2D/3D-printed PEDOT/PSS conductive hydrogel for biomedical sensors
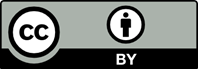
The integration of conductive hydrogels and advanced three-dimensional (3D) printing is a trigger of the development of biomedical sensors for healthcare diagnostics and personalized treatment. Poly(3,4-ethylenedioxythiophene):poly(styr ene sulfonate) (PEDOT:PSS) is a versatile conductive hydrogel materials renowned for its exceptional conductivity and hydrophilicity, and 3D printing technology allows for precise and customized fabrication of electronic components and devices. In this review, we aim to explore the potential of 3D-printed PEDOT/PSS conductive hydrogel in the fabrication of biomedical sensors, with a focus on their distinct characteristics, application potential, and systematic classification. We also discuss the methods for fabricating PEDOT:PSS hydrogel electronic devices by employing 3D printing techniques, including extrusion-based 3D printing technology (fused deposition modeling, direct ink writing, and inkjet printing), powder-based 3D printing technology (selective laser sintering and selective laser melting), and photopolymerization-based 3D printing technology (stereolithography and digital light processing). The applications of 2D/3D-printed PEDOT:PSS hydrogels in biomedical sensors, such as strain sensors, pressure sensors, stretchable sensors, electrochemical sensors, temperature sensors, humidity sensors, and electrocardiogram sensor, are also summarized in this review. Finally, we provide insights into the development of 3D-printed PEDOT:PSS-based biomedical sensors and the innovative techniques for biomedical sensor integration.
- Zhang X, Wang Y, Wu X, et al. A universal post-treatment strategy for biomimetic composite hydrogel with anisotropic topological structure and wide range of adjustable mechanical properties. Biomater Adv. 2022;133:112654. doi: 10.1016/j.msec.2022.112654
- Han IK, Chung T, Han J, Kim YS. Nanocomposite hydrogel actuators hybridized with various dimensional nanomaterials for stimuli responsiveness enhancement. Nano Converg. 2019;6(1):18. doi: 10.1186/s40580-019-0188-z
- Yumin A, Liguo D, Yi Y, Yongna J. Mechanical properties of an interpenetrating network poly(vinyl alcohol)/alginate hydrogel with hierarchical fibrous structures. RSC Adv. 2022;12(19):11632-11639. doi: 10.1039/d1ra07368k
- Zhou T, Qiao Z, Yang M, et al. Hydrogen-bonding topological remodeling modulated ultra-fine bacterial cellulose nanofibril-reinforced hydrogels for sustainable bioelectronics. Biosens Bioelectron. 2023;231:115288. doi: 10.1016/j.bios.2023.115288
- Chen ZY, Zhou RB, Wang RD, Su SL, Zhou F. Dual-crosslinked network of polyacrylamide-carboxymethylcellulose hydrogel promotes osteogenic differentiation in vitro. Inj J Biol Macromol. 2023;234:123788. doi: 10.1016/j.ijbiomac.2023.123788
- Jiang Y, Wang Y, Li Q, Yu C, Chu W. Natural polymer-based stimuli-responsive hydrogels. Curr Med Chem. 2020;27(16):2631-2657. doi: 10.2174/0929867326666191122144916
- Tang L, Wu S, Qu J, Gong L, Tang J. A review of conductive hydrogel used in flexible strain sensor. Materials (Basel). 2020;13(18):3947. doi: 10.3390/ma13183947
- Walker BW, Lara RP, Mogadam E, Yu CH, Kimball W, Annabi N. Rational design of microfabricated electroconductive hydrogels for biomedical applications. Prog Polym Sci. 2019;92:135-157. doi: 10.1016/j.progpolymsci.2019.02.007
- Chen Z, Chen Y, Hedenqvist MS, et al. Multifunctional conductive hydrogels and their applications as smart wearable devices. J Mater Chem B. 2021;9(11):2561-2583. doi: 10.1039/D0TB02929G
