3D-printed electronics for biomedical applications
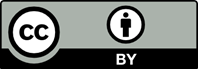
Biomedical electronics have garnered significant interest due to the rising demand for advanced healthcare devices for diagnosis, monitoring, and treatment. Three-dimensional (3D) printing, or additive manufacturing, has emerged as an attractive fabrication method for developing these advanced biomedical devices. Its unique features, such as versatility, cost-effectiveness, and rapid prototyping capabilities, when combined with medical imaging technologies enable the creation of highly precise and customized patient-specific structures. Extensive research in the field of 3D printing has focused on developing biomedical devices, including wearable and implantable devices, as well as scaffolds or platforms. Recently, the integration of 3D printing with state-of-the-art electronic materials, known for their high flexibility, conductivity, stretchability, stability, and biocompatibility, has led to the development of innovative biomedical electronics. In this review, we outline the recent advancements in 3D printing technologies and their applications in bioelectronic devices. Firstly, we describe various 3D printing methods and printable electronic materials, highlighting their advantages and limitations. Subsequently, we explore the applications of these technologies in biomedical research, spanning from surgical guidance and prosthetics to health monitoring devices and tissue-engineered scaffolds. Finally, this review discusses the current challenges and future directions to fully exploit the potential of 3D-printed bioelectronic devices, aimed at transforming personalized healthcare.

- Xu L, Qin H, Tan J, et al. Clinical study of 3D printed personalized prosthesis in the treatment of bone defect after pelvic tumor resection. J Orthop Translat. 2021;29:163-169. doi: 10.1016/j.jot.2021.05.007
- Tejo-Otero A, Lustig-Gainza P, Fenollosa-Artés F, Valls A, Krauel L, Buj-Corral I. 3D printed soft surgical planning prototype for a biliary tract rhabdomyosarcoma. J Mech Behav Biomed Mater. 2020;109:103844. doi: 10.1016/j.jmbbm.2020.103844
- Uhl JF, Sufianov A, Ruiz C, et al. The use of 3D printed models for surgical simulation of cranioplasty in craniosynostosis as training and education. Brain Sci. 2023;13(6):894. doi: 10.3390/brainsci13060894
- Wang F, Xue Y, Chen X, et al. 3D printed implantable hydrogel bioelectronics for electrophysiological monitoring and electrical modulation. Adv Funct Mater. 2024;34(21):2314471. doi: 10.1002/adfm.202314471
- Krishnadoss V, Kanjilal B, Hesketh A, et al. In situ 3D printing of implantable energy storage devices. Chem Eng J. 2021;409:128213. doi: 10.1016/j.cej.2020.128213
- Picco CJ, Domínguez-Robles J, Utomo E, et al. 3D-printed implantable devices with biodegradable rate-controlling membrane for sustained delivery of hydrophobic drugs. Drug Deliv. 2022;29(1):1038-1048. doi: 10.1080/10717544.2022.2057620
- Yi Q, Najafikhoshnoo S, Das P, et al. All‐3D‐printed, flexible, and hybrid wearable bioelectronic tactile sensors using biocompatible nanocomposites for health monitoring. Adv Mater Technol. 2022;7(5):2101034. doi: 10.1002/admt.202101034
- Tang Z, Jia S, Zhou C, Li B. 3D printing of highly sensitive and large-measurement-range flexible pressure sensors with a positive piezoresistive effect. ACS Appl Mater Interfaces. 2020;12(25):28669-28680. doi: 10.1021/acsami.0c06977
- Davoodi E, Montazerian H, Haghniaz R, et al. 3D-printed ultra-robust surface-doped porous silicone sensors for wearable biomonitoring. ACS Nano. 2020;14(2):1520-1532. doi: 10.1021/acsnano.9b06283
- Kwon Y-T, Kim Y-S, Kwon S, et al. All-printed nanomembrane wireless bioelectronics using a biocompatible solderable graphene for multimodal human-machine interfaces. Nat Commun. 2020;11(1):3450. doi: 10.1038/s41467-020-17288-0
- Yan W-C, Davoodi P, Vijayavenkataraman S, et al. 3D bioprinting of skin tissue: from pre-processing to final product evaluation. Adv Drug Deliv Rev. 2018;132: 270-295. doi: 10.1016/j.addr.2018.07.016
- Bao G, Yang P, Yi J, et al. Full-sized realistic 3D printed models of liver and tumour anatomy: a useful tool for the clinical medicine education of beginning trainees. BMC Med Educ. 2023;23(1):574. doi: 10.1186/s12909-023-04535-3
