Effects of modeling strategies of triply periodic minimal surface on the mechanical properties and permeability of biomedical TC4 porous scaffolds
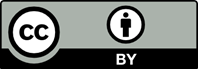
Modeling strategies play a crucial role in determining the unit shapes of triply periodic minimal surface (TPMS), significantly affecting the mechanical and permeability properties of porous scaffolds. In this study, two distinct strategies including surface thickening and surface filling were used to construct scaffold models based on four basic TPMS structures (Primitive [P], Gyroid [G], Diamond [D], and I-graph-wrapped package [IW-P]). These models were successfully prepared using TC4 alloy and selective laser melting technology. Macro/micro morphology, mechanical properties, and permeability tests of porous implants were carried out. The results indicate that the scaffolds effectively replicated the designed models, exhibiting mechanical properties that match those of human tissue. The elastic modulus ranges from 3.03 to 4.57 GPa, and the tensile strength varies between 135.78 and 250.90 MPa. The surface thickening strategy alters the material distribution within the unit, enhancing load uniformity on the scaffolds, thereby increasing the strength of the scaffolds with G, D, and IW-P units, while reducing stress fluctuations during compression. In contrast, the surface filling structure exhibits excellent permeability, with permeability rates falling within the range of 0.88 to 1.91 × 10-9 m2, aligning with the permeability performance of trabecular bone. This study offers new insights into the design of porous scaffold models tailored for various application scenarios.
- Gupta K, Meena K. Artificial bone scaffolds and bone joints by additive manufacturing: a review. Bioprinting. 2023;31:e00268. doi: 10.1016/j.bprint.2023.e00268
- Fang Y, Wang Q, Yang Z, et al. An efficient approach to endow TiNbTaZr implant with osteogenic differentiation and antibacterial activity in vitro. Mater Des. 2022;221: 110987. doi: 10.1016/j.matdes.2022.110987
- Zhang Y, Attarilar S, Wang L, Lu W, Yang J, Fu Y. A review on design and mechanical properties of additively manufactured NiTi implants for orthopedic applications. Int J Bioprint. 2021;7(2):340. doi: 10.18063/ijb.v7i2.340
- Qu H. Additive manufacturing for bone tissue engineering scaffolds. Mater Today Commun. 2020;24:101024. doi: 10.1016/j.mtcomm.2020.101024
- Yuan L, Ding S, Wen C. Additive manufacturing technology for porous metal implant applications and triple minimal surface structures: a review. Bioact Mater. 2019;4:56-70. doi: 10.1016/j.bioactmat.2018.12.003
- Wang X, Xu S, Zhou S, et al. Topological design and additive manufacturing of porous metals for bone scaffolds and orthopaedic implants: a review. Biomaterials. 2016;83: 127-141. doi: 10.1016/j.biomaterials.2016.01.012
- Oh I-H, Nomura N, Masahashi N, Hanada S. Mechanical properties of porous titanium compacts prepared by powder sintering. Scr Mater. 2003;49(12):1197-1202. doi: 10.1016/j.scriptamat.2003.08.018
- Yang G, Xu B, Lei X, et al. Preparation of porous titanium by direct in-situ reduction of titanium sesquioxide. Vacuum. 2018;157:453-457. doi: 10.1016/j.vacuum.2018.09.021
- Wang L, Xie L, Zhang L, et al. Microstructure evolution and superelasticity of layer-like NiTiNb porous metal prepared by eutectic reaction. Acta Mater. 2018;143:214-226. doi: 10.1016/j.actamat.2017.10.021
- Biasetto L, de Moraes EG, Colombo P, Bonollo F. Ovalbumin as foaming agent for Ti6Al4V foams produced by gelcasting. J Alloys Compd. 2016;687:839-844. doi: 10.1016/j.jallcom.2016.06.218
- Ma HY, Wang JC, Qin P, et al. Advances in additively manufactured titanium alloys by powder bed fusion and directed energy deposition: microstructure, defects, and mechanical behavior. J Mech Behav Biomed Mater. 2024;183:32-62. doi: 10.1016/j.jmst.2023.11.003
- Hafeez N, Liu J, Wang L, et al. Superelastic response of low-modulus porous beta-type Ti-35Nb-2Ta-3Zr alloy fabricated by laser powder bed fusion. Addit Manuf. 2020;34. doi: 10.1016/j.addma.2020.101264