- Zhang W, Feng P, Chen J, Sun Z, Zhao B. Electrically conductive hydrogels for flexible energy storage systems. Prog Polym Sci. 2019;88:220-240. doi: 10.1016/j.progpolymsci.2018.09.001
- Xu J, Chen TY, Tai CH, Hsu SH. Bioactive self-healing hydrogel based on tannic acid modified gold nano-crosslinker as an injectable brain implant for treating Parkinson’s disease. Biomater Res. 2023;27(1):8. doi: 10.1186/s40824-023-00347-0
- Liu X, Miller AL, Park S, et al. Functionalized carbon nanotube and graphene oxide embedded electrically conductive hydrogel synergistically stimulates nerve cell differentiation. ACS Appl Mater Inter. 2017;9(17):14677-14690. doi: 10.1021/acsami.7b02072
- Meng Z, Stolz RM, Mendecki L, Mirica KA. Electrically-transduced chemical sensors based on two-dimensional nanomaterials. Chem Rev. 2019;119(1):478-598. doi: 10.1021/acs.chemrev.8b00311
- Gan D, Shuai T, Wang X, et al. Mussel-inspired redox-active and hydrophilic conductive polymer nanoparticles for adhesive hydrogel bioelectronics. Nano-Micro Lett. 2020;12(1):169. doi: 10.1007/s40820-020-00507-0
- Lu B, Yuk H, Lin S, et al. Pure PEDOT:PSS hydrogels. Nat Commun. 2019;10(1):1043. doi: 10.1038/s41467-019-09003-5
- Yun C, Han JW, Kim S, et al. Generating semi-metallic conductivity in polymers by laser-driven nanostructural reorganization. Mater Horiz. 2019;6(10):2143-2151. doi: 10.1039/C9MH00959K
- Won D, Kim J, Choi J, et al. Digital selective transformation and patterning of highly conductive hydrogel bioelectronics by laser-induced phase separation. Sci Adv. 2022;8(23):eabo3209. doi: 10.1126/sciadv.abo3209
- Zhang XS, Yang WT Zhang HN, Xie MG. PEDOT:PSS: From conductive polymers to sensors. Nanotechnol Precis Eng, 2021;4(4):045004. doi: 10.1063/10.0006866
- Tseghai GB, Mengistie DA, Malengier B, Fante KA, Langenhove LV. PEDOT:PSS-based conductive textiles and their applications. Sensors. 2020;20(7):1881. doi: 10.3390/s20071881
- Zhang WY, Su Z, Zhang XC, Wang WT, Li ZF. Recent progress on PEDOT-based wearable bioelectronics. View. 2022;3(5):20220030. doi: 10.1002/VIW.20220030
- Falco A, Petrelli M, Bezzeccheri E,Abdelhalim A, Lugli P. Towards 3D-printed organic electronics: Planarization and spray-deposition of functional layers onto 3D-printed objects. Org Electron. 2016;39:340-347. doi: 10.1016/j.orgel.2016.10.027
- Lee JH, So HY. 3D-printing-assisted flexible pressure sensor with a concentric circle pattern and high sensitivity for health monitoring. Microsyst Nanoeng. 2023;9:44. doi: 10.1038/s41378-023-00509-z
- Da Silva TA, Braunger ML, Countinho MAN. 3D-printed graphene electrodes applied in an impedimetric electronic tongue for soil analysis. Chemosensors. 2019;7(4):50. doi: 10.3390/chemosensors7040050
- Wu KB, Kim KW, Kwon JH, Kim JK, Kim SH, Moon HC. Direct ink writing of PEDOT:PSS inks for flexible micro-supercapacitors. J Ind Eng Chem. 2023;123:272-277. doi: 10.1016/j.jiec.2023.03.042
- Li L, Meng J, Bao XR, Huang Y. Direct-ink-write 3D printing of programmable micro-supercapacitors from MXene-regulating conducting polymer inks. Adv Energy Mater. 2023;13 (9):2203683. doi: 10.1002/aenm.202203683
- Dominguez-Alfaro A, Gabirondo E, Alegret N. 3D printable conducting and biocompatible PEDOT-graft-PLA copolymers by direct ink writing. Macromol Rapid Comm. 2021;42 (12):2100100. doi: 10.1002/marc.202100100
- Thaibunnak A, Pakdee U. Pen-based writing of functionalized MWCNT-PEDOT:PSS ink on flexible substrate for application in ammonia gas sensor. Suranaree J Sci Tech. 2022;29(2).
