Design and optimization of 3D-bioprinted cell-laden scaffolds in dynamic culture
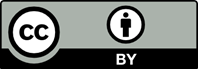
Light-based 3D printing enables the fabrication of biological scaffolds with high precision, versatility and biocompatibility, particularly the cell-laden scaffolds with architecturally complex geometric features. However, many bioprinted tissue scaffolds suffer from low cell viability due to insufficient oxygen and nutrient supply, which is heavily influenced by scaffold structure and cultivation conditions. Current practice relies mainly on resource-intensive trial-and-error methods to optimize scaffolds’ structures and cultivation parameters. In this study, we developed a comprehensive multi-physics model integrating fluid dynamics, oxygen mass transfer, cell oxygen consumption, and cell growth processes to capture cell growth behaviors in scaffolds, establishing a robust theoretical foundation for scaffold structure optimization. The modeling results showed that a large number of parameters, such as system inlet flow rate, geometric feature size, cell parameters, and material properties, significantly impact oxygen concentration and cell growth within the scaffold. A two-step optimization strategy is proposed in this paper and was applied to obtain optimal geometric parameters of channeled scaffolds to demonstrate the model’s effectiveness for scaffold optimization. The model can be employed for scaffolds with arbitrary shapes and various materials, facilitating the optimal design of sophisticated scaffolds for more advanced tissue engineering.
- Ventola CL. Medical applications for 3D printing: current and projected uses. P&T. 2014;39(10):704-711.
- Reddy VS, Ramasubramanian B, Telrandhe VM, Ramakrishna S. Contemporary standpoint and future of 3D bioprinting in tissue/organs printing. Curr Opin Biomed Eng. 2023;27:100461. doi: 10.1016/j.cobme.2023.100461
- Murphy SV, Atala AJ. 3D bioprinting of tissues and organs. Nat Biotechnol. 2014;32:773-785. doi: 10.1038/nbt.2958
- Muskan, Gupta D, Negi NP. 3D bioprinting: printing the future and recent advances. Bioprinting. 2022;27:2405-8866. doi: 10.1016/j.bprint.2022.e00211
- Matai I, Kaur G, Seyedsalehi A, McClinton A, Laurencin CT. Progress in 3D bioprinting technology for tissue/organ regenerative engineering. Biomaterials. 2020;226:119536. doi: 10.1016/j.biomaterials.2019.119536
- Li T, Chang J, Zhu Y, Wu C. 3D printing of bioinspired biomaterials for tissue regeneration. Adv Healthc Mater. 2020;9(23):2000208. doi: 10.1002/adhm.202000208
- Javaid M, Haleem A. 3D printing applications towards the required challenge of stem cells printing. Clin Epidemiol Global Health. 2020;8:862-867. doi: 10.1016/j.cegh.2020.02.014
- Cidonio G, Glinka M, Dawson JI, Oreffo ROC. The cell in the ink: improving biofabrication by printing stem cells for skeletal regenerative medicine. Biomaterials. 2019;20910-24. doi: 10.1016/j.biomaterials.2019.04.009
- Sun Y, Yu K, Gao Q, He Y. Projection-based 3D bioprinting for hydrogel scaffold manufacturing. Bio-Des Manuf. 2022;5:633-639. doi: 10.1007/s42242-022-00189-0
- He Y-X, Wang F, Wang X, Zhang J, Wang D, Huang X. A photocurable hybrid chitosan/acrylamide bioink for DLP based 3D bioprinting. Mater Des. 2021;202:109588. doi: 10.1016/j.matdes.2021.109588
- Tao J-L, Zhu S, Liao X, et al. DLP-based bioprinting of void-forming hydrogels for enhanced stem-cell-mediated bone regeneration. Mater Today Bio. 2022;17:100487. doi: 10.1016/j.mtbio.2022.100487
- Lee A, Hudson AR, Shiwarski DJ, et al. 3D bioprinting of collagen to rebuild components of the human heart. Science. 2019;365:482-487. doi: 10.1126/science.aav9051