- Fu H, Zhang D, Zeng J, et al. Application of 3D-printed tissue-engineered skin substitute using innovative biomaterial loaded with human adipose-derived stem cells in wound healing. Int J Bioprint. 2023;9(2):674. doi: 10.18063/ijb.v9i2.674
- Zhu Z, Guo SZ, Hirdler T, et al. 3D printed functional and biological materials on moving freeform surfaces. Adv Mater. 2018;30(23):e1707495. doi: 10.1002/adma.201707495
- Guo X, Li H, Hong W, et al. 3D printed fourth-order star-like negative poisson’s ratio structure for high-sensitivity bionic flexible capacitive pressure sensor. IEEE Sens J. 2024;24(9) 13937-13945. doi: 10.1109/JSEN.2024.3374304
- He Q, Zeng Y, Jiang L, et al. Growing recyclable and healable piezoelectric composites in 3D printed bioinspired structure for protective wearable sensor. Nat Commun. 2023;14(1):6477. doi: 10.1038/s41467-023-41740-6
- Wei J, Xie J, Zhang P, et al. Bioinspired 3D printable, self-healable, and stretchable hydrogels with multiple conductivities for skin-like wearable strain sensors. ACS Appl Mater Interfaces. 2021;13(2):2952-2960. doi: 10.1021/acsami.0c19512
- Jain K, Wang Z, Garma LD, et al. 3D printable composites of modified cellulose fibers and conductive polymers and their use in wearable electronics. Appl Mater Today. 2023;30:101703. doi: 10.1016/j.apmt.2022.101703
- Yu J, Tian F, Wang W, et al. Design of highly conductive, intrinsically stretchable, and 3D printable PEDOT: PSS hydrogels via PSS-chain engineering for bioelectronics. Chem Mater. 2023;35(15):5936-5944. doi: 10.1021/acs.chemmater.3c00844
- Peng S, Li Y, Wu L, et al. 3D printing mechanically robust and transparent polyurethane elastomers for stretchable electronic sensors. ACS Appl Mater Interfaces. 2020; 12(5):6479-6488 doi: 10.1021/acsami.9b20631
- Tan P, Xi Y, Chao S, et al. An artificial intelligence-enhanced blood pressure monitor wristband based on piezoelectric nanogenerator. Biosensors. 2022;12(4):234. doi: 10.3390/bios12040234
- Kim B, Jang S, Geier ML, Prabhumirashi PL, Hersam MC, Dodabalapur A. High-speed, inkjet-printed carbon nanotube/zinc tin oxide hybrid complementary ring oscillators. Nano Lett. 2014;14(6):3683-3687. doi: 10.1021/nl5016014
- Minemawari H, Yamada T, Matsui H, et al. Inkjet printing of single-crystal films. Nature. 2011;475(7356):364-367. doi: 10.1038/nature10313
- De Gans BJ, Duineveld PC, Schubert US. Inkjet printing of polymers: state of the art and future developments. Adv Mater. 2004;16(3):203-213. doi: 10.1002/adma.200300385
- Calvert P. Printing cells. Science. 2007;318(5848):208-209. doi: 10.1126/science.1144212
- Cui X, Boland T. Human microvasculature fabrication using thermal inkjet printing technology. Biomaterials. 2009;30(31):6221-6227. doi: 10.1016/j.biomaterials.2009.07.056
- Huang Z, Tang Y, Guo H, et al. 3D printing of ceramics and graphene circuits-on-ceramics by thermal bubble inkjet technology and high temperature sintering. Ceramics Int. 2020;46(8):10096-10104. doi: 10.1016/j.ceramint.2019.12.278
- Wood V, Panzer MJ, Chen J, et al. Inkjet‐printed quantum dot–polymer composites for full‐color ac‐driven displays. Adv Mater. 2009;21(21):2151-2155. doi: 10.1002/adma.200990078
- Teo MY, RaviChandran N, Kim N, et al. Direct patterning of highly conductive PEDOT: PSS/ionic liquid hydrogel via microreactive inkjet printing. ACS Appl Mater Interfaces. 2019;11(40):37069-37076. doi: 10.1021/acsami.9b12069
- Mangoma TN, Yamamoto S, Malliaras GG, Daly R. Hybrid 3D/Inkjet-printed organic neuromorphic transistors. Adv Mater Technol. 2022;7(2):2000798. doi: 10.1002/admt.202000798
- Carey T, Cacovich S, Divitini G, et al. Fully inkjet-printed two-dimensional material field-effect heterojunctions for wearable and textile electronics. Nat Commun. 2017;8(1):1202. doi: 10.1038/s41467-017-01210-2
- Gibertini E, Lissandrello F, Bertoli L, Viviani P, Magagnin L. All-inkjet-printed Ti3C2 MXene capacitor for textile energy storage. Coatings. 2023;13(2):230. doi: 10.3390/coatings13020230
- Li Y, Dahhan O, Filipe CD, Brennan JD, Pelton RH. Deposited nanoparticles can promote air clogging of piezoelectric inkjet printhead nozzles. Langmuir. 2019;35(16): 5517-5524. doi: 10.1021/acs.langmuir.8b04335