- Ovhal MM, Kumar N, Kang JW. 3D direct ink writing fabrication of high-performance all-solid-state micro-supercapacitors. Mol Cryst Liq Cryst. 2020;705(1):105-111. doi: 10.1080/15421406.2020.1743426
- Zheng Y, Wang YD, Zhang F, et al. Coagulation bath-assisted 3D printing of PEDOT:PSS with high resolution and strong substrate adhesion for bioelectronic devices. Adv Mater Technol-US. 2022;7(7):2101514. doi: 10.1002/admt.202101514
- Hou SY, Chen HQ, Lv D, et al. Highly conductive inkjet-printed PEDOT:PSS film under cyclic stretching. ACS Appl Mater Interfaces. 2023;15(23):28503-28515. doi: 10.1021/acsami.3c03378
- Rivers G, Austin JS, He YF, et al. Stable large area drop-on-demand deposition of a conductive polymer ink for 3D-printed electronics, enabled by bio-renewable co-solvents. Addit Manuf. 2023;66:103452. doi: 10.1016/j.addma.2023.103452
- Cinquino M, Prontera CT, Zizzari A, et al. Effect of surface tension and drying time on inkjet-printed PEDOT:PSS for ITO-free OLED devices. J Sci-Adv Mater Dev. 2022;7(1):100394. doi: 10.1016/j.jsamd.2021.09.001
- Doering OM, Vetter C, Alhawwash A, Horn MR, Yoshida K. Durable scalable 3D SLA-printed cuff electrodes with high performance carbon plus PEDOT:PSS-based contacts. Artif Organs. 2022;46(10):2085-2096. doi: 10.1111/aor.14387
- Bertana V, Scordo G, Parmeggiani M, et al. Rapid prototyping of 3D organic electrochemical transistors by composite photocurable resin. Sci Rep. 2020; 10(1):13335. doi: 10.1038/s41598-020-70365-8
- Lopez-Larrea N, Gallastegui A, Lezama L, Criado-Gonzalez M, Casado N, Mecerreyes D. Fast visible-light 3D printing of conductive PEDOT:PSS hydrogels. Macromol Rapid Comm. 2023;26:2300229. doi: 10.1002/marc.202300229
- Ye XL, Wang C, Wang L, Lu BH, Gao FL, Shao D. DLP printing of a flexible micropattern Si/PEDOT:PSS/ PEG electrode for lithium-ion batteries. Chem Comm. 2022;58(55):7642-7645. doi: 10.1039/D2CC01626E
- Park D, Lee S, Kim J. Thermoelectric and mechanical properties of PEDOT:PSS-coated Ag2Se nanowire composite fabricated via digital light processing based 3D printing. Compos Commun. 2022;30:101084. doi: 10.1016/j.coco.2022.101084
- Hill IM, Hernandez V, Xu BH. Imparting high conductivity to 3D printed PEDOT:PSS. ACS Appl Polym Mater. 2023;5(6):3989-3998. doi: 10.1021/acsapm.3c00232
- Aguzin A, Dominguez-Alfaro A, Criado-Gonzalez M, et al. Direct ink writing of PEDOT eutectogels as substrate-free dry electrodes for electromyography. Mater Horiz. 2023;10(7):2516-2524. doi: 10.1039/D3MH00310H
- Greco G, Giuri A, Bagheri S, et al. Pedot:PSS/graphene oxide (GO) ternary nanocomposites for electrochemical applications. Molecules. 2023;28(7):2963. doi: 10.3390/molecules28072963
- Bao P, Lu Y, Tao P, Liu B, Li J, Cui X. 3D printing PEDOT-CMC-based high areal capacity electrodes for Li-ion batteries. Ionics. 2021;27(7):2857-2865. doi: 10.1007/s11581-021-04063-4
- Yu JW, Tian FJ, Wang W, et al. Design of highly conductive, intrinsically stretchable, and 3D printable PEDOT:PSS hydrogels via PSS-chain engineering for bioelectronics. Chem Mater. 2023;35(15):5936-5944. doi: 10.1021/acs.chemmater.3c00844
- Kye MJ, Cho J, Yu HC, et al. “Drop-on-textile” patternable aqueous PEDOT composite ink providing highly stretchable and wash-resistant electrodes for electronic textiles. Dyes Pigments. 2018;155:150-158. doi: 10.1016/j.dyepig.2018.03.024
- Liu J, Garcia J, Leahy LM, et al. 3D printing of multifunctional conductive polymer composite hydrogels. Adv Funct Mater. 2023;2214196. doi: 10.1002/adfm.202214196
- Fan JX, Montemagno C, Gupta M. 3D printed high transconductance organic electrochemical transistors on flexible substrates. Org Electron. 2019;73:122-129. doi: 10.1016/j.orgel.2019.06.012
