3D-printed hydrogels dressings with bioactive borate glass for continuous hydration and treatment of second-degree burns
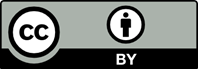
Recent advances in additive manufacturing have led to the development of innovative solutions for tissue regeneration. Hydrogel materials have gained significant attention for burn wound treatment in clinical practice among various advanced dressings due to their soothing and moisturizing activity. However, prolonged healing, pain, and traumatic removal due to the lack of long-term wound hydration are some of the challenges in the treatment of second-degree burn wounds. In this study, 3D-printed dressings were fabricated using gelatin, alginate, and bioactive borate glass (BBG) using an extrusion-based bioprinter. After ionic crosslinking, the 3D-printed dressings were characterized for mechanical properties, degradation rate, hydration activity, and in vitro cell viability using human fibroblasts. The results demonstrated that in 3D-printed dressings with 20 wt% BBG, Young’s modulus increased by 105%, and 10-day degradation rate decreased by 62%. Addition of BBG prevented the burst release of water from hydrogel dressings and enabled the continuous water release for up to 10 days, which is crucial in treating second-degree burn wounds. 3D-printed hydrogel dressings with BBG showed long-term cell viability that can be a result of the accumulative release of therapeutic ions from BBG particulate. The in vivo wound healing functionality of the dressings was investigated using a rat model with a second-degree burn wound. Our animal study showed that the 3D-printed dressings with BBG exhibited faster wound closure, non-adhesive contact, non-invasive debridement, and non-traumatic dressing removal. Histological analysis suggested that 3D-printed dressings contributed to more uniform re-epithelialization and tissue remodeling compared to the non-printed hydrogels of the same compositions. Critically, 3D-printed dressings with BBG led to significant regeneration of hair follicles compared to the 3D-printed hydrogel, non-printed hydrogel, and the control groups. The superior outcome of the 3D-printed hydrogel–BBG20 dressings can be attributed to the bioactive formulation, which promotes moist wound healing for longer time periods, and the non-adhesive porous texture of the 3D-printed dressings with increased wound-dressing interactions. Our findings provided proof of concept for the synergistic effect of bioactive formulation and the porous texture of the 3D-printed hydrogel dressings incorporated with BBG on continuous water release and, consequently, on second-degree burn wound healing.
1. Greenhalgh DG, 2019, Management of burns. N Engl J Med, 380(24): 2349–2359.
2. Stoica AE, Chircov C, Grumezescu AM, 2020, Hydrogel dressings for the treatment of burn wounds: An up-to-date overview. Materials, 13(12): 2853.
3. Centers for Disease Control and Prevention. U.S. Department of Health and Human Services, Burns: Fact Sheet. 2004. https://www.cdc.gov/masstrauma/factsheets/ public/burns.pdf.
4. Zavlin D, Chegireddy V, Boukovalas S, et al., 2018, Multi-institutional analysis of independent predictors for burn mortality in the United States. Burns Trauma, 6: 24–34.
5. Yu T-C, Zhang X, Smiell J, et al., 2020, Healthcare resource utilization, treatment patterns, and cost of care among patients with thermal burns and inpatient autografting in two large privately insured populations in the United States. Burns, 46(4): 825–835.
6. Sen CK, 2019, Human Wounds and Its Burden: An Updated Compendium of Estimates. Mary Ann Liebert, Inc., New York, United States 39–48.
7. Kordestani SS, 2019, Atlas of Wound Healing-E-Book: A Tissue Regeneration Approach, Elsevier, Missouri, United States.
8. Middelkoop E, Sheridan RL, 2018, 15—Skin substitutes and ‘the next level’. In: Herndon DN, editor. Total Burn Care (Fifth Edition), Elsevier, 167–173.e162, Amsterdam, Netherlands.
9. Hayes TR, Su B, 2011, 15—Wound dressings. In: Bosworth LA, Downes S, editors. Electrospinning for Tissue Regeneration, Woodhead Publishing, 317–339, Sawston, United Kingdom.
10. Rogers AD, Jeschke MG, 2016, Managing severe burn injuries: Challenges and solutions in complex and chronic wound care. Chronic Wound Care Manage Res, 3: 59–71.
11. Kowal S, Kruger E, Bilir P, et al., 2019, Cost-effectiveness of the use of autologous cell harvesting device compared to standard of care for treatment of severe burns in the United States. Adv Ther, 36(7): 1715–1729.
12. Zheng W, Zhao D-L, Zhao Y-Q, et al., 2022, Effectiveness of platelet rich plasma in burn wound healing: A systematic review and meta-analysis. J Dermatol Treatment, 33(1): 131–137.
13. Dhaliwal K, Lopez N, 2018, Hydrogel dressings and their application in burn wound care. Br J Commun Nurs, 23(Suppl 9): S24–S27.
14. Bazaliński D, Karnas M, Wołkowicz M, et al., 2018, ZASTOSOWANIE LARW LUCILIA SERICATA W OCZYSZCZANIU RAN PRZEWLEKŁYCH-OPIS TRZECH PRZYPADKÓW. Leczenie Ran, 15(3): 153–159.
15. Wang Y, Beekman J, Hew J, et al., 2018, Burn injury: Challenges and advances in burn wound healing, infection, pain and scarring. Adv Drug Deliv Rev, 123: 3–17.
16. Fayyazbakhsh F, Khayat MJ, Leu MC, 2022, 3D-printed gelatin-alginate hydrogel dressings for burn wound healing: A comprehensive study. Int J Bioprint, 8(4): 274–291.
17. Kordestani SS, 2019, Atlas of Wound Healing: A Tissue Engineering Approach, Elsevier Health Sciences, Missouri, United States.