- Li Y, Dahhan O, Filipe CD, Brennan JD, Pelton RH. Optimizing piezoelectric inkjet printing of silica sols for biosensor production. J Sol-Gel Sci Technol. 2018; 87:657-664. doi: 10.1007/s10971-018-4762-3
- Li J, Rossignol F, Macdonald J. Inkjet printing for biosensor fabrication: combining chemistry and technology for advanced manufacturing. Lab Chip. 2015;15(12): 2538-2558. doi: 10.1039/C5LC00235D
- Zhu W, Ma X, Gou M, Mei D, Zhang K, Chen S. 3D printing of functional biomaterials for tissue engineering. Curr Opin Biotechnol. 2016;40:103-112. doi: 10.1016/j.copbio.2016.03.014
- Yan K, Li J, Pan L, Shi Y. Inkjet printing for flexible and wearable electronics. APL Mater. 2020;8(12):120705. doi: 10.1063/5.0031669
- Shah MA, Lee D-G, Lee B-Y, Hur S. Classifications and applications of inkjet printing technology: a review. IEEE Access. 2021 doi: 10.1109/ACCESS.2021.3119219
- Tekin E, Smith PJ, Schubert US. Inkjet printing as a deposition and patterning tool for polymers and inorganic particles. Soft Matter. 2008;4(4):703-713. doi: 10.1039/B711984D
- Chung S, Cho K, Lee T. Recent progress in inkjet-printed thin-film transistors. Adv Sci (Weinh). 2019;6(6):1801445. doi: 10.1002/advs.201801445
- Rahmati S, Shirazi S, Baghayeri H. Piezo‐electric head application in a new 3D printing design. Rapid Prototyping J. 2009;15(3):187-191. doi: 10.1108/13552540910960280
- Wang Z, Wu W, Yang Q, Li Y, Noh C-H. In-situ fabrication of flexible vertically integrated electronic circuits by inkjet printing. J Alloys Compd. 2009;486(1-2):706-710. doi: 10.1016/j.jallcom.2009.07.044
- Lo L-W, Zhao J, Wan H, Wang Y, Chakrabartty S, Wang C. An inkjet-printed PEDOT: PSS-based stretchable conductor for wearable health monitoring device applications. ACS Appl Mater Interfaces. 2021;13(18):21693-21702. doi: 10.1021/acsami.1c00537
- Delekta SS, Adolfsson KH, Erdal NB, Hakkarainen M, Östling M, Li J. Fully inkjet printed ultrathin microsupercapacitors based on graphene electrodes and a nano-graphene oxide electrolyte. Nanoscale. 2019;11(21):10172-10177. doi: 10.1039/C9NR01427F
- Su C-H, Chiu H-L, Chen Y-C, et al. Highly responsive PEG/gold nanoparticle thin-film humidity sensor via inkjet printing technology. Langmuir. 2019;35(9):3256-3264. doi: 10.1021/acs.langmuir.8b03433
- Wen D, Ying G, Liu L, et al. Flexible and high‐performance MXene/MnO2 film electrodes fabricated by inkjet printing: toward a new generation supercapacitive application. Adv Mater Interfaces. 2021;8(21):2101453. doi: 10.1002/admi.202101453
- Kim JD, Choi JS, Kim BS, Choi YC, Cho YW. Piezoelectric inkjet printing of polymers: stem cell patterning on polymer substrates. Polymer. 2010;51(10):2147-2154. doi: 10.1016/j.polymer.2010.03.038
- Remaggi G, Zaccarelli A, Elviri L. 3D printing technologies in biosensors production: recent developments. Chemosensors. 2022;10(2):65. doi: 10.3390/chemosensors10020065
- Huang J, Qin Q, Wang J. A review of stereolithography: processes and systems. Processes. 2020;8(9):1138. doi: 10.3390/pr8091138
- Quan H, Zhang T, Xu H, Luo S, Nie J, Zhu X. Photo-curing 3D printing technique and its challenges. Bioact Mater. 2020;5(1):110-115. doi: 10.1016/j.bioactmat.2019.12.003
- Song Q, Chen Y, Hou P, et al. Fabrication of multi-material pneumatic actuators and microactuators using stereolithography. Micromachines. 2023;14(2):244. doi: 10.3390/mi14020244
- Pagac M, Hajnys J, Ma Q-P, et al. A review of vat photopolymerization technology: materials, applications, challenges, and future trends of 3D printing. Polymers. 2021;13(4):598. doi: 10.3390/polym13040598
- Peng X, Kuang X, Roach DJ, et al. Integrating digital light processing with direct ink writing for hybrid 3D printing of functional structures and devices. Addit Manuf. 2021;40:101911. doi: 10.1016/j.addma.2021.101911
- Liu M, Zhang Q, Shao Y, Liu C, Zhao Y. Research of a novel 3D printed strain gauge type force sensor. Micromachines. 2018;10(1):20. doi: 10.3390/mi10010020
- Kim NP, Eo J-S, Cho D. Optimization of piston type extrusion (PTE) techniques for 3D printed food. J Food Eng. 2018;235:41-49. doi: 10.1016/j.jfoodeng.2018.04.019
- Valkenaers H, Vogeler F, Voet A, Kruth J-P. Screw Extrusion Based 3D Printing, A Novel Additive Manufacturing Technology. University of Stellenbosch: Stellenbosch; 2013:97-103.