- Buga C, Viana JC. Optimization of print quality of inkjet printed PEDOT:PSS patterns. Flex Print Electron. 2022;7(4):045004. doi: 10.1088/2058-8585/ac931e
- Khalaf AM, Issa HH, Ramírez JL, Mohamed SA. All inkjet-printed temperature sensors based on PEDOT:PSS. IEEE Access. 2022;10:61094-61100. doi: 10.1109/ACCESS.2022.3176822
- Wang XP, Mu BY, Zhang LW, Zhang X. Drift characteristic analysis of additive manufactured Ag NPs-PEDOT:PSS flexible temperature sensor. Results Eng. 2022;13:100384. doi: 10.1016/j.rineng.2022.100384
- Khalaf AM, Ramirez JL, Mohamed SA, Issa H. Highly sensitive interdigitated thermistor based on PEDOT:PSS for human body temperature monitoring. Flex Print Electron. 2022;7(4):045012. doi: 10.1088/2058-8585/acaabc
- Jung EM, Lee SW, Kim SH. Printed ion-gel transistor using electrohydrodynamic (EHD) jet printing process. Org Electron. 2018;52:123-129. doi: 10.1016/j.orgel.2017.10.013
- Tang X, Kwon HJ, Ye HQ, et al. Enhanced solvent resistance and electrical performance of electrohydrodynamic jet printed PEDOT:PSS composite patterns: Effects of hardeners on the performance of organic thin-film transistors. Phys Chem Chem Phys. 2019;21(46):25690-25699. doi: 10.1039/C9CP04864B
- Park SH, Kim J, Lee SY, et al. Organic thin-film transistors with sub-10-micrometer channel length with printed polymer/carbon nanotube electrodes. Org Electron. 2018;52:165-171. doi: 10.1016/j.orgel.2017.10.023
- Lim S, Park SH, An TK, Lee HS, Kim SH. Electrohydrodynamic printing of poly(3,4-ethylenedioxythiophene):poly(4- styrenesulfonate) electrodes with ratio-optimized surfactant. Rsc Adv. 2016;6(3):2004-2010. doi: 10.1039/C5RA19462H
- Park SH, Kim J, Park CE. Optimization of electrohydrodynamic-printed organic electrodes for bottom-contact organic thin film transistors. Org Electron. 2016;38:48-54. doi: 10.1016/j.orgel.2016.07.040
- Chen J, Wu T, Zhang L, Tang C, Yang X. Flexible conductive patterns using electrohydrodynamic jet printing method based on high-voltage electrostatic focusing lens. Int J Adv Manuf Technol. 2023;127:4321-4329. doi: 10.1007/s00170-023-11833-z
- Chang JK, He JK, Lei Q, Li D. Electrohydrodynamic printing of microscale PEDOT:PSS-PEO features with tunable conductive/thermal properties. ACS Appl Mater Interfaces. 2018;10(22):19116-19122. doi: 10.1021/acsami.8b04051
- Balasankar A, Anbazhakan K, Arul V, et al. Recent advances in the production of pharmaceuticals using selective laser sintering. Biomimetics. 2023;8(4):330. doi: 10.3390/biomimetics8040330
- Gao BW, Zhao HJ, Peng LQ, Sun Z. A review of research progress in selective laser melting (SLM). Micromachines. 2023;14(1):57. doi: 10.3390/mi14010057
- Heo DN, Lee SJ, Timsina R, Qiu X, Castro NJ, Zhang LG. Development of 3D printable conductive hydrogel with crystallized PEDOT:PSS for neural tissue engineering. Mater Sci Eng: C. 2019;99:582-590. doi: 10.1016/j.msec.2019.02.008
- Scordo G, Bertana V, Scaltrito L, et al. A novel highly electrically conductive composite resin for stereolithography. Mater Today Commun. 2019;19:12-17. doi: 10.1016/j.mtcomm.2018.12.017
- Zhu H, Hu XC, Liu BH, Chen Z, Qu S. 3D printing of conductive hydrogel-elastomer hybrids for stretchable electronics. ACS Appl Mater Interfaces. 2021;13(49):59243-59251. doi: 10.1021/acsami.1c17526
- Borghetti M, Serpelloni M, Sardini E. Mechanical behavior of strain sensors based on PEDOT:PSS and silver nanoparticles inks deposited on polymer substrate by inkjet printing. Sensor Actuat A-Phys. 2016;243:71-80. doi: 10.1016/j.sna.2016.03.021
- Wuhao Z, Zhou P, Sun L, Liu L, Zhao MS, Yu H. Flexible wearable sensor based on graphene/PEDOT:PSS composite material. Scientia Sinica Technologica. 2019;49(7):851-860.
