A study on the synergistic chondro-inductive effects of collagen II and its high-resolution 3D-printed scaffolds
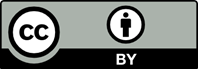
Collagen II is the most essential component of the cartilage-specific extracellular matrix (ECM). However, research on collagen II-based scaffold fabrication, structural optimization, and correlation to the chondrogenic activities of stem cells is currently limited, presumably due to challenges related to its hydrogel processability. Existing collagen II-containing scaffolds are mainly produced by freeze-drying and exhibit limited properties, such as pore interconnectivity and tortuosity. Additionally, the chondro-inductive capability of collagen II composition and its underlying mechanism remains unclear, warranting further research. In this study, we addressed the aforementioned issues by investigating and enhancing the rheological properties of collagen II-based hydrogel, resulting in a high printing resolution exceeding 150 μm. The collagen II composition reportedly facilitated the condensation and chondrogenic activities of mesenchymal stem cells (MSCs) compared to gelatin. Moreover, high-resolution collagen II-based scaffolds promoted cell proliferation and chondrogenic differentiation to a higher degree. Therefore, we optimized the compositional and structural characteristics of collagen II-based scaffolds for enhancing chondrogenic activities. We anticipate that this study will broaden our understanding of collagen II-based scaffold designs and condition optimizations for cartilage tissue engineering.
- Petitjean N, Canadas P, Royer P, Noël D, Le Floc’h S. Cartilage biomechanics: from the basic facts to the challenges of tissue engineering. J Biomed Mater Res A. 2023;111(7):1067-1089. doi: 10.1002/jbm.a.37478
- Xu X, Xu L, Xia J, Wen C, Liang Y, Zhang Y. Harnessing knee joint resident mesenchymal stem cells in cartilage tissue engineering. Acta Biomater. 2023;168:372-387. doi: 10.1016/j.actbio.2023.07.024
- Stampoultzis T, Karami P, Pioletti DP. Thoughts on cartilage tissue engineering: a 21st century perspective. Curr Res Transl Med. 2021;69(3):103299. doi: 10.1016/j.retram.2021.103299
- Nguyen TPT, Li F, Shrestha S, et al. Cell-laden injectable microgels: current status and future prospects for cartilage regeneration. Biomaterials. 2021;279:121214. doi: 10.1016/j.biomaterials.2021.121214
- Yang Z, Li H, Yuan Z, et al. Endogenous cell recruitment strategy for articular cartilage regeneration. Acta Biomater. 2020;114:31-52. doi: 10.1016/j.actbio.2020.07.008
- Wu Z, Korntner SH, Mullen AM, Zeugolis DI. Collagen type II: from biosynthesis to advanced biomaterials for cartilage engineering. Biomater Biosyst. 2021;4:100030. doi: 10.1016/j.bbiosy.2021.100030
- Huang H, Ayariga J, Ning H, Nyairo E, Dean D. Freeze-printing of pectin/alginate scaffolds with high resolution, overhang structures and interconnected porous network. Addit Manuf. 2021;46:102120. doi: 10.1016/j.addma.2021.102120
- Statham P, Jones E, Jennings LM, Fermor HL. Reproducing the biomechanical environment of the chondrocyte for cartilage tissue engineering. Tissue Eng Part B Rev. 2021;28(2):405-420. doi: 10.1089/ten.teb.2020.0373
- Bielajew BJ, Donahue RP, Lamkin EK, Hu JC, Hascall VC, Athanasiou KA. Proteomic, mechanical, and biochemical characterization of cartilage development. Acta Biomater. 2022;143:52-62. doi: 10.1016/j.actbio.2022.02.037
- Sani M, Hosseinie R, Latifi M, et al. Engineered artificial articular cartilage made of decellularized extracellular matrix by mechanical and IGF-1 stimulation. Biomater Adv. 2022;139:213019. doi: 10.1016/j.bioadv.2022.213019
- Peng Z, Sun H, Bunpetch V, et al. The regulation of cartilage extracellular matrix homeostasis in joint cartilage degeneration and regeneration. Biomaterials. 2021;268:120555. doi: 10.1016/j.biomaterials.2020.120555
- Sarrigiannidis SO, Rey JM, Dobre O, González-García C, Dalby MJ, Salmeron-Sanchez M. A tough act to follow: collagen hydrogel modifications to improve mechanical and growth factor loading capabilities. Mater Today Bio. 2021;10:100098. doi: 10.1016/j.mtbio.2021.100098