- Seoane-Viaño I, Januskaite P, Alvarez-Lorenzo C, Basit AW, Goyanes A. Semi-solid extrusion 3D printing in drug delivery and biomedicine: personalised solutions for healthcare challenges. J Control Release. 2021;332:367-389. doi: 10.1016/j.jconrel.2021.02.027
- Tetsuka H, Shin SR. Materials and technical innovations in 3D printing in biomedical applications. J Mater Chem B. 2020;8(15):2930-2950. doi: 10.1039/D0TB00034E
- Dababneh AB, Ozbolat IT. Bioprinting technology: a current state-of-the-art review. J Manuf Sci Eng. 2014;136(6):061016. doi: 10.1115/1.4028512
- Li B, Liang W, Zhang L, Ren F, Xuan F. TPU/CNTs flexible strain sensor with auxetic structure via a novel hybrid manufacturing process of fused deposition modeling 3D printing and ultrasonic cavitation-enabled treatment. Sens Actuators A: Phys. 2022;340:113526. doi: 10.1016/j.sna.2022.113526
- Ma C, Zhu B, Qian Z, Ren L, Yuan H, Meng Y. 3D-printing of conductive inks based flexible tactile sensor for monitoring of temperature, strain and pressure. J Manuf Processes. 2023;87:1-10. doi: 10.1016/j.jmapro.2023.01.008
- Park SH, Su R, Jeong J, et al. 3D printed polymer photodetectors. Adv Mater. 2018;30(40):1803980. doi: 10.1002/adma.201803980
- Zhu Z, Park HS, McAlpine MC. 3D printed deformable sensors. Sci Adv. 2020;6(25):eaba5575. doi: 10.1126/sciadv.aba5575
- Schouten M, Wolterink G, Dijkshoorn A, Kosmas D, Stramigioli S, Krijnen G. A review of extrusion-based 3d printing for the fabrication of electro-and biomechanical sensors. IEEE Sens J. 2020;21(11):12900-12912. doi: 10.1109/JSEN.2020.3042436
- Saadi MASR, Maguire A, Pottackal NT, et al. Direct ink writing: A 3D printing technology for diverse materials. Adv Mater. 2022;34(28):2108855. doi: 10.1002/adma.201707495
- Wei P, Leng H, Chen Q, Advincula RC, Pentzer EB. Reprocessable 3D-printed conductive elastomeric composite foams for strain and gas sensing. ACS Appl Polym Mater. 2019;1(4):885-892. doi: 10.1021/acsapm.9b00118
- Huang K, Dong S, Yang J, et al. Three-dimensional printing of a tunable graphene-based elastomer for strain sensors with ultrahigh sensitivity. Carbon. 2019;143:63-72. doi: 10.1016/j.carbon.2018.11.008
- Baker DV, Bao C, Kim WS. Highly conductive 3D printable materials for 3D structural electronics. ACS Appl Electron Mater. 2021;3(6):2423-2433. doi: 10.1021/acsaelm.1c00296
- Neumann TV, Dickey MD. Liquid metal direct write and 3D printing: a review. Adv Mater Technol. 2020;5(9): 2000070. doi: 10.1002/admt.202000070
- Tan HW, An J, Chua CK, Tran T. Metallic nanoparticle inks for 3D printing of electronics. Adv Electronic Mater. 2019;5(5):1800831. doi: 10.1002/aelm.201800831
- Zou Z, Chen Y, Yuan S, Luo N, Li J, He Y. 3D printing of liquid metals: recent advancements and challenges. Adv Funct Mater. 2023;33(10):2213312. doi: 10.1002/adfm.202213312
- Chen R. Liquid Metal Based Flexible Pressure Sensor for Tactile Sensing of Robots. IOP Publishing; 2021:052025. doi: 10.1088/1742-6596/1885/5/052025
- Cook A, Parekh DP, Ladd C, et al. Shear‐driven direct‐write printing of room‐temperature gallium‐based liquid metal alloys. Adv Eng Mater. 2019;21(11):1900400. doi: 10.1002/adem.201900400
- Wu P, Fu J, Xu Y, He Y. Liquid metal microgels for three-dimensional printing of smart electronic clothes. ACS Appl Mater Interfaces. 2022;14(11):13458-13467. doi: 10.1021/acsami.1c22975
- Kim S, Oh J, Jeong D, Bae J. Direct wiring of eutectic gallium–indium to a metal electrode for soft sensor systems. ACS Appl Mater Interfaces. 2019;11(22):20557-20565. doi: 10.1021/acsami.9b05363
- Wang Y, Yu Z, Mao G, et al. Printable liquid‐metal@ PDMS stretchable heater with high stretchability and dynamic stability for wearable thermotherapy. Adv Mater Technol. 2019;4(2):1800435. doi: 10.1002/admt.201800435
- Oh J, Kim S, Lee S, Jeong S, Ko SH, Bae J. A liquid metal based multimodal sensor and haptic feedback device for thermal and tactile sensation generation in virtual reality. Adv Funct Mater. 2021;31(39):2007772. doi: 10.1002/adfm.202007772
