3D-bioprinted cell-laden hydrogel with anti-inflammatory and anti-bacterial activities for tracheal cartilage regeneration and restoration
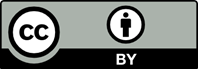
Despite the notable advances in tissue-engineered tracheal cartilage (TETC), there remain several challenges that need to be addressed, such as uneven cell distribution for cartilage formation, customized C-shaped tracheal morphology, local inflammatory reactions, and infections. To overcome these challenges, this study proposed the addition of icariin (ICA) and chitosan (CS) into a gelatin methacryloyl (GelMA) hydrogel to develop a new ICA/CS/GelMA hydrogel with anti-inflammatory and anti-bacterial properties, and three-dimensional (3D)-bioprinting feasibility. The aim of this study was to construct a TETC, a customized C-shaped cartilage structure, with uniform chondrocyte distribution as well as anti-inflammatory and anti-bacterial functions. Our results confirmed that ICA/CS/GelMA hydrogel provides desirable rheological properties, suitable printability, favorable biocompatibility, and simulated microenvironments for chondrogenesis. Moreover, the addition of ICA stimulated chondrocyte proliferation, extracellular matrix synthesis, and anti-inflammatory ability, while the encapsulation of CS enhanced the hydrogels’ anti-bacterial ability. All these led to the formation of an enhanced TETC after submuscular implantation and an elevated survival rate of experimental rabbits after orthotopic tracheal transplantation. This study provides a reliable cell-laden hydrogel with anti-inflammatory and anti-bacterial activities, suitable printability, and significant advancements in in vivo cartilage regeneration and in situ tracheal cartilage restoration.
- Xu Y, Li D, Yin ZQ, et al. Tissue-engineered trachea regeneration using decellularized trachea matrix treated with laser micropore technique. Acta Biomater. 2017;58: 113–121. doi: 10.1016/j.actbio.2017.05.010
- Xu Y, Duan H, Li YQ, et al. Nanofibrillar decellularized Wharton’s jelly matrix for segmental tracheal repair. Adv Funct Mater. 2020;30(14): 1910067. doi: 10.1002/adfm.201910067
- Xu Y, Dai J, Zhu XS, et al. Biomimetic trachea engineering via a modular ring strategy based on bone-marrow stem cells and atelocollagen for use in extensive tracheal reconstruction. Adv Mater. 2022;34(6): 2106755. doi: 10.1002/adma.202106755
- Gao E, Li G, Cao RF, et al. Bionic tracheal tissue regeneration using a ring-shaped scaffold comprised of decellularized cartilaginous matrix and silk fibroin. Compos Part B-Eng. 2022;229: 109470. doi: 10.1016/j.compositesb.2021.109470
- Xu Y, Guo Y, Li Y, et al. Biomimetic trachea regeneration using a modular ring strategy based on poly(sebacoyl diglyceride)/polycaprolactone for segmental trachea defect repair. Adv Funct Mater. 2020;30(42): 2004276. doi: 10.1002/adfm.202004276
- Gao ER, Wang Y, Wang PL, et al. C-shaped cartilage development using Wharton’s jelly-derived hydrogels to assemble a highly biomimetic neotrachea for use in circumferential tracheal reconstruction. Adv Funct Mater. 2023;33(14) 2212830. doi: 10.1002/adfm.202212830
- Hong H, Seo YB, Kim DY, et al. Digital light processing 3D printed silk fibroin hydrogel for cartilage tissue engineering. Biomaterials. 2020;232: 119679. doi: 10.1016/j.biomaterials.2019.119679