- Kilmer CE, Battistoni CM, Cox A, Breur GJ, Panitch A, Liu JC. Collagen Type I and II blend hydrogel with autologous mesenchymal stem cells as a scaffold for articular cartilage defect repair. ACS Biomater Sci Eng. 2020;6(6):3464-3476. doi: 10.1021/acsbiomaterials.9b01939
- Cámara-Torres M, Sinha R, Mota C, Moroni L. Improving cell distribution on 3D additive manufactured scaffolds through engineered seeding media density and viscosity. Acta Biomater. 2020;101(1):183-195. doi: 10.1016/j.actbio.2019.11.020
- Pfeiffer E, Vickers SM, Frank E, Grodzinsky AJ, Spector M. The effects of glycosaminoglycan content on the compressive modulus of cartilage engineered in type II collagen scaffolds. Osteoarthritis Cartilage. 2008;16(10):1237-1244. doi: 10.1016/j.joca.2008.02.014
- Yang K, Sun J, Dan W, et al. Photo-crosslinked mono-component type II collagen hydrogel as matrix to induce chondrogenic diffrentiation of bone marrow mesenchymal stem cells. J Mater Chem B. 2017;5:8707-8718. doi: 10.1039/C7TB02348K
- Piperigkou Z, Bainantzou D, Makri N, et al. Enhancement of mesenchymal stem cells’ chondrogenic potential by type II collagen-based bioscaffolds. Mol Biol Rep. 2023;50(6): 5125-5135. doi: 10.1007/s11033-023-08461-x
- Kato YP, Christiansen DL, Hahn RA, Shieh S-J, Goldstein JD, Silver FH. Mechanical properties of collagen fibres: a comparison of reconstituted and rat tail tendon fibres. Biomaterials. 1989;10(1):38-42. doi: 10.1016/0142-9612(89)90007-0
- Sun Y-L, Luo Z-P, Fertala A, An K-N. Stretching type II collagen with optical tweezers. J Biomech. 2004;37(11): 1665-1669. doi: 10.1016/j.jbiomech.2004.02.028
- Hu X, Jin M, Sun K, et al. Type II collagen scaffolds repair critical-sized osteochondral defects under induced conditions of osteoarthritis in rat knee joints via inhibiting TGF-β-Smad1/5/8 signaling pathway. Bioactive Mater. 2024;35:416-428. doi: 10.1016/j.bioactmat.2024.02.008
- Tamaddon M, Burrows M, Ferreira S, et al. Monomeric, porous type II collagen scaffolds promote chondrogenic differentiation of human bone marrow mesenchymal stem cells in vitro. Sci Rep. 2017;7:43519. doi: 10.1038/srep43519
- Intini C, Lemoine M, Hodgkinson T, Casey S, Gleeson J, O’Brien F. A highly porous type II collagen containing scaffold for the treatment of cartilage defects enhances MSC chondrogenesis and early cartilaginous matrix deposition. Biomater Sci. 2022;10:970-983. doi: 10.1039/D1BM01417J
- Ko CS, Huang J-P, Huang C-W, Chu IM. Type II collagen-chondroitin sulfate-hyaluronan scaffold cross-linked by genipin for cartilage tissue engineering. J Biosci Bioeng. 2009;107(2):177-182. doi: 10.1016/j.jbiosc.2008.09.020
- Rustom LE, Poellmann MJ, Johnson AJW. Mineralization in micropores of calcium phosphate scaffolds. Acta Biomater. 2019;83:435-455. doi: 10.1016/j.actbio.2018.11.003
- Suo H, Zhang J, Xu M, Wang L. Low-temperature 3D printing of collagen and chitosan composite for tissue engineering. Mater Sci Eng C. 2021;123:111963. doi: 10.1016/j.msec.2021.111963
- Bhardwaj D, Singhmar R, Garg M, et al. Designing advanced hydrogel inks with direct ink writing based 3D printability for engineered biostructures. Eur Polym J. 2024;205:112736. doi: 10.1016/j.eurpolymj.2023.112736
- Gupta D, Singh AK, Dravid A, Bellare J. Multiscale porosity in compressible cryogenically 3D printed gels for bone tissue engineering. ACS Appl Mater Interfaces. 2019;11(22): 20437-20452. doi: 10.1021/acsami.9b05460
- Gupta D, Vashisth P, Bellare J. Multiscale porosity in a 3D printed gellan–gelatin composite for bone tissue engineering. Biomed Mater. 2021;16(3):034103. doi: 10.1088/1748-605x/abf1a7
- Livak KJ, Schmittgen TD. Analysis of relative gene expression data using real-time quantitative PCR and the 2− ΔΔCT method. Methods. 2001;25(4):402-408. doi: 10.1006/meth.2001.1262
- Townsend JM, Beck EC, Gehrke SH, Berkland CJ, Detamore MS. Flow behavior prior to crosslinking: the need for precursor rheology for placement of hydrogels in medical applications and for 3D bioprinting. Prog Polymer Sci. 2019;91:126-140. doi: 10.1016/j.progpolymsci.2019.01.003
- Shi L, Hu Y, Ullah MW, et al. Cryogenic free-form extrusion bioprinting of decellularized small intestinal submucosa for potential applications in skin tissue engineering. Biofabrication. 2019;11(3):035023.