- Allen G, Bayles R, Gile W, Jesser W. Small particle melting of pure metals. Thin Solid Films. 1986;144(2):297-308. doi: 10.1016/0040-6090(86)90422-0
- McNamara K, Tofail SA. Nanoparticles in biomedical applications. Adv Phys: X. 2017;2(1):54-88. doi: 10.1080/23746149.2016.1254570
- Das S, Langbang L, Haque M, Belwal VK, Aguan K, Roy AS. Biocompatible silver nanoparticles: an investigation into their protein binding efficacies, anti-bacterial effects and cell cytotoxicity studies. J Pharm Anal. 2021;11(4):422-434. doi: 10.1016/j.jpha.2020.12.003
- Rajendran G, Rajamuthuramalingam T, Jesse DMI, Kathiravan K. Synthesis and characterization of biocompatible acetaminophen stabilized gold nanoparticles. Mater Res Express. 2019;6(9):095043. doi: 10.1088/2053-1591/ab2e4d
- Nejati K, Dadashpour M, Gharibi T, Mellatyar H, Akbarzadeh A. Biomedical applications of functionalized gold nanoparticles: a review. J Clust Sci. 2021;33:1-16. doi: 10.1007/s10876-020-01955-9
- Madhavan R. Flexible and stretchable strain sensors fabricated by inkjet printing of silver nanowire-ecoflex composites. J Mater Sci: Mater Electron. 2022;33(7):3465-3484. doi: 10.1007/s10854-022-09087-8
- Kong YL, Tamargo IA, Kim H, et al. 3D printed quantum dot light-emitting diodes. Nano Lett. 2014;14(12):7017-7023. doi: 10.1021/nl5033292
- Rosati G, Ravarotto M, Scaramuzza M, De Toni A, Paccagnella A. Silver nanoparticles inkjet-printed flexible biosensor for rapid label-free antibiotic detection in milk. Sens Actuators B: Chem. 2019;280:280-289. doi: 10.1016/j.snb.2018.09.084
- Agarwala S, Goh GL, Yap YL, et al. Development of bendable strain sensor with embedded microchannels using 3D printing. Sens Actuators A: Phys. 2017;263:593-599. doi: 10.1016/j.sna.2017.07.025
- Kwon SN, Kim SW, Kim IG, Hong YK, Na SI. Direct 3D Printing of graphene nanoplatelet/silver nanoparticle‐based nanocomposites for multiaxial piezoresistive sensor applications. Adv Mater Technol. 2019;4(2):1800500. doi: 10.1002/admt.201800500
- Ali MA, Hu C, Jahan S, et al. Sensing of COVID‐19 antibodies in seconds via aerosol jet nanoprinted reduced‐graphene‐oxide‐coated 3D electrodes. Adv Mater. 2021;33(7):2006647. doi: 10.1002/adma.202006647
- Zhu C, Han TY-J, Duoss EB, et al. Highly compressible 3D periodic graphene aerogel microlattices. Nat Commun. 2015;6(1):6962. doi: 10.1038/ncomms7962
- Zhu D, Ren Y, Liao G, et al. Thermal and mechanical properties of polyamide 12/graphene nanoplatelets nanocomposites and parts fabricated by fused deposition modeling. J Appl Polym Sci. 2017;134(39):45332. doi: 10.1002/app.45332
- Wang J, Liu Y, Fan Z, Wang W, Wang B, Guo Z. Ink-based 3D printing technologies for graphene-based materials: a review. Adv Compos Hybrid Mater. 2019;2:1-33. doi: 10.1007/s42114-018-0067-9
- Thostenson ET, Li C, Chou T-W. Nanocomposites in context. Compos Sci Technol. 2005;65(3-4):491-516. doi: 10.1016/j.compscitech.2004.11.003
- Balasubramanian K, Burghard M. Chemically functionalized carbon nanotubes. Small. 2005;1(2):180-192. doi: 10.1002/smll.200400118
- Goh GL, Agarwala S, Yeong WY. Directed and on-demand alignment of carbon nanotube: a review toward 3D printing of electronics. Adv Mater Interfaces. 2019;6(4):1801318. doi: 10.1002/admi.201801318
- Lee S-J, Wei Z, Nowicki M, et al. 3D printing nano conductive multi-walled carbon nanotube scaffolds for nerve regeneration. J Neural Eng. 2018;15(1):016018. doi: 10.1088/1741-2552/aa95a5
- Jarosova R, Mcclure SE, Gajda M, et al. Inkjet-printed carbon nanotube electrodes for measuring pyocyanin and uric acid in a wound fluid simulant and culture media. Anal Chem. 2019;91(14):8835-8844. doi: 10.1021/acs.analchem.8b05591
- Eng H, Maleksaeedi S, Yu S, et al. Development of CNTs-filled photopolymer for projection stereolithography. Rapid Prototyping J. 2017;23(1):129-136. doi: 10.1108/RPJ-10-2015-0148