- Kandi R, Sachdeva K, Choudhury SD, Pandey PM, Mohanty S. A facile 3D bio-fabrication of customized tubular scaffolds using solvent-based extrusion printing for tissue-engineered tracheal grafts. J Biomed Mater Res A. 2023;111(2): 278–293. doi: 10.1002/jbm.a.37458
- Yang ML, Sun WY, Wang L, Tang H. Curcumin loaded polycaprolactone scaffold capable of anti-inflammation to enhance tracheal cartilage regeneration. Mater Design. 2022;224: 111299. doi: 10.1016/j.matdes.2022.111299
- Bush A, Floto RA. Pathophysiology, causes and genetics of paediatric and adult bronchiectasis. Respirology. 2019;24(11): 1053–1062. doi: 10.1111/resp.13509
- Kolwijck E, van de Veerdonk FL. The potential impact of the pulmonary microbiome on immunopathogenesis of Aspergillus-related lung disease. Eur J Immunol. 2014;44(11): 3156–3165. doi: 10.1002/eji.201344404
- Dhasmana A, Singh A, Rawal S. Biomedical grafts for tracheal tissue repairing and regeneration “Tracheal tissue engineering: an overview”. J Tissue Eng Regen Med. 2020;14(5): 653–672. doi: 10.1002/term.3019
- Reynolds PM, Holzmann Rasmussen C, Hansson M, Dufva M, Riehle MO, Gadegaard N. Controlling fluid flow to improve cell seeding uniformity. PLoS One. 2018;13(11): e0207211. doi: 10.1371/journal.pone.0207211
- Xu Y, Wang Z, Hua Y, et al. Photocrosslinked natural hydrogel composed of hyaluronic acid and gelatin enhances cartilage regeneration of decellularized trachea matrix. Mater Sci Eng C Mater Biol Appl. 2021;120: 111628. doi: 10.1016/j.msec.2020.111628
- Xu Y, Li Y, Liu Y, et al. Surface modification of decellularized trachea matrix with collagen and laser micropore technique to promote cartilage regeneration. Am J Transl Res. 2019;11(9): 5390–5403.
- Lazaridou M, Bikiaris DN, Lamprou DA. 3D bioprinted chitosan-based hydrogel scaffolds in tissue engineering and localised drug delivery. Pharmaceutics. 2022;14(9): 1978. doi: 10.3390/pharmaceutics14091978
- Park JH, Ahn M, Park SH, et al. 3D bioprinting of a trachea-mimetic cellular construct of a clinically relevant size. Biomaterials. 2021;279: 121246. doi: 10.1016/j.biomaterials.2021.121246
- Huo Y, Xu Y, Wu X, et al. Functional trachea reconstruction using 3D-bioprinted native-like tissue architecture based on designable tissue-specific bioinks. Adv Sci (Weinh). 2022;9(29): e2202181. doi: 10.1002/advs.202202181
- Liu Y, Li D, Yin Z, et al. Prolonged in vitro precultivation alleviates post-implantation inflammation and promotes stable subcutaneous cartilage formation in a goat model. Biomed Mater. 2016;12(1): 015006. doi: 10.1088/1748-605X/12/1/015006
- Luo X, Zhou G, Liu W, et al. In vitro precultivation alleviates post-implantation inflammation and enhances development of tissue-engineered tubular cartilage. Biomed Mater. 2009;4(2): 025006. doi: 10.1088/1748-6041/4/2/025006
- Gao E, Wang P, Chen F, et al. Skin-derived epithelial lining facilitates orthotopic tracheal transplantation by protecting the tracheal cartilage and inhibiting granulation hyperplasia. Biomater Adv. 2022;139: 213037. doi: 10.1016/j.bioadv.2022.213037
- Jungebluth P, Moll G, Baiguera S, Macchiarini P. Tissue-engineered airway: a regenerative solution. Clin Pharmacol Ther. 2012;91(1): 81–93. doi: 10.1038/clpt.2011.270
- Dikina AD, Strobel HA, Lai BP, Rolle MW, Alsberg E. Engineered cartilaginous tubes for tracheal tissue replacement via self-assembly and fusion of human mesenchymal stem cell constructs. Biomaterials. 2015;52: 452–462. doi: 10.1016/j.biomaterials.2015.01.073
