi: 10.1136/gutjnl-2019-319960
- McCormack A, Highley CB, Leslie NR, Melchels FPW. 3D printing in suspension baths: keeping the promises of bioprinting afloat. Trends Biotechnol. 2020;38(6):584-593. doi: 10.1016/j.tibtech.2019.12.020
- Lee A, Hudson AR, Shiwarski DJ, et al. 3D bioprinting of collagen to rebuild components of the human heart. Science. 2019;365(6452):482-487. doi: 10.1126/science.aav9051
- Hinton TJ, Jallerat Q, Palchesko RN, et al. Three-dimensional printing of complex biological structures by freeform reversible embedding of suspended hydrogels. Sci Adv. 2015;1(9):e1500758. doi: 10.1126/sciadv.1500758
- Fang Y, Ji M, Yang Y, et al. 3D printing of vascularized hepatic tissues with a high cell density and heterogeneous microenvironment. Biofabrication. 2023;15(4):045004. doi: 10.1088/1758-5090/ace5e0
- Taymour R, Chicaiza-Cabezas NA, Gelinsky M, Lode A. Core–shell bioprinting of vascularized in vitro liver sinusoid models. Biofabrication. 2022;14(4):045019. doi: 10.1088/1758-5090/ac9019
- Wang H, Liu X, Gu Q, Zheng X. Vascularized organ bioprinting: from strategy to paradigm. Cell Prolif. 2023;56(5):e13453. doi: 10.1111/cpr.13453
- Kinstlinger IS, Saxton SH, Calderon GA, et al. Generation of model tissues with dendritic vascular networks via sacrificial laser-sintered carbohydrate templates. Nat Biomed Eng. 2020;4(9):916-932. doi: 10.1038/s41551-020-0566-1
- O’Connor C, Brady E, Zheng Y, Moore E, Stevens KR. Engineering the multiscale complexity of vascular networks. Nat Rev Mater. 2022;7(9):702-716. doi: 10.1038/s41578-022-00447-8
- Zong Y, Stanger BZ. Molecular mechanisms of bile duct development. Int J Biochem Cell Biol. 2011;43(2): 257-264. doi: 10.1016/j.biocel.2010.06.020
- Raynaud P, Carpentier R, Antoniou A, Lemaigre FP. Biliary differentiation and bile duct morphogenesis in development and disease. Int J Biochem Cell Biol. 2011;43(2): 245-256. doi: 10.1016/j.biocel.2009.07.020
- Justin AW, Saeb-Parsy K, Markaki AE, Vallier L, Sampaziotis F. Advances in the generation of bioengineered bile ducts. Biochim Biophys Acta Mol Basis Dis. 2018;1864(4PtB): 1532-1538. doi: 10.1016/j.bbadis.2017.10.034
- Wang Z, Faria J, Penning LC, Masereeuw R, Spee B. Tissue-engineered bile ducts for disease modeling and therapy. Tissue Eng Part C Methods. 2021;27(2):59-76. doi: 10.1089/ten.TEC.2020.0283
- Squires RH, Ng V, Romero R, et al. Evaluation of the pediatric patient for liver transplantation. J Pediatr Gastroenterol Nutr. 2014;59(1):112-131. doi: 10.1097/MPG.0000000000000431
- Brevini T, Tysoe OC, Sampaziotis F. Tissue engineering of the biliary tract and modelling of cholestatic disorders. J Hepatol. 2020;73(4):918-932. doi: 10.1016/j.jhep.2020.05.049
- Sun Q, Shen Z, Liang X, et al. Progress and current limitations of materials for artificial bile duct engineering. Materials. 2021;14(23):7468. doi: 10.3390/ma14237468
- Lewis PL, Su J, Yan M, et al. Complex bile duct network formation within liver decellularized extracellular matrix hydrogels. Sci Rep. 2018;8(1):12220. doi: 10.1038/s41598-018-30433-6
- Sampaziotis F, Muraro D, Tysoe OC, et al. Cholangiocyte organoids can repair bile ducts after transplantation in the human liver. Science. 2021;371(6531):839-846. doi: 10.1126/science.aaz6964
- Sampaziotis F, Justin AW, Tysoe OC, et al. Reconstruction of the mouse extrahepatic biliary tree using primary human extrahepatic cholangiocyte organoids. Nat Med. 2017;23(8):954-963. doi: 10.1038/nm.4360