- Lei D, Yang Y, Liu Z, et al. 3D printing of biomimetic vasculature for tissue regeneration. Mater Horiz. 2019;6(6):1197-1206. doi: 10.1039/C9MH00174C
- Grigoryan B, Paulsen SJ, Corbett DC, et al. Multivascular networks and functional intravascular topologies within biocompatible hydrogels. Science. 2019;364:458-464. doi: 10.1126/science.aav9750
- He N, Wang X, Shi L, et al. Photoinhibiting via simultaneous photoabsorption and free-radical reaction for high-fidelity light-based bioprinting. Nat Commun. 2023;14(1):3063. doi: 10.1038/s41467-023-38838-2
- Bernal PN, Bouwmeester MC, Madrid-Wolff J, et al. Volumetric bioprinting of organoids and optically tuned hydrogels to build liver‐like metabolic biofactories. Adv Mater. 2022;34(15):2110054. doi: 10.1002/adma.202110054
- Wang M, Li W, Hao J, et al. Molecularly cleavable bioinks facilitate high-performance digital light processing-based bioprinting of functional volumetric soft tissues. Nat Commun. 2022;13(1):3317. doi: 10.1038/s41467-022-31002-2
- Kolesky DB, Homan KA, Skylar-Scott MA, Lewis JA. Three-dimensional bioprinting of thick vascularized tissues. Proc Natl Acad Sci. 2016;113:3179-3184. doi: 10.1073/pnas.1521342113
- Han X, Courseaus J, Khamassi J, et al. Optimized vascular network by stereolithography for tissue engineered skin. Int J Bioprint. 2018;4(2). doi: 10.18063/ijb.v4i2.134
- Fang Y, Ouyang L, Zhang T, Wang C, Lu B, Sun W. Optimizing bifurcated channels within an anisotropic scaffold for engineering vascularized oriented tissues. Adv Healthc Mater. 2020;9(24):2000782. doi: 10.1002/adhm.202000782
- Margolis EA, Friend NE, Rolle MW, Alsberg E, Putnam AJ. Manufacturing the multiscale vascular hierarchy: progress toward solving the grand challenge of tissue engineering. Trends Biotechnol. 2023;41(11):P1400-1416. doi: 10.1016/j.tibtech.2023.04.003
- Nascu I, Sebastia‐Saez D, Chen T, Nascu I, Du W. Global sensitivity analysis for a perfusion bioreactor based on CFD modelling. Comput Chem Eng. 2022;163:107829. doi: 10.1016/j.compchemeng.2022.107829
- Capuana E, Pavia FC, Lombardo ME, et al. Mathematical and numerical modeling of an airlift perfusion bioreactor for tissue engineering applications. Biochem Eng J. 2021;178:108298. doi: 10.1016/j.bej.2021.108298
- Zhu X, Chen F, Cao H-Q, Li L, He N, Han X. Design and fused deposition modeling of triply periodic minimal surface scaffolds with channels and hydrogel for breast reconstruction. Int J Bioprint. 2023;9(2). doi: 10.18063/ijb.685
- Allen JW, Bhatia SN. Formation of steady-state oxygen gradients in vitro: application to liver zonation. Biotechnol Bioeng. 2003;82(3):253-262. doi: 10.1002/bit.10569
- Brown DA, MacLellan WR, Laks H, Dunn JCY, Wu BM, Beygui RE. Analysis of oxygen transport in a diffusion‐limited model of engineered heart tissue. Biotechnol Bioeng. 2007;97(4):962-975. doi: 10.1002/bit.21295
- Yu P, Lee T-S, Zeng Y, Low HT. Fluid dynamics and oxygen transport in a micro-bioreactor with a tissue engineering scaffold. Int J Heat Mass Transfer. 2009;52:316-327. doi: 10.1016/j.ijheatmasstransfer.2008.06.021
- Mokhtari-Jafari F, Amoabediny G, Haghighipour N, et al. Mathematical modeling of cell growth in a 3D scaffold and validation of static and dynamic cultures. Eng Life Sci. 2016;16(3):290-298. doi: 10.1002/elsc.201500047
- Coletti F, Macchietto S, Elvassore N. Mathematical modeling of three-dimensional cell cultures in perfusion bioreactors. Ind Eng Chem Res. 2006;45:8158-8169. doi: 10.1016/S1570-7946(06)80292-0