- Hafeez N, Wei D, Xie L, et al. Evolution of microstructural complex transitions in low-modulus β-type Ti-35Nb-2Ta- 3Zr alloy manufactured by laser powder bed fusion. Addit Manuf. 2021;48. doi: 10.1016/j.addma.2021.102376
- Zhang T, Wei D, Lu E, et al. Microstructure evolution and deformation mechanism of α+β dual-phase Ti-xNb-yTa- 2Zr alloys with high performance. J Mater Sci Technol. 2022;131:68-81. doi: 10.1016/j.jmst.2022.04.052
- Lv Y, Wang B, Liu G, et al. Metal material, properties and design methods of porous biomedical scaffolds for additive manufacturing: a review. Front Bioeng Biotechnol. 2021;9:641130. doi: 10.3389/fbioe.2021.641130
- Guo W, Yang Y, Liu C, et al. 3D printed TPMS structural PLA/GO scaffold: process parameter optimization, porous structure, mechanical and biological properties. J Mech Behav Biomed Mater. 2023;142. doi: 10.1016/j.jmbbm.2023.105848
- Belda R, Megías R, Marco M, Vercher-Martínez A, Giner E. Numerical analysis of the influence of triply periodic minimal surface structures morphometry on the mechanical response. Comput Methods Programs Biomed. 2023;230:107342. doi: 10.1016/j.cmpb.2023.107342
- Yang L, Li Y, Wu S, et al. Tailorable and predictable mechanical responses of additive manufactured TPMS lattices with graded structures. Mat Sci Eng A. 2022;843. doi: 10.1016/j.msea.2022.143109
- Yang L, Han C, Wu H, et al. Insights into unit cell size effect on mechanical responses and energy absorption capability of titanium graded porous structures manufactured by laser powder bed fusion, J Mech Behav Biomed Mater. 2020;109:103843. doi: 10.1016/j.jmbbm.2020.103843
- Kaur I, Singh P. Flow and thermal transport characteristics of triply-periodic minimal surface (TPMS)-based gyroid and Schwarz-P cellular materials. Numer Heat Transfer, Part A. 2021;79(8):553-569. doi: 10.1080/10407782.2021.1872260
- Wu G, More KL, Johnston CM, Zelenay P. High-performance electrocatalysts for oxygen reduction derived from polyaniline, iron, and cobalt. Science. 2011;332(6028): 443-447. doi: 10.1126/science.1200832
- Yadroitsev I, Shishkovsky I, Bertrand P, Smurov I. Manufacturing of fine-structured 3D porous filter elements by selective laser melting. Appl Surf Sci. 2009;255(10): 5523-5527. doi: 10.1016/j.apsusc.2008.07.154
- Attarilar S, Ebrahimi M, Djavanroodi F, Fu Y, Wang L, Yang J. 3D printing technologies in metallic implants: a thematic review on the techniques and procedures. Int J Bioprint. 2021;7(1). doi: 10.18063/ijb.v7i1.306
- Yoo D. Computer-aided porous scaffold design for tissue engineering using triply periodic minimal surfaces. Int J Precis Eng Manuf. 2011;12(1):61-71. doi: 10.1007/s12541-011-0008-9
- Ma S, Tang Q, Han X, et al. Manufacturability, mechanical properties, mass-transport properties and biocompatibility of triply periodic minimal surface (TPMS) porous scaffolds fabricated by selective laser melting. Mater Des. 2020;195. doi: 10.1016/j.matdes.2020.109034
- Ma S, Tang Q, Feng Q, Song J, Han X, Guo F. Mechanical behaviours and mass transport properties of bone-mimicking scaffolds consisted of gyroid structures manufactured using selective laser melting. J Mech Behav Biomed Mater. 2019;93:158-169. doi: 10.1016/j.jmbbm.2019.01.023
- Yang N, Quan Z, Zhang D, Tian Y. Multi-morphology transition hybridization CAD design of minimal surface porous structures for use in tissue engineering. Comput- Aided Des. 2014;56:11-21. doi: 10.1016/j.cad.2014.06.006
- Aliyu A, Poungsiri K, Shinjo J, Panwisawas C, et al. Additive manufacturing of tantalum scaffolds:Processing, microstructure and process-induced defects, Int J Refract Met H. 2023;112:106132. doi: 10.1016/j.ijrmhm.2023.106132
- Naghavi SA, Tamaddon M, Marghoub A, et al. Mechanical characterisation and numerical modelling of TPMS-based gyroid and diamond Ti6Al4V scaffolds for bone implants: an integrated approach for translational consideration. Bioengineering. 2022;9(10). doi: 10.3390/bioengineering9100504