- Hu Y, Wu B, Xiong Y, et al. Cryogenic 3D printed hydrogel scaffolds loading exosomes accelerate diabetic wound healing. Chem Eng J. 2021;426:130634. doi: 10.1016/j.cej.2021.130634
- Yang L, Jin S, Shi L, et al. Cryogenically 3D printed biomimetic scaffolds containing decellularized small intestinal submucosa and Sr2+/Fe3+ co-substituted hydroxyapatite for bone tissue engineering. Chem Eng J. 2022;431:133459. doi: 10.1016/j.cej.2021.133459
- Lu Z, Zandieh Doulabi B, Huang C, Bank R, Helder M. Collagen type II enhances chondrogenesis in adipose tissue– derived stem cells by affecting cell shape. Tissue Eng Part A. 2010;16(1):81-90. doi: 10.1089/ten.TEA.2009.0222
- Li R, Xu J, Wong DSH, Li J, Zhao P, Bian L. Self-assembled N-cadherin mimetic peptide hydrogels promote the chondrogenesis of mesenchymal stem cells through inhibition of canonical Wnt/β-catenin signaling. Biomaterials. 2017;145:33-43. doi: 10.1016/j.biomaterials.2017.08.031
- Ke W, Ma L, Wang B, et al. N-cadherin mimetic hydrogel enhances MSC chondrogenesis through cell metabolism. Acta Biomater. 2022;150:83-95. doi: 10.1016/j.actbio.2022.07.050
- Wang Y, Xiao Y, Long S, Fan Y, Zhang X. Role of N-Cadherin in a niche-mimicking microenvironment for chondrogenesis of mesenchymal stem cells in vitro. ACS Biomater Sci Eng. 2020;6(6):3491-3501. doi: 10.1021/acsbiomaterials.0c00149
- Eren Cimenci C, Kurtulus GU, Caliskan OS, Guler MO, Tekinay AB. N-cadherin mimetic peptide nanofiber system induces chondrogenic differentiation of mesenchymal stem cells. Bioconjugate Chem. 2019;30(9):2417-2426. doi: 10.1021/acs.bioconjchem.9b00514
- Choi B, Kim S, Lin B, Wu BM, Lee M. Cartilaginous extracellular matrix-modified chitosan hydrogels for cartilage tissue engineering. ACS Appl Mater Interfaces. 2014;6(22):20110-20121. doi: 10.1021/am505723k
- Ren X, Wang F, Chen C, Gong X, Yin L, Yang L. Engineering zonal cartilage through bioprinting collagen type II hydrogel constructs with biomimetic chondrocyte density gradient. BMC Musculoskeletal Disord. 2016;17(1):301. doi: 10.1186/s12891-016-1130-8
- Gibney R, Ferraris E. Bioprinting of collagen Type I and II via aerosol jet printing for the replication of dense collagenous tissues. original research. Front Bioeng Biotechnol. 2021;9: 786945. doi: 10.3389/fbioe.2021.786945
- Eviana Putri NR, Wang X, Chen Y, Li X, Kawazoe N, Chen G. Preparation of PLGA-collagen hybrid scaffolds with controlled pore structures for cartilage tissue engineering. Prog Nat Sci Mater Int. 2020;30(5):642-650. doi: 10.1016/j.pnsc.2020.07.003
- Cheng A, Schwartz Z, Kahn A, et al. Advances in porous scaffold design for bone and cartilage tissue engineering and regeneration. Tissue Eng Part B Rev. 2019;25(1):14-29. doi: 10.1089/ten.teb.2018.0119
- Zhang Q, Lu H, Kawazoe N, Chen G. Preparation of collagen scaffolds with controlled pore structures and improved mechanical property for cartilage tissue engineering. J Bioactive Compat Polym. 2013;28(5):426-438. doi: 10.1177/0883911513494620
- Harley BAC, Kim H-D, Zaman MH, Yannas IV, Lauffenburger DA, Gibson LJ. Microarchitecture of three-dimensional scaffolds influences cell migration behavior via junction interactions. Biophys J. 2008;95(8):4013-4024. doi: 10.1529/biophysj.107.122598