- Roos FJM, van Tienderen GS, Wu H, et al. Human branching cholangiocyte organoids recapitulate functional bile duct formation. Cell Stem Cell. 2022;29(5):776-794.e13. doi: 10.1016/j.stem.2022.04.011
- Du Y, de Jong IEM, Gupta K, et al. Human vascularized bile duct-on-a chip: a multi-cellular micro-physiological system for studying cholestatic liver disease. Biofabrication. 2023;16(1):015004. doi: 10.1088/1758-5090/ad0261
- Du Y, Khandekar G, Llewellyn J, Polacheck W, Chen CS, Wells RG. A bile duct-on-a-chip with organ-level functions. Hepatology. 2020;71(4):1350-1363. doi: 10.1002/hep.30918
- Tanimizu N, Ichinohe N, Sasaki Y, et al. Generation of functional liver organoids on combining hepatocytes and cholangiocytes with hepatobiliary connections ex vivo. Nature Commun. 2021;12(1):3390. doi: 10.1038/s41467-021-23575-1
- Loterie D, Delrot P, Moser C. High-resolution tomographic volumetric additive manufacturing. Nat Commun. 2020;11(1):852. doi: 10.1038/s41467-020-14630-4
- Kelly BE, Bhattacharya I, Heidari H, Shusteff M, Spadaccini CM, Taylor HK. Volumetric additive manufacturing via tomographic reconstruction. Science. 2019;363(6431): 1075-1079. doi: 10.1126/science.aau7114
- Bernal PN, Delrot P, Loterie D, et al. Volumetric bioprinting of complex living-tissue constructs within seconds. Adv Mater. 2019;31(42):e1904209. doi: 10.1002/adma.201904209
- Cai H, Wu Z, Ao Z, et al. Trapping cell spheroids and organoids using digital acoustofluidics. Biofabrication. 2020;12(3):035025. doi: 10.1088/1758-5090/ab9582
- Johnson K, Melchert D, Gianola DS, Begley M, Ray TR. Recent progress in acoustic field-assisted 3D-printing of functional composite materials. MRS Adv. 2021;6(25): 636-643. doi: 10.1557/s43580-021-00090-5
- Foresti D, Kroll KT, Amissah R, et al. Acoustophoretic printing. Sci Adv. 2018;4(8):eaat1659. doi: 10.1126/sciadv.aat1659
- Jentsch S, Nasehi R, Kuckelkorn C, Gundert B, Aveic S, Fischer H. Multiscale 3D bioprinting by nozzle-free acoustic droplet ejection. Small Methods. 2021;5(6):2000971. doi: 10.1002/smtd.202000971
- Guo Q, Su X, Zhang X, Shao M, Yu H, Li D. A review on acoustic droplet ejection technology and system. Soft Matter. 2021;17(11):3010-3021. doi: 10.1039/D0SM02193H
- Liu C, Pandit PP, Parsons C, Khan F, Hu Y. Acoustic field-assisted inkjet-based additive manufacturing of carbon fiber-reinforced polydimethylsiloxane composites. J Manuf Process. 2022;80:87-94. doi: 10.1016/j.jmapro.2022.05.059
- Wadsworth P, Nelson I, Porter DL, Raeymaekers B, Naleway SE. Manufacturing bioinspired flexible materials using ultrasound directed self-assembly and 3D printing. Mater Design. 2020;185:108243. doi: 10.1016/j.matdes.2019.108243
- Chen K, Jiang E, Wei X, et al. The acoustic droplet printing of functional tumor microenvironments. Lab Chip. 2021;21(8):1604-1612. doi: 10.1039/D1LC00003A
- Lei Y, Hu H. SAW-driven droplet jetting technology in microfluidic: a review. Biomicrofluidics. 2020;14(6): 061505. doi: 10.1063/5.0014768
- Kim SH, Seo YB, Yeon YK, et al. 4D-bioprinted silk hydrogels for tissue engineering. Biomaterials. 2020;260: 120281. doi: 10.1016/j.biomaterials.2020.120281
- Li K, Huang W, Guo H, et al. Advancements in robotic arm-based 3D bioprinting for biomedical applications. Life Med. 2023;2(6):lnad046 doi: 10.1093/lifemedi/lnad046
- An J, Zhang S, Wu J, et al. Assessing bioartificial organ function: the 3P model framework and its validation. Lab Chip. 2024;24(6):1586-1601. doi: 10.1039/D3LC01020A