- Lu Y, Cheng L, Yang Z, Li J, Zhu H. Relationship between the morphological, mechanical and permeability properties of porous bone scaffolds and the underlying microstructure. Plos One. 2020;15(9). doi: 10.1371/journal.pone.0238471
- Guo X, Zheng X, Yang Y, Yang X, Yi Y. Mechanical behavior of TPMS-based scaffolds: a comparison between minimal surfaces and their lattice structures. SN Appl Sci. 2019;1(10). doi: 10.1007/s42452-019-1167-z
- Zhang X, Fang G, Xing L, Liu W, Zhou J. Effect of porosity variation strategy on the performance of functionally graded Ti-6Al-4V scaffolds for bone tissue engineering. Mater Des. 2018;157:523-538. doi: 10.1016/j.matdes.2018.07.064
- Pires T, Santos J, Ruben RB, Gouveia BP, Castro APG, Fernandes PR. Numerical-experimental analysis of the permeability-porosity relationship in triply periodic minimal surfaces scaffolds. J Biomech. 2021;117. doi: 10.1016/j.jbiomech.2021.110263
- Santos J, Pires T, Gouveia BP, Castro APG, Fernandes PR. On the permeability of TPMS scaffolds. J Mech Behav Biomed Mater. 2020;110. doi: 10.1016/j.jmbbm.2020.103932
- Zhao S, Hou W, Xu Q, Li SJ, Hao YL, Yang R. Ti-6Al-4V lattice structures fabricated by electron beam melting for biomedical applications. Titanium Med Dent Appl. 2018; 277-301. doi: 10.1016/B978-0-12-812456-7.00013-5
- Yan C, Hao L, Hussein A, Young P, Raymont D. Advanced lightweight 316L stainless steel cellular lattice structures fabricated via selective laser melting. Mater Des. 2014;55: 533-541. doi: 10.1016/j.matdes.2013.10.027
- Li X, Xiong Y-Z, Zhang H, Gao R-N. Development of functionally graded porous titanium/silk fibroin composite scaffold for bone repair. Mater Lett. 2021;282. doi: 10.1016/j.matlet.2020.128670
- Huo P, Zhao Z, Bai P, et al. Deformation evolution and fracture mechanism of porous TC4 alloy scaffolds fabricated using selective laser melting under uniaxial compression. J Alloys Compd. 2021;861:158529. doi: 10.1016/j.jallcom.2020.158529
- Wang S, Liu L, Li K, Zhu L, Chen J, Hao Y. Pore functionally graded Ti6Al4V scaffolds for bone tissue engineering application. Mater Des. 2019;168. doi: 10.1016/j.matdes.2019.107643
- McGregor M, Patel S, McLachlin S, Vlasea M. Architectural bone parameters and the relationship to titanium lattice design for powder bed fusion additive manufacturing. Addit Manuf. 2021;47. doi: 10.1016/j.addma.2021.102273
- Hara D, Nakashima Y, Sato T, et al. Bone bonding strength of diamond-structured porous titanium-alloy implants manufactured using the electron beam-melting technique. Mater Sci Eng C Mater Biol Appl. 2016;59:1047-1052. doi: 10.1016/j.msec.2015.11.025
- Wang S, Shi Z, Liu L, Zhou X, Zhu L, Hao Y. The design of Ti6Al4V primitive surface structure with symmetrical gradient of pore size in biomimetic bone scaffold. Mater Des. 2020;193. doi: 10.1016/j.matdes.2020.108830
- Carluccio D, Xu C, Venezuela J, et al. Additively manufactured iron-manganese for biodegradable porous load-bearing bone scaffold applications. Acta Biomater. 2020;103:346-360. doi: 10.1016/j.actbio.2019.12.018
- Lv Y, Liu G, Wang B, et al. Pore strategy design of a novel NiTi- Nb biomedical porous scaffold based on a triply periodic minimal surface. Front Bioeng Biotechnol. 2022;10:910475. doi: 10.3389/fbioe.2022.910475
- Lv Y, Guo J, Zhang Q, Wei G, Yu H. Design of low elastic modulus and high strength TC4 porous scaffolds via new variable gradient strategies. Mater Lett. 2022;325: 132616. doi: 10.1016/j.matlet.2022.132616
- Tan C, Zou J, Li S, et al. Additive manufacturing of bio-inspired multi-scale hierarchically strengthened lattice structures. Int J Mach Tools Manuf. 2021;167. doi: 10.1016/j.ijmachtools.2021.103764
- Yu G, Li Z, Li S, et al. The select of internal architecture for porous Ti alloy scaffold: a compromise between mechanical properties and permeability. Mater Des. 2020;192:108754. doi: 10.1016/j.matdes.2020.108754
- Xiong Y, Gao R, Zhang H, Dong L-L, Li J-T, Li X. Rationally designed functionally graded porous Ti6Al4V scaffolds with high strength and toughness built via selective laser melting for load-bearing orthopedic applications. J Mech Behav Biomed Mater. 2020;104:103673. doi: 10.1016/j.jmbbm.2020.103673