- Saidina DS, Mariatti M, Zubir, SA, Fontana S, Hérold C. Performance of graphene hybrid-based ink for flexibleelectronics. J Mater Sci: Mater Electron. 2019;30(22): 19906-19916. doi: 10.1007/s10854-019-02357-y
- Cruz S, Rocha LA, Viana JC. Piezo-resistive behaviour at high strain levels of PEDOT:PSS printed on a flexible polymeric substrate by a novel surface treatment. J Mater Sci: Mater Electron. 2017;28(3):2563-2573. doi: 10.1007/s10854-016-5832-3
- Tetsu Y, Yamagishi K, Kato A, et al. Ultrathin epidermal strain sensor based on an elastomer nanosheet with an inkjet-printed conductive polymer. Appl Phys Express. 2017;10(8):087201. doi: 10.7567/APEX.10.087201
- Kang TK. Inkjet printing of highly sensitive, transparent, flexible linear piezoresistive strain sensors. Coatings. 2021;11(1):51. doi: 10.3390/coatings11010051
- Li Z, Li YR, Wang ZW, et al. 3D-printable and multifunctional conductive nanocomposite with tunable mechanics inspired by sesame candy. Nano Energy. 2023;108:108116. doi: 10.1016/j.nanoen.2023.108166
- Lu Y, Yang HR, Diao YF, et al. Solution-processable PEDOT particles for coatings of untreated 3D-printed thermoplastics. ACS Appl Mater Interfaces. 2023;15(2):3433-3441. doi: 10.1021/acsami.2c18328
- Jabbar F, Soomro AM, Lee JW, et al. Robust fluidic biocompatible strain sensor based on PEDOT:PSS/CNT composite for human-wearable and high-end robotic applications. Sens Mater. 2020;32(12):4077-4093. doi: 10.18494/SAM.2020.3085
- Mirza F, Sahasrabuddhe RR, Baptist JR, Wijesundara MBJ, Lee WH, Popa D. Piezoresistive pressure sensor array for robotic skin. In: Proceedings of the Sensors for Next- Generation Robotics III, 98590K; 2016. doi: 10.1117/12.2225411
- Das SK, Baptist JR, Sahasrabuddhe R, Le WHH, Popa D. Package analysis of 3D-printed piezo-resistive strain gauge sensors. In: Proceedings of the Sensors for Next-Generation Robotics III, 985905; 2016. doi: 10.1117/12.2224352
- Shao YW, Zhang Q, Zhao Y, et al. Flexible pressure sensor with micro-structure arrays based on PDMS and PEDOT:PSS/PUD&CNTs composite film with 3D printing. Materials. 2021;14(21):6499. doi: 10.3390/ma14216499
- Karagiorgis X, Ntagios M, Skabara P, Dahiya R. Elastomeric foam-based soft capacitive pressure sensors using direct ink writing. IEEE J Flex Electron. 2023;2(2):175-182. doi: 10.1109/JFLEX.2023.3264190
- Khan S, Ali S, Khan A, Bermak A. Developing pressure sensors from impregnated textile sandwiched in inkjet-printed electrodes. J Mater Sci: Mater Electron. 2021;33(1):541-553. doi: 10.1007/s10854-021-07325-z
- Kraft U, Molina-Lopez F, Son D, Bao Z, Murmann B. Ink development and printing of conducting polymers for intrinsically stretchable interconnects and circuits. Adv Electron Mater. 2020;6(1):1900681. doi: 10.1002/aelm.201900681
- Cheng XY, Peng SQ, Wu LX, Sun QF. 3D-printed stretchable sensor based on double network PHI/PEDOT:PSS hydrogel annealed with cosolvent of H2O and DMSO. Chem Eng J. 2023;470:144058. doi: 10.1016/j.cej.2023.144058