- Bai Y, Gong X, Dou C, Cao Z, Dong S. Redox control of chondrocyte differentiation and chondrogenesis. Free Radic Biol Med. 2019;132:83-89. doi: 10.1016/j.freeradbiomed.2018.10.443
- Di Luca A, Szlazak K, Lorenzo-Moldero I, et al. Influencing chondrogenic differentiation of human mesenchymal stromal cells in scaffolds displaying a structural gradient in pore size. Acta Biomater. 2016;36:210-219. doi: 10.1016/j.actbio.2016.03.014
- Sun Y, Wu Q, Zhang Y, Dai K, Wei Y. 3D-bioprinted gradient-structured scaffold generates anisotropic cartilage with vascularization by pore-size-dependent activation of HIF1α/FAK signaling axis. Nanomedicine. 2021;37:102426. doi: 10.1016/j.nano.2021.102426
- Luo C, Wang C, Wu X, et al. Influence of porous tantalum scaffold pore size on osteogenesis and osteointegration: a comprehensive study based on 3D-printing technology. Mater Sci Eng C Mater Biol Appl. 2021;129:112382. doi: 10.1016/j.msec.2021.112382
- Mahzoon S, Detamore MS. Chondroinductive peptides: drawing inspirations from cell–matrix interactions. Tissue Eng Part B Rev. 2019;25(3):249-257. doi: 10.1089/ten.teb.2018.0003
- Asgarpour K, Shojaei Z, Amiri F, et al. Exosomal microRNAs derived from mesenchymal stem cells: cell-to-cell messages. Cell Commun Signal. 2020;18(1):149. doi: 10.1186/s12964-020-00650-6
- Kwon H, Paschos NK, Hu JC, Athanasiou K. Articular cartilage tissue engineering: the role of signaling molecules. Cell Mol Life Sci. 2016;73(6):1173-1194. doi: 10.1007/s00018-015-2115-8
- Chen L, Liu J, Guan M, Zhou T, Duan X, Xiang Z. Growth factor and its polymer scaffold-based delivery system for cartilage tissue engineering. Int J Nanomed. 2020;15: 6097-6111. doi: 10.2147/IJN.S249829
- Lee C-R, Grodzinsky A, Spector M. Biosynthetic response of passaged chondrocytes in a type II collagen scaffold to mechanical compression. J Biomed Mater Res A. 2003;64(3):560-569. doi: 10.1002/jbm.a.10443
- Li Y, Liu Y, Li R, et al. Collagen-based biomaterials for bone tissue engineering. Mater Design. 2021;210:110049. doi: 10.1016/j.matdes.2021.110049
- Shields KJ, Beckman MJ, Bowlin GL, Wayne JS. Mechanical properties and cellular proliferation of electrospun collagen type II. Tissue Eng. 2004;10(9-10):1510-1517. doi: 10.1089/ten.2004.10.1510
- Jang J-W, Min K-E, Kim C, Shin J, Lee J, Yi S. Review: scaffold characteristics, fabrication methods, and biomaterials for the bone tissue engineering. Int J Precision Eng Manuf. 2023;24(3):511-529. doi: 10.1007/s12541-022-00755-7
- Koo E, Choi E-J, Lee J, Kim H, Kim G, Do S-H. 3D printed cell-laden collagen and hybrid scaffolds for in vivo articular cartilage tissue regeneration. J Ind Eng Chem. 2018;66: 343-355. doi: 10.1016/j.jiec.2018.05.049
- Maihemuti A, Zhang H, Lin X, et al. 3D-printed fish gelatin scaffolds for cartilage tissue engineering. Bioact Mater. 2023;26:77-87. doi: 10.1016/j.bioactmat.2023.02.007
- Nocera AD, Comín R, Salvatierra NA, Cid MP. Development of 3D printed fibrillar collagen scaffold for tissue engineering. Biomed Microdevices. 2018;20(2):26. doi: 10.1007/s10544-018-0270-z