- Bobbert FSL, Lietaert K, Eftekhari AA, et al. Additively manufactured metallic porous biomaterials based on minimal surfaces: a unique combination of topological, mechanical, and mass transport properties. Acta Biomater. 2017;53:572-584. doi: 10.1016/j.actbio.2017.02.024
- Lv Y, Wang B, Liu G, et al. Design of bone-like continuous gradient porous scaffold based on triply periodic minimal surfaces. J Mater Res Technol. 2022;21:3650-3665. doi: 10.1016/j.jmrt.2022.10.160
- Xiong Y, Han Z, Qin J, et al. Effects of porosity gradient pattern on mechanical performance of additive manufactured Ti- 6Al-4V functionally graded porous structure. Mater Des. 2021;208. doi: 10.1016/j.matdes.2021.109911
- Zhao S, Li S, Hou W, Hao YL, Yang R, Misra RDK. The influence of cell morphology on the compressive fatigue behavior of Ti-6Al-4V meshes fabricated by electron beam melting. J Mech Behav Biomed Mater. 2016;59: 251-264. doi: 10.1016/j.jmbbm.2016.01.034
- Heinl P, Muller L, Korner C, Singer RF, Müller FA. Cellular Ti-6Al-4V structures with interconnected macro porosity for bone implants fabricated by selective electron beam melting. Acta Biomater. 2008;4(5):1536-1544. doi: 10.1016/j.actbio.2008.03.013
- Heinl P, Körner C, Singer RF. Selective electron beam melting of cellular titanium: mechanical properties. Adv Eng Mater. 2008;10(9):882-888. doi: 10.1002/adem.200800137
- Cheng X, Li S, Murr L, et al. Compression deformation behavior of Ti-6Al-4V alloy with cellular structures fabricated by electron beam melting. J Mech Behav Biomed Mater. 2012;16:153-162. doi: 10.1016/j.jmbbm.2012.10.005
- Chen S, Huang J, Pan C, et al. Microstructure and mechanical properties of open-cell porous Ti-6Al-4V fabricated by selective laser melting. J Alloys Compd. 2017;713:248-254. doi: 10.1016/j.jallcom.2017.04.190
- Nauman EA, Fong KE, Keaveny TM. Dependence of intertrabecular permeability on flow direction and anatomic site. Ann Biomed Eng. 1999;27:517-524. doi: 10.1114/1.195
- Zhang X-Y, Yan X-C, Fang G, Liu M. Biomechanical influence of structural variation strategies on functionally graded scaffolds constructed with triply periodic minimal surface. Addit Manuf. 2020;32. doi: 10.1016/j.addma.2019.101015
- Zou S, Mu Y, Pan B, et al. Mechanical and biological properties of enhanced porous scaffolds based on triply periodic minimal surfaces. Mater Des. 2022;219. doi: 10.1016/j.matdes.2022.110803
- Wang S, Shi Z, Liu L, et al. Honeycomb structure is promising for the repair of human bone defects. Mater Des. 2021;207. doi: 10.1016/j.matdes.2021.109832
- Wang H, Su K, Su L, Liang P, Ji P, Wang C. Comparison of 3D-printed porous tantalum and titanium scaffolds on osteointegration and osteogenesis. Mater Sci Eng C Mater Biol Appl. 2019;104:109908. doi: 10.1016/j.msec.2019.109908
- Li Y, Zhou J, Pavanram P, et al. Additively manufactured biodegradable porous magnesium. Acta Biomater. 2018;67:378-392. doi: 10.1016/j.actbio.2017.12.008
- Wang Z, Wang C, Li C, et al. Analysis of factors influencing bone ingrowth into three-dimensional printed porous metal scaffolds: a review, J Alloy Compd. 2017;717: 271-285. doi: 10.1016/j.jallcom.2017.05.079
- Li Y, Shi Y, Lu Y, et al. Additive manufacturing of vascular stents. Acta Biomater. 2023;167:16-37. doi: 10.1016/j.actbio.2023.06.014
- Zhang Y, Liu J, Wang L, et al. Porous NiTiNb alloys with superior strength and ductility induced by modulating eutectic microregion. Acta Mater. 2022;239:118295. doi: 10.1016/j.actamat.2022.118295
- Lv Y, Shi Z, Wang B, et al. Design of biomedical gradient porous scaffold via a minimal surface dual-unit continuous transition connection strategy. Int J Bioprint. 2024;10(1). doi: 10.36922/ijb.1263
- Zhang Y, Wei D, Chen Y, et al. Non-negligible role of gradient porous structure in superelasticity deterioration and improvement of NiTi shape memory alloys. J Mater Sci Technol. 2024;186:48-63. doi: 10.1016/j.jmst.2023.10.053