- Lo LW, Zhao JY, Wan HC, Wang Y, Chakrabartty S, Wang C. An inkjet-printed PEDOT:PSS-based stretchable conductor for wearable health monitoring device applications. ACS Appl Mater Interfaces. 2021;13(18):21693-21702. doi: 10.1021/acsami.1c00537
- Basak I, Nowicki G, Ruttens B, et al. Inkjet printing of PEDOT:PSS based conductive patterns for 3D forming applications. Polymers. 2020;12(12):2915. doi: 10.3390/polym12122915
- Shen ZQ, Zhang ZL, Zhang NB, et al. 2022. High- Stretchability, Ultralow-Hysteresis Conducting Polymer Hydrogel Strain Sensors for Soft Machines. Adv Mater, 34 (32):2203650. doi: 10.1002/adma.202203650
- Mousavi S, Thai MT, Amjadi M, et al. Unidirectional, highly linear strain sensors with thickness-engineered conductive films for precision control of soft machines. J Mater Chem A. 2022;10(26):13673-13684. doi: 10.1039/D2TA02064E
- Kim T, Kim D, Joo Y, Park J, Yoon J, Hong Y. Crack propagation design in transparent polymeric conductive films via carbon nanotube fiber-reinforcement and its application for highly sensitive and mechanically durable strain sensors. Smart Mater Struct. 2019;28(2):025008. doi: 10.1088/1361-665X/aaf0e9
- Shao YW, Zhao YL, Liu J, et al. Flexible force sensor with micro-pyramid arrays based on 3D printing. IEEE Sens. 2018;1-4. doi: 10.1109/ICSENS.2018.8589555
- Nair RR. Glucose sensing and hybrid instrumentation based on printed organic electrochemical transistors. Flex Print Electron. 2020;5(1):015001. doi: 10.1088/2058-8585/ab63a0
- Ramli NI, Abd-Wahab MF, Salim WWAW. Characterization of enzymatic glucose biosensor in buffer solution, in artificial saliva, and in potassium ferricyanide by linear sweep voltammetry. AIP Conf Proc. 2018;2031(1):020037. doi: 10.1063/1.5066993
- Bihar E, Wustoni S, Pappa AM, Salama KN, Baran D, Inal S. A fully inkjet-printed disposable glucose sensor on paper. npj Flex Electron. 2018;2(1):30. doi: 10.1038/s41528-018-0044-y
- Khan S, Ali S, Khan A, Wang B, Bermak A. Printing sensors on biocompatible substrates for selective detection of glucose. IEEE Sens J. 2021;21(4):4167-4175. doi: 10.1109/JSEN.2020.3032539
- Nolan JK, Nguyen TNH, Le KVH, DeLong LE, Lee H. Simple fabrication of flexible biosensor arrays using direct writing for multianalyte measurement from human astrocytes. SLAS Technol. 2019;25(1):33-46. doi: 10.1177/2472630319888442
- Majak D, Fan JX, Gupta M. Fully 3D printed OECT based logic gate for detection of cation type and concentration. Sensor Actuat B-Chem. 2019:286:111-118. doi: 10.1016/j.snb.2019.01.120
- Nair RR. Organic electrochemical transistor on paper for the detection of halide anions in biological analytes. Flex Print Electron. 2020;5(4):045004. doi: 10.1088/2058-8585/abc9c9