- Marques CF, Diogo GS, Pina S, Oliveira JM, Silva TH, Reis RL. Collagen-based bioinks for hard tissue engineering applications: a comprehensive review. J Mater Sci Mater Med. 2019;30(3):32. doi: 10.1007/s10856-019-6234-x
- Diao J, OuYang J, Deng T, et al. 3D-plotted beta-tricalcium phosphate scaffolds with smaller pore sizes improve in vivo bone regeneration and biomechanical properties in a critical-sized calvarial defect rat model. Adv Healthc Mater. 2018;7(17):e1800441. doi: 10.1002/adhm.201800441
- Cacopardo L, Guazzelli N, Nossa R, Mattei G, Ahluwalia A. Engineering hydrogel viscoelasticity. J Mech Behav Biomed Mater. 2019;89:162-167. doi: 10.1016/j.jmbbm.2018.09.031
- Vining KH, Stafford A, Mooney DJ. Sequential modes of crosslinking tune viscoelasticity of cell-instructive hydrogels. Biomaterials. 2019;188:187-197. doi: 10.1016/j.biomaterials.2018.10.013
- Ma Y, Han T, Yang Q, et al. Viscoelastic cell microenvironment: hydrogel-based strategy for recapitulating dynamic ECM mechanics. Adv Funct Mater. 2021;31(24):2100848. doi: 10.1002/adfm.202100848
- Oh SH, Lee JH. Hydrophilization of synthetic biodegradable polymer scaffolds for improved cell/tissue compatibility. Biomed Mater. 2013;8(1):014101. doi: 10.1088/1748-6041/8/1/014101
- Wang W, Caetano G, Ambler WS, et al. Enhancing the hydrophilicity and cell attachment of 3D printed PCL/ Graphene scaffolds for bone tissue engineering. Materials (Basel). 2016;9(12):992. doi: 10.3390/ma9120992
- Chollet C, Lazare S, Guillemot F, Durrieu MC. Impact of RGD micro-patterns on cell adhesion. Colloids Surf B Biointerfaces. 2010;75(1):107-114. doi: 10.1016/j.colsurfb.2009.08.024
- Wu S-C, Chang W-H, Dong G-C, Chen K-Y, Chen Y-S, Yao C-H. Cell adhesion and proliferation enhancement by gelatin nanofiber scaffolds. J Bioactive Compatible Polyms. 2011;26(6):565-577. doi: 10.1177/0883911511423563
- Steward AJ, Liu Y, Wagner DR. Engineering cell attachments to scaffolds in cartilage tissue engineering. JOM. 2011;63(4):74-82. doi: 10.1007/s11837-011-0062-x
- Padhi A, Nain AS. ECM in Differentiation: a review of matrix structure, composition and mechanical properties. Ann Biomed Eng. 2020;48(3):1071-1089. doi: 10.1007/s10439-019-02337-7
- Ross JJ, Tranquillo RT. ECM gene expression correlates with in vitro tissue growth and development in fibrin gel remodeled by neonatal smooth muscle cells. Matrix Biol. 2003;22(6):477-490. doi: 10.1016/S0945-053X(03)00078-7
- Kjaer M. Role of extracellular matrix in adaptation of tendon and skeletal muscle to mechanical loading. Physiol Rev. 2004;84(2):649-698. doi: 10.1152/physrev.00031.2003
- Ho-Shui-Ling A, Bolander J, Rustom LE, Johnson AW, Luyten FP, Picart C. Bone regeneration strategies: engineered scaffolds, bioactive molecules and stem cells current stage and future perspectives. Biomaterials. 2018;180:143-162. doi: 10.1016/j.biomaterials.2018.07.017
- Andreaus U, Giorgio I, Madeo A. Modeling of the interaction between bone tissue and resorbable biomaterial as linear elastic materials with voids. Z Angew Math Phys. 2015;66(1):209-237. doi: 10.1007/s00033-014-0403-z