Frequency response analysis and in vitro verification of 3D-printed ossicular replacement materials
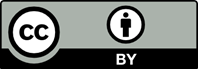
As a bridge that transmits airborne sound signals to the auditory receptors of the inner ear, the eardrum and ossicular chain of the middle ear convert sound through two types of conversions: gas–solid (airborne sound signal–eardrum and ossicular chain) and solid–liquid (eardrum and ossicular chain–internal and external lymphatic fluid in the cochlea). This process concentrates and amplifies the sound to the inner ear through the lever principle structure formed by the three ossicles. However, diseases, hereditary factors, or trauma can reduce the sound transmission function of the middle ear. The effectiveness of middle ear replacement prostheses depends on their vibration response to the human auditory perception frequency, from the eardrum to the stapes plate. This response is influenced by the materials, geometry, and design of the replacement prosthesis and eardrum. This study explores the effects of different materials on hearing after artificial ossicular replacement. Usually, human temporal bone models are used for testing and validating numerical results. However, obtaining specimens from living humans is not always feasible. Therefore, we used three-dimensional printing technology to build a model of the middle ear to test the ossicular bone. Titanium alloy TC4, stainless steel 316L, and composite HA/PCL are chosen as materials for ossicular replacement. Using finite element analysis and an in vitro verification experiment, individual replacements of the ossicles and three bone material replacements were conducted for frequency response analysis. The combination of the malleus made of TC4, the incus made of TC4, and the stapes made of HA/PCL were found to bear higher resemblance to a real normal ear ossicular model.
- Bornitz M, Hardtke HJ, Zahnert T. Evaluation of implantable actuators by means of a middle ear simulation model. Hear Res. 2010;263(1-2):145-151. doi: 10.1016/j.heares.2010.02.007
- Cheng JT, Ravicz M, Guignard J, Furlong C, Rosowski JJ. The effect of ear canal orientation on tympanic membrane motion and the sound field near the tympanic membrane. J Assoc Res Otolaryngol. 2015;16:413-432. doi: 10.1007/s10162-015-0516-x
- Eiber A. Mechanical modeling and dynamical behavior of the human middle ear. Audiol Neurotol. 1999;4(3-4): 170-177. doi: 10.1159/000013837
- Ferris P, Prendergast PJ. Middle-ear dynamics before and after ossicular replacement. J Biomech. 2000;33(5):581-590. doi: 10.1016/s0021-9290(99)00213-4
- Aernouts J, Aerts JR, Dirckx JJ. Mechanical properties of human tympanic membrane in the quasi-static regime from in situ point indentation measurements. Hear Res. 2012;290(1-2):45-54. doi: 10.1016/j.heares.2012.05.001
- De Greef D, Pires F, Dirckx JJ. Effects of model definitions and parameter values in finite element modeling of human middle ear mechanics. Hear Res. 2017;344:195-206. doi: 10.1016/j.heares.2016.11.011
- Kim N, Steele CR, Puria S. The importance of the hook region of the cochlea for bone-conduction hearing. Biophys J. 2014;107(1):233-241. doi: 10.1016/j.bpj.2014.04.052
- Aibara R, Welsh JT, Puria S, Goode RL. Human middle-ear sound transfer function and cochlear input impedance. Hear Res. 2001;152(1-2):100-109. doi: 10.1016/S0378-5955(00)00240-9
- Gan RZ, Sun Q, Dyer RK, Chang K-H, Dormer KJ. Three-dimensional modeling of middle ear biomechanics and its applications. Otol Neurotol. 2002;23(3):271-280. doi: 10.1097/00129492-200205000-00008
- Huber A, Linder T, Dillier N, et al. Intraoperative assessment of stapes movement. Ann Otol Rhinol Laryngol. 2001;110(1):31-35. doi: 10.1177/000348940111000106
- Rohani SA, Ghomashchi S, Agrawal SK, Ladak HM. Estimation of the Young’s modulus of the human pars tensa using in-situ pressurization and inverse finite-element analysis. Hear Res. 2017;345:69-78. doi: 10.1016/j.heares.2017.01.002
- De Greef D, Aernouts J, Aerts J, et al. Viscoelastic properties of the human tympanic membrane studied with stroboscopic holography and finite element modeling. Hear Res. 2014;312:69-80. doi: 10.1016/j.heares.2014.03.002
- Salmi M. Additive manufacturing processes in medical applications. Materials. 2021;14(1):191. doi: 10.3390/ma14010191
- Iwasa J, Engebretsen L, Shima Y, Ochi M. Clinical application of scaffolds for cartilage tissue engineering. Knee Surg Sports Traumatol Arthrosc. 2009;17(6):561-577. doi: 10.1007/s00167-008-0663-2
- Liu H, Cheng J, Yang J, et al. Concept and evaluation of a new piezoelectric transducer for an implantable middle ear hearing device. Sensors. 2017;17(11):2515. doi: 10.3390/s17112515
- Xue L, Liu H, Yang J, Liu S, Zhao Y, Huang X. Research on coupling effects of actuator and round window membrane on reverse stimulation of human cochlea. Proc Inst Mech Eng H. 2021;235(4):447-458. doi: 10.1177/0954411920987960
- Kuru I, Maier H, Müller M, Lenarz T, Lueth TC. A 3D-printed functioning anatomical human middle ear model. Hear Res. 2016;340:204-213. doi: 10.1016/j.heares.2015.12.025