- Cavallo A, Losi P, Buscemi M, et al. Biocompatible organic electrochemical transistor on polymeric scaffold for wound healing monitoring. Flex Print Electron. 2022;7(3):035009. doi: 10.1088/2058-8585/ac84ec
- Setti L, Fraleoni-Morgera A, Mencarelli I, Filippini A, Ballarin B, Di Biase M. An HRP-based amperometric biosensor fabricated by thermal inkjet printing. Sensor Actuat B-Chem. 2007;126(1):252-257. doi: 10.1016/j.snb.2006.12.015
- Uzuncar S, Kac H, Ak M. Electro-templating of prussian blue nanoparticles in PEDOT:PSS and soluble silkworm protein for hydrogen peroxide sensing. Talanta. 2022;252: 123841. doi: 10.1016/j.talanta.2022.123841
- Phongphut A, Sriprachuabwong C, Wisitsoraat A, Tuantranont A, Prichanont A, Sritongkham P. A disposable amperometric biosensor based on inkjet-printed Au/ PEDOT-PSS nanocomposite for triglyceride determination. Sensor Actuat B-Chem. 2013;178:501-507. doi: 10.1016/j.snb.2013.01.012
- Beduk T, Bihar E, Surya SG, Castillo AN, Inal S, Salama KN. A paper-based inkjet-printed PEDOT:PSS/ ZnO sol-gel hydrazine sensor. Sensor Actuat B-Chem. 2020;306:127539. doi: 10.1016/j.snb.2019.127539
- Zeng RJ, Wang WJ, Chen MM, et al. CRISPR-Cas12a-driven MXene-PEDOT:PSS piezoresistive wireless biosensor. Nano Energy. 2021;82:105711. doi: 10.1016/j.nanoen.2020.105711
- Majak D, Fan JX, Kang S, Gupta M. Delta-9- tetrahydrocannabinol (Delta(9)-THC) sensing using an aerosol jet printed organic electrochemical transistor (OECT). J Mater Chem B. 2021;9(8):2107-2117. doi: 10.1039/D0TB02951C
- Amr AGE, Kamel AH, Almehizia AA, Sayed AYA, Elsayed EA, Abd-Rabboh HSM. Paper-based potentiometric sensors for nicotine determination in smokers’ sweat. ACS Omega. 2021;6(17):11340-11347. doi: 10.1021/acsomega.1c00301
- Kuzubasoglu BA, Sayar E, Bahadir SK. Inkjet-printed CNT/ PEDOT:PSS temperature sensor on a textile substrate for wearable intelligent systems. IEEE Sens J. 2021;21(12): 13090-13097. doi: 10.1109/JSEN.2021.3070073
- Soni M, Bhattacharjee M, Ntagio M, Dahiya R. Printed temperature sensor based on PEDOT: PSS-graphene oxide composite. IEEE Sens J. 2020;20(14):7525-7531. doi: 10.1109/JSEN.2020.2969667
- Wang YF, Sekine T, Takeda Y., et al. Fully printed PEDOT:PSS-based temperature sensor with high humidity stability for wireless healthcare monitoring. Sci Rep. 2020;10(1):2467. doi: 10.1038/s41598-020-59432-2
- Aidit SN, Rezali FAM, Nor NHM, Yusoff N. Hydrothermal synthesis of zinc oxide/PEDOT:PSS composite for flexible temperature sensor application. Flex Print Electron. 2023;8(2):025008. doi: 10.1088/2058-8585/acd06e
- Wang P, Yu W, Li GX, Meng C, Guo S. Printable, flexible, breathable and sweatproof bifunctional sensors based on an all-nanofiber platform for fully decoupled pressure-temperature sensing application. Chem Eng J. 2022;452:139174. doi: 10.1016/j.cej.2022.139174
- Guo H, Song J, Zhang X. Research and fabrication and test of flexible temperature sensor based on fabric substrate. Transduc Microsyst Technol. 2022;41(6):86-89,93.