- Nascu I, Sebastia‐Saez D, Chen T, Du W. A combined computational-fluid-dynamics model and control strategies for perfusion bioreactor systems in tissue engineering. IFAC-PapersOnLine. 2021;54(3):324-329. doi: 10.1016/j.ifacol.2021.08.262
- Han X, Bibb R, Harris RA. Design of bifurcation junctions in artificial vascular vessels additively manufactured for skin tissue engineering. J Vis Lang Comput. 2015;28:238-249. doi: 10.1016/j.jvlc.2014.12.005
- Han X, Bibb R, Harris RA. Engineering design of artificial vascular junctions for 3D printing. Biofabrication. 2016;8(2):025018. doi: 10.1088/1758-5090/8/2/025018
- Nie J, Gao Q, Xie C, et al. Construction of multi-scale vascular chips and modelling of the interaction between tumours and blood vessels. Mater Horiz. 2020;7:82-92. doi: 10.1039/C9MH01283D
- Soltani M, Maleki MA, Kaboodrangi AH, Mosadegh B. Optimization of oxygen transport within a tissue engineered vascular graft model using embedded micro-channels inspired by vasa vasorum. Chem Eng Sci. 2018;184:1-13. doi: 10.1016/j.ces.2018.02.044
- Poon C. Measuring the density and viscosity of culture media for optimized computational fluid dynamics analysis of in vitro devices. J Mech Behav Biomed Mater. 2022; 126: 105024. doi: 10.1016/j.jmbbm.2021.105024
- Haselgrove JC, Shapiro IM, Silverton SF. Computer modeling of the oxygen supply and demand of cells of the avian growth cartilage. Am J Physiol. 1993;265 2 Pt 1:C497-506. doi: 10.1152/ajpcell.1993.265.2.C497
- Acevedo CA, Weinstein-Oppenheimer CR, Brown DI, Huebner H, Buchholz R, Young ME. A mathematical model for the design of fibrin microcapsules with skin cells. Bioprocess Biosyst Eng. 2009;32:341-351. doi: 10.1007/s00449-008-0253-1
- McMahon D, Anderson PA, Nassar R, et al. C2C12 cells: biophysical, biochemical, and immunocytochemical properties. Am J Physiol. 1994;266 6 Pt 1:C1795-802. doi: 10.1152/ajpcell.1994.266.6.c1795
- Schlie S, Gruene M, Dittmar H, Chichkov BN. Dynamics of cell attachment: adhesion time and force. Tissue Eng Part C, Methods. 2012;18(9):688-696. doi: 10.1089/ten.tec.2011.0635
- Ricotti L, Taccola S, Pensabene V, et al. Adhesion and proliferation of skeletal muscle cells on single layer poly(lactic acid) ultra-thin films. Biomed Microdevices. 2010;12:809-819. doi: 10.1007/s10544-010-9435-0
- Zhou B, Yang B, Liu Q, et al. Effects of univariate stiffness and degradation of DNA hydrogels on the transcriptomics of neural progenitor cells. J Am Chem Soc. 2023;145(16):8954- 8964. doi: 10.1021/jacs.2c13373
- Jarrett AM, Lima EABF, Hormuth DA, et al. Mathematical models of tumor cell proliferation: a review of the literature. Expert Rev Anticancer Ther. 2018;18:1271-1286. doi: 10.1080/14737140.2018.1527689
- Kim K, Dean D, Mikos AG, Fisher JP. Effect of initial cell seeding density on early osteogenic signal expression of rat bone marrow stromal cells cultured on cross-linked poly(propylene fumarate) disks. Biomacromolecules. 2009;10(7):1810-1817. doi: 10.1021/bm900240k
- Yin J, Yan M, Wang Y-c, Fu J, Suo H. 3D bioprinting of low-concentration cell-laden gelatin methacrylate (GelMA) bioinks with a two-step cross-linking strategy. ACS Appl Mater Interfaces. 2018;10(8):6849-6857. doi: 10.1021/acsami.7b16059
- Cámara-Torres M, Sinha R, Mota C, Moroni L. Improving cell distribution on 3D additive manufactured scaffolds through engineered seeding media density and viscosity. Acta Biomater. 2020;101:183-195. doi: 10.1016/j.actbio.2019.11.020
- Su J, Hua S, Chen A, et al. Three-dimensional printing of gyroid-structured composite bioceramic scaffolds with tuneable degradability. Biomater Adv. 2022;133:112595. doi: 10.1016/j.msec.2021.112595