- Lei D, Luo B, Guo YF, et al. 4-Axis printing microfibrous tubular scaffold and tracheal cartilage application. Sci China Mater. 2019;62(12): 1910–1920. doi: 10.1007/s40843-019-9498-5
- Cao Y. Icariin alleviates MSU-induced rat GA models through NF-kappaB/NALP3 pathway. Cell Biochem Funct. 2021;39(3): 357–366. doi: 10.1002/cbf.3598
- Mi B, Wang J, Liu Y, et al. Icariin activates autophagy via down-regulation of the NF-kappaB signaling-mediated apoptosis in chondrocytes. Front Pharmacol. 2018;9: 605. doi: 10.3389/fphar.2018.00605
- Oprita EI, Iosageanu A, Craciunescu O. Progress in composite hydrogels and scaffolds enriched with icariin for osteochondral defect healing. Gels. 2022;8(10): 648. doi: 10.3390/gels8100648
- Qi W, Dong N, Wu L, et al. Promoting oral mucosal wound healing using a DCS-RuB(2)A(2) hydrogel based on a photoreactive antibacterial and sustained release of BMSCs. Bioact Mater. 2023;23: 53–68. doi: 10.1016/j.bioactmat.2022.10.027
- Xiang L, Cui W. Biomedical application of photo-crosslinked gelatin hydrogels. J Leather Sci Eng. 2021;3(1): 3. doi: 10.1186/s42825-020-00043-y
- Zhang M, Zhu JY, Qin X, et al. Cardioprotection of tetrahedral DNA nanostructures in myocardial ischemia-reperfusion injury. Acs Appl Mater Inter. 2019;11(34): 30631–30639. doi: 10.1021/acsami.9b10645
- Xu Y, Guo Z, Liu R, et al. Bioengineered carina reconstruction using in-vivo bioreactor technique in human: Proof of concept study. Transl Lung Cancer Res. 2020;9(3): 705–712. doi: 10.21037/tlcr-20-534
- Murphy MP, Koepke LS, Lopez MT, et al. Articular cartilage regeneration by activated skeletal stem cells. Nat Med. 2020;26(10): 1583. doi: 10.1038/s41591-020-1013-2
- Wu CL, Harasymowicz NS, Klimak MA, Collins KH, Guilak F. The role of macrophages in osteoarthritis and cartilage repair. Osteoarthr Cartilage. 2020;28(5): 544–554. doi: 10.1016/j.joca.2019.12.007
- Koh RH, Jin Y, Kim J, Hwang NS. Inflammation-modulating hydrogels for osteoarthritis cartilage tissue engineering. Cells. 2020;9(2): 419. doi: 10.3390/cells9020419
- Hamilton N, Bullock AJ, Macneil S, Janes SM, Birchall M. Tissue engineering airway mucosa: A systematic review. Laryngoscope. 2014;124(4): 961–968. doi: 10.1002/lary.24469
- Wypych TP, Wickramasinghe LC, Marsland BJ. The influence of the microbiome on respiratory health. Nat Immunol. 2019;20(10): 1279–1290. doi: 10.1038/s41590-019-0451-9
- Mindt BC, DiGiandomenico A. Microbiome modulation as a novel strategy to treat and prevent respiratory infections. Antibiotics (Basel).2022; 11(4): 474. doi: 10.3390/antibiotics11040474
- Maurizi E, Adamo D, Magrelli FM, et al. Regenerative medicine of epithelia: Lessons from the past and future goals. Front Bioeng Biotechnol. 2021;9: 652214. doi: 10.3389/fbioe.2021.652214
- Rahimnejad M, Adoungotchodo A, Demarquette NR, Lerouge S. FRESH bioprinting of biodegradable chitosan thermosensitive hydrogels. Bioprinting. 2022;27: e00209. doi: 10.1016/j.bprint.2022.e00209
- Zhu Y, Ye L, Cai X, Li Z, Fan Y, Yang F. Icariin-loaded hydrogel regulates bone marrow mesenchymal stem cell chondrogenic differentiation and promotes cartilage repair in osteoarthritis. Front Bioeng Biotechnol. 2022;10: 755260. doi: 10.3389/fbioe.2022.755260
- Luo X, Liu Y, Zhang Z, et al. Long-term functional reconstruction of segmental tracheal defect by pedicled tissue-engineered trachea in rabbits. Biomaterials. 2013;34(13): 3336–3344. doi: 10.1016/j.biomaterials.2013.01.060