- Nadernezhad A, Unal S, Khani N, Koc B. Material extrusion-based additive manufacturing of structurally controlled poly (lactic acid)/carbon nanotube nanocomposites. Int J Adv Manuf Technol. 2019;102:2119-2132 doi: 10.1007/s00170-018-03283-9
- Belaid H, Nagarajan S, Teyssier C, et al. Development of new biocompatible 3D printed graphene oxide-based scaffolds. Mater Sci Eng: C. 2020;110:110595. doi: 10.1016/j.msec.2019.110595
- eSilva EP, Huang B, Helaehil JV, et al. In vivo study of conductive 3D printed PCL/MWCNTs scaffolds with electrical stimulation for bone tissue engineering. Bio-Des Manuf. 2021;4(2):190-202. doi: 10.1007/s42242-020-00116-1
- Ryan KR, Down MP, Hurst NJ, Keefe EM, Banks CE. Additive manufacturing (3D printing) of electrically conductive polymers and polymer nanocomposites and their applications. eScience. 2022;2(4):365–381. doi: 10.1016/j.esci.2022.07.003
- Shi H, Liu C, Jiang Q, Xu J. Effective approaches to improve the electrical conductivity of PEDOT: PSS: a review. Adv Electron Mater. 2015;1(4):1500017. doi: 10.1002/aelm.201500017
- Su R, Park SH, Ouyang X, Ahn SI, McAlpine MC. 3D-printed flexible organic light-emitting diode displays. Sci Adv. 2022;8(1):eabl8798. doi: 10.1126/sciadv.abl8798
- Vuorinen T, Niittynen J, Kankkunen T, Kraft TM, Mäntysalo M. Inkjet-printed graphene/PEDOT: PSS temperature sensors on a skin-conformable polyurethane substrate. Sci Rep. 2016;6(1):35289. doi: 10.1038/srep35289
- Yang J, Cao Q, Tang X, et al. 3D-printed highly stretchable conducting polymer electrodes for flexible supercapacitors. J Mater Chem A. 2021;9(35):19649-19658. doi: 10.1039/D1TA02617H
- Shen Z, Zhang Z, Zhang N, et al. High‐stretchability, ultralow‐hysteresis conductingpolymer hydrogel strain sensors for soft machines. Adv Mater. 2022;34(32): 2203650. doi: 10.1002/adma.202203650
- Molina-Lopez F, Gao T, Kraft U, et al. Inkjet-printed stretchable and low voltage synaptic transistor array. Nat Commun. 2019;10(1):2676. doi: 10.1038/s41467-019-10569-3
- Soni M, Bhattacharjee M, Ntagios M, Dahiya R. Printed temperature sensor based on PEDOT: PSS-graphene oxide composite. IEEE Sens J. 2020;20(14):7525-7531. doi: 10.1109/JSEN.2020.2969667
- Yuk H, Lu B, Lin S, et al. 3D printing of conducting polymers. Nat Commun. 2020;11(1):1604. doi: 10.1038/s41467-020-15316-7
- Scordo G, Bertana V, Scaltrito L, et al. A novel highly electrically conductive composite resin for stereolithography. Mater Today Commun. 2019;19:12-17. doi: 10.1016/j.mtcomm.2018.12.017
- Vijayavenkataraman S, Kannan S, Cao T, Fuh JY, Sriram G, Lu WF. 3D-printed PCL/PPy conductive scaffolds as three-dimensional porous nerve guide conduits (NGCs) for peripheral nerve injury repair. Front Bioeng Biotechnol. 2019;7:266. doi: 10.3389/fbioe.2019.00266
- Qian J, Xiao R, Su F, Guo M, Liu D. 3D wet-spinning printing of wearable flexible electronic sensors of polypyrrole@ polyvinyl formate. J Ind Eng Chem. 2022;111:490-498. doi: 10.1016/j.jiec.2022.04.030
- Ajdary R, Ezazi NZ, Correia A, et al. Multifunctional 3D-printed patches for long-term drug release therapies after myocardial infarction. Adv Funct Mater. 2020;30(34):2003440. doi: 10.1002/adfm.202003440
- Distler T, Polley C, Shi F, et al. Electrically conductive and 3D-printable oxidized alginate-gelatin polypyrrole:PSS hydrogels for tissue engineering. Adv Healthc Mater. 2021;10(9):2001876. doi: 10.1002/adhm.202001876
- Gu Y, Zhang Y, Shi Y, Zhang L, Xu X. 3D all printing of polypyrrole nanotubes for high mass loading flexible supercapacitor. ChemistrySelect. 2019;4(36):10902-10906. doi: 10.1002/slct.201902721
- Li W, Liu J, Wei J, Yang Z, Ren C, Li B. Recent progress of conductive hydrogel fibers for flexible electronics: fabrications, applications, and perspectives. Adv Funct Mater. 2023;33(17):2213485. doi: 10.1002/adfm.202213485
- Yuk H, Lu B, Zhao X. Hydrogel bioelectronics. Chem Soc Rev. 2019;48(6):1642-1667. doi: 10.1039/c8cs00595h