- Lee JW, Choi Y, Jiang J, Yeom SH, Lee W, Ju BK. High sensitivity flexible paper temperature sensor and body-attachable patch for thermometers. Sensor Actuat A-Phys. 2020;313:112205. doi: 10.1016/j.sna.2020.112205
- Roy AL, Beaumont C, Leclerc M. Evaluating polythiophenes as temperature sensing materials using combinatorial inkjet printing. Flex Print Electron. 2023;8(1):014002. doi: 10.1088/2058-8585/acc07f
- Pradhan S, Yadavalli VK. Photolithographically printed flexible silk/PEDOT:PSS temperature sensors. ACS Appl Electron Mater. 2021;3(1):21-29. doi: 10.1021/acsaelm.0c01017
- Bucher T, Huber R, Eschenbaum C, Mertens A, Lemmer U, Amrouch H. Printed temperature sensor array for high-resolution thermal mapping. Sci Rep. 2022;12(1):14231. doi: 10.1038/s41598-022-18321-6
- Wang G, Zhang Y, Yang H. Fast-response humidity sensor based on laser printing for respiration monitoring. RSC Adv. 2020;10(15):8910-8916. doi: 10.1039/C9RA10409G
- Galliani M, Ferrari LM, Ismailova E. Interdigitated organic sensor in multimodal facemask’s barrier integrity and wearer’s respiration monitoring. Biosensors. 2022;12(5):305. doi: 10.3390/bios12050305
- Tekcin M, Sayar E, Yalcin MK. Wearable and flexible humidity sensor integrated to disposable diapers for wetness monitoring and urinary incontinence. Electronics. 2022;11(7):1025. doi: 10.3390/electronics11071025
- Hassan G, Sajid M, Choi C. Highly sensitive and full range detectable humidity sensor using PEDOT:PSS, methyl red and graphene oxide materials. Sci Rep. 2019;9: 15227. doi: 10.1038/s41598-019-51712-w
- Siddiqui GU, Sajid M, Ali J. Wide range highly sensitive relative humidity sensor based on series combination of MoS2 and PEDOT:PSS sensors array. Sensor Actuat B-Chem. 2018;266:354-363. doi: 10.1016/j.snb.2018.03.134
- Conti S, Nepa F, Pascoli SD. Hybrid flexible NFC sensor on paper. IEEE J Flex Electron. 2023;2(1):4-10. doi: 10.1109/JFLEX.2023.3238682
- Barmpakos D, Tsamis C, Kaltsas G. Multi-parameter paper sensor fabricated by inkjet-printed silver nanoparticle ink and PEDOT:PSS. Microelectron Eng. 2020;225: 111266. doi: 10.1016/j.mee.2020.111266
- Xu SY, Li TY, Ren HX, Mao X, Ye X, Liang B. PEDOT:PSS hydrogel based flexible electrodes for wearable ECG monitoring. IEEE Sensors. 2020;20242466.
- Achilli A, Bonfiglio A, Pani D. Design and characterization of screen-printed textile electrodes for ECG monitoring. IEEE Sens J. 2018;18(10):4097-4107.
- Sinha SK, Noh Y, Reljin N, et al. Screen-printed PEDOT:PSS electrodes on commercial finished textiles for electrocardiography. ACS Appl Mater Interfaces. 2017;9(43):37524-37528. doi: 10.1021/acsami.7b09954
- Zalar P, Saalmink M, Raiteri D, van den Brand J, Smits ECP. Screen-printed dry electrodes: basic characterization and benchmarking. Adv Eng Mater. 2020;22(11):2000714. doi: 10.1002/adem.202000714
- Shathi MA, Chen MZ, Khoso NA, Deb H, Ahmed A, Sai WS. All organic graphene oxide and Poly (3, 4-ethylene dioxythiophene) - Poly (styrene sulfonate) coated knitted textile fabrics for wearable electrocardiography (ECG) monitoring. Synth Met. 2020;263:116329. doi: 10.1016/j.synthmet.2020.116329
- Li TY, Liang B, Ye ZC, et al. An integrated and conductive hydrogel-paper patch for simultaneous sensing of Chemical-Electrophysiological signals. Biosens Bioelectron. 2021;198:113855. doi: 10.1016/j.bios.2021.113855
- Picchio ML, Gallastegui A, Casado N, et al. Mixed ionic and electronic conducting eutectogels for 3D-printable wearable sensors and bioelectrodes. Adv Mater Technol. 2022;7(10):2101680. doi: 10.1002/admt.202101680
- Lidon-Roger JV, Prats-Boluda G, Ye-Lin Y, Garcia-Casado J, Garcia-Breijo E. Textile concentric ring electrodes for ECG recording based on screen-printing technology. Sensors. 2018;18(1):300. doi: 10.3390/s18010300
- Pless CJ, Nikzad S, Papiano I, et al. Soft electronic block copolymer elastomer composites for multi-material printing of stretchable physiological sensors on textiles. Adv Eng Mater. 2023;9(5):2201173. doi: 10.1002/aelm.202201173