- Kang M, Park J, Kim SA, et al. Modulus-tunable multifunctional hydrogel ink with nanofillers for 3D-Printed soft electronics. Biosens Bioelectron. 2024;255:116257. doi: 10.1016/j.bios.2024.116257
- Zhou Y, Wan C, Yang Y, et al. Highly stretchable, elastic, and ionic conductive hydrogel for artificial soft electronics. Adv Funct Mater. 2019;29(1):1806220. doi: 10.1002/adfm.201806220
- Zhou J, Yan H, Wang C, Gong H, Nie Q, Long Y. 3D printing highly stretchable conductors for flexible electronics with low signal hysteresis. Virt Phys Prototyping. 2022;17(1):19-32. doi: 10.1080/17452759.2021.1980283
- Fantino E, Roppolo I, Zhang D, et al. 3D printing/interfacial polymerization coupling for the fabrication of conductive hydrogel. Macromol Mater Eng. 2018;303(4):1700356. doi: 10.1002/mame.201700356
- Carcione R, Pescosolido F, Montaina L, et al. Self-standing 3D-printed PEGDA–PANIs electroconductive hydrogel composites for pH monitoring. Gels. 2023;9(10):784. doi: 10.3390/gels9100784
- Distler T, Boccaccini AR. 3D printing of electrically conductive hydrogels for tissue engineering and biosensors–a review. Acta Biomater. 2020;101:1-13. doi: 10.1016/j.actbio.2019.08.044
- Serafin A, Murphy C, Rubio MC, Collins MN. Printable alginate/gelatin hydrogel reinforced with carbon nanofibers as electrically conductive scaffolds for tissue engineering. Mater Sci Eng: C. 2021;122:111927. doi: 10.1016/j.msec.2021.111927
- Liu J, Garcia J, Leahy LM, et al. 3D printing of multifunctional conductive polymer composite hydrogels. Adv Funct Mater. 2023;33(37):2214196. doi: 10.1002/adfm.202214196
- Deng Z, Hu T, Lei Q, He J, Ma PX, Guo B. Stimuli-responsive conductive nanocomposite hydrogels with high stretchability, self-healing, adhesiveness, and 3D printability for human motion sensing. ACS Appl Mater Interfaces. 2019;11(7):6796-6808. doi: 10.1021/acsami.8b20178
- Qiu K, Haghiashtiani G, McAlpine MC. 3D printed organ models for surgical applications. Annu Rev Anal Chem (Palo Alto Calif). 2018;11(1):287-306. doi: 10.1146/annurev-anchem-061417-125935
- Mitsouras D, Liacouras P, Imanzadeh A, et al. Medical 3D printing for the radiologist. Radiographics. 2015;35(7): 1965-1988. doi: 10.1148/rg.2015140320
- Qiu K, Zhao Z, Haghiashtiani G, et al. 3D printed organ models with physical properties of tissue and integrated sensors. Adv Mater Technol. 2018;3(3):1700235. doi: 10.1002/admt.201700235
- Haghiashtiani G, Qiu K, Zhingre Sanchez JD, et al. 3D printed patient-specific aortic root models with internal sensors for minimally invasive applications. Sci Adv. 2020;6(35):eabb4641. doi: 10.1126/sciadv.abb4641
- Said S, Boulkaibet I, Sheikh M, Karar AS, Alkork S, Nait-ali A. Machine-learning-based muscle control of a 3D-printed bionic arm. Sensors. 2020;20(11):3144. doi: 10.3390/s20113144
- Corona-Castuera J, Rodriguez-Delgado D, Henao J, Castro- Sandoval JC, Poblano-Salas CA. Design and fabrication of a customized partial hip prosthesis employing CT-scan data and lattice porous structures. ACS Omega. 2021;6(10):6902-6913. doi: 10.1021/acsomega.0c06144
- Sang S, Pei Z, Zhang F, et al. Three-dimensional printed bimodal electronic skin with high resolution and breathability for hair growth. ACS Appl Mater Interfaces. 2022;14(27):31493-31501. doi: 10.1021/acsami.2c09311
- Gao H, An J, Chua CK, Bourell D, Kuo C-N, Tan DT. 3D printed optics and photonics: processes, materials and applications. Mater Today. 2023;69:107-132. doi: 10.1016/j.mattod.2023.06.019
- Kong YL, Gupta MK, Johnson BN, McAlpine MC. 3D printed bionic nanodevices. Nano Today. 2016;11(3):330-350. doi: 10.1016/j.nantod.2016.04.007
- Mannoor MS, Jiang Z, James T, et al. 3D printed bionic ears. Nano Lett. 2013;13(6):2634-2639. doi: 10.1021/nl4007744
- Lu L, Zhang J, Xie Y, et al. Wearable health devices in health care: narrative systematic review. JMIR mHealth uHealth. 2020;8(11):e18907. doi: 10.2196/18907
- Ho DH, Hong P, Han JT, et al. 3D‐printed sugar scaffold for high‐precision and highly sensitive active and passive wearable sensors. Adv Sci. 2020;7(1):1902521. doi: 10.1002/advs.201902521
- Ouyang X, Su R, Ng DWH, Han G, Pearson DR, McAlpine MC. 3D printed skin-interfaced UV-visible hybrid photodetectors. Adv Sci (Weinh). 2022;9(25):e2201275. doi: 10.1002/advs.202201275
- Wang Z, Gao W, Zhang Q, et al. 3D-printed graphene/ polydimethylsiloxane composites for stretchable and strain-insensitive temperature sensors. ACS Appl Mater Interfaces. 2019;11(1):1344-1352. doi: 10.1021/acsami.8b16139
- Hou Y, Gao M, Gao J, et al. 3D printed conformal strain and humidity sensors for human motion prediction and health monitoring via machine learning. Adv Sci (Weinh). 2023;10(36):e2304132. doi: 10.1002/advs.202304132
- Zhu G, Dai H, Yao Y, et al. 3D printed skin‐inspired flexible pressure sensor with gradient porous structure for tunable high sensitivity and wide linearity range. Adv Mater Technol. 2022;7(7):2101239. doi: 10.1002/admt.202101239
- Herbert R, Lim H-R, Rigo B, Yeo W-H. Fully implantable wireless batteryless vascular electronics with printed soft sensors for multiplex sensing of hemodynamics. Sci Adv. 2022;8(19):eabm1175. doi: 10.1126/sciadv.abm1175
- Chen C, Bai X, Ding Y, Lee I-S. Electrical stimulation as a novel tool for regulating cell behavior in tissue engineering. Biomater Res. 2019;23(1):1-12. doi: 10.1186/s40824-019-0176-8
- Bedir T, Ulag S, Aydogan K, et al. Effect of electric stimulus on human adipose‐derived mesenchymal stem cells cultured in 3D‐printed scaffolds. Polym Adv Technol. 2021;32(3):1114-1125. doi: 10.1002/pat.5159
- Lind JU, Busbee TA, Valentine AD, et al. Instrumented cardiac microphysiological devices via multimaterial three-dimensional printing. Nat Mater. 2017;16(3):303-308. doi: 10.1038/nmat4782
- Wu Q, Zhang P, O’Leary G, et al. Flexible 3D printed microwires and 3D microelectrodes for heart-on-a-chip engineering. Biofabrication. 2023;15(3):035023. doi: 10.1088/1758-5090/acd8f4
- Aimar A, Palermo A, Innocenti B. The role of 3D printing in medical applications: a state of the art. J Healthc Eng. 2019;2019:5340616. doi: 10.1155/2019/5340616
- Yan Q, Dong H, Su J, et al. A review of 3D printing technology for medical applications. Engineering. 2018;4(5):729-742. doi: 10.1016/j.eng.2018.07.021
- Ventola CL. Medical applications for 3D printing: current and projected uses. P T. 2014;39(10):704-711.
- Jiang Y, Islam MN, He R, et al. Recent advances in 3D printed sensors: materials, design, and manufacturing. Adv Mater Technol. 2023;8(2):2200492. doi: 10.1002/admt.202200492
- Jin J, Zhang F, Yang Y, et al. Hybrid multimaterial 3D printing using photocuring‐while‐dispensing. Small. 2023;19(50):2302405. doi: 10.1002/smll.202302405
- Pless CJ, Nikzad S, Papiano I, et al. Soft electronic block copolymer elastomer composites for multi‐material printing of stretchable physiological sensors on textiles. Adv Electron Mater. 2023;9(5):2201173. doi: 10.1002/aelm.202201173
- Balakrishnan G, Song J, Mou C, Bettinger CJ. Recent progress in materials chemistry to advance flexible bioelectronics in medicine. Adv Mater. 2022;34(10):2106787. doi: 10.1002/adma.202106787
- Pillai S, Upadhyay A, Sayson D, Nguyen BH, Tran SD. Advances in medical wearable biosensors: Design, fabrication and materials strategies in healthcare monitoring. Molecules. 2021;27(1):165. doi: 10.3390/molecules27010165
- Chow L, Yick Kl, Wong KH, et al. 3D printing auxetic architectures for hypertrophic scar therapy. Macromol Mater Eng. 2022;307(5):2100866. doi: 10.1002/mame.202100866
- Dong S, Hu H. Sensors based on auxetic materials and structures: a review. Materials. 2023;16(9):3603. doi: 10.3390/ma16093603
- Ma C, Li G, Qin L, et al. Analytical model of micropyramidal capacitive pressure sensors and machine‐learning‐assisted design. Adv Mater Technol. 2021;6(12):2100634. doi: 10.1002/admt.202100634