18. Rowan MP, Cancio LC, Elster EA, et al., 2015, Burn wound healing and treatment: Review and advancements. Crit Care, 19(1): 243.
19. Shpichka A, Butnaru D, Bezrukov EA, et al., 2019, Skin tissue regeneration for burn injury. Stem Cell Res Ther, 10(1): 94.
20. Sood A, Granick MS, Tomaselli NL, 2014, Wound dressings and comparative effectiveness data. Adv Wound Care, 3(8): 511–529. https://doi.org/10.1089/wound.2012.0401
21. Huangfu Y, Li S, Deng L, et al., 2021, Skin-adaptable, long-lasting moisture, and temperature-tolerant hydrogel dressings for accelerating burn wound healing without secondary damage. ACS Appl Mater Interfaces, 13(50): 59695–59707.
22. Szymański K, Waś J, 2014, POTENCJALNE MOŻLIWOŚCI LECZENIA I REGENERACJI SKÓRY W ROZLEGŁYCH RANACH OPARZENIOWYCH PRZY ZASTOSOWANIU SUBSTYTUTÓW REGENERUJĄCYCH SKÓRĘ. Leczenie Ran, 11(1): 1338–1363.
23. Selig HF, Lumenta DB, Giretzlehner M, et al., 2012, The properties of an “ideal” burn wound dressing: What do we need in daily clinical practice? Results of a worldwide online survey among burn care specialists. Burns, 38(7): 960–966.
24. Markiewicz-Gospodarek A, Kozioł M, Tobiasz M, et al., 2022, Burn wound healing: Clinical complications, medical care, treatment, and dressing types: The current state of knowledge for clinical practice. Int J Environ Res Public Health, 19(3): 1338.
25. Zheng K, Kapp M, Boccaccini AR, 2019, Protein interactions with bioactive glass surfaces: A review. Appl Mater Today, 15: 350–371.
26. Mehrabi T, Mesgar AS, Mohammadi Z, 2020, Bioactive glasses: A promising therapeutic ion release strategy for enhancing wound healing. ACS Biomater Sci Eng, 6(10): 5399–5430.
27. Solanki AK, Lali FV, Autefage H, et al., 2021, Bioactive glasses and electrospun composites that release cobalt to stimulate the HIF pathway for wound healing applications. Biomater Res, 25(1): 1. https://doi.org/10.1186/s40824-020- 00202-6.
28. Chen Y-H, Rao Z-F, Liu Y-J, et al., 2021, Multifunctional injectable hydrogel loaded with cerium-containing bioactive glass nanoparticles for diabetic wound healing. Biomolecules, 11(5): 702.
29. S. Food and Drug Administration. U.S. Department of Health and Human Services, Establishment Registration & Device Listing, Mirragen Advanced Wound Matrix, 510(k) Premarket Notification. https://www.accessdata.fda.gov/ cdrh_docs/pdf16/K161067.pdf.
30. Ege D, Zheng K, Boccaccini AR, 2022, Borate bioactive glasses (BBG): Bone regeneration, wound healing applications, and future directions. ACS Appl Bio Mater, 5(8): 3608–3622. https://doi.org/10.1021/acsabm.2c00384
31. Bi L, Rahaman MN, Day DE, et al., 2013, Effect of bioactive borate glass microstructure on bone regeneration, angiogenesis, and hydroxyapatite conversion in a rat calvarial defect model. Acta Biomater, 9(8): 8015–8026.
32. Lin Y, Brown RF, Jung SB, et al., 2014, Angiogenic effects of borate glass microfibers in a rodent model. J Biomed Mater Res Part A, 102(12): 4491–4499.
33. Zhao S, Li L, Wang H, et al., 2015, Wound dressings composed of copper-doped borate bioactive glass microfibers stimulate angiogenesis and heal full-thickness skin defects in a rodent model. Biomaterials, 53: 379–391.
34. Jung SB, 2010, Borate based bioactive glass scaffolds for hard and soft tissue engineering. Doctoral Dissertation, Missouri University of Science and Technology. https://scholarsmine. mst.edu/doctoral_dissertations/2075
35. Jung S, 2013, Treatment of Chronic Wounds With Bioactive Borate Glass Fibers. An Introduction to Bioceramics, World Scientific, Tuck Link, Singapore, 487–493.
36. Hu H, Tang Y, Pang L, et al., 2018, Angiogenesis and full-thickness wound healing efficiency of a copper-doped borate bioactive glass/poly (lactic-co-glycolic acid) dressing loaded with vitamin E in vivo and in vitro. ACS Appl Mater Interfaces, 10(27): 22939–22950.
37. Zhou J, Wang H, Zhao S, et al., 2016, In vivo and in vitro studies of borate based glass micro-fibers for dermal repairing. Mater Sci Eng, C, 60: 437–445.
38. Ottomeyer M, Mohammadkah A, Day D, et al., 2016, Broad-spectrum antibacterial characteristics of four novel borate-based bioactive glasses. Adv Microbiol, 6(10): 776–787.
39. Thyparambil NJ, Gutgesell LC, Bromet BA, et al., 2020, Bioactive borate glass triggers phenotypic changes in adipose stem cells. J Mater Sci Mater Med, 31(4): 1–15.
40. Mouriño V, Vidotto R, Cattalini J, et al., 2019, Enhancing biological activity of bioactive glass scaffolds by inorganic ion delivery for bone tissue engineering. Curr Opin Biomed Eng, 10: 23–34.
41. Ramos Rivera C, 2020, Development of HIF-Mimicking Materials for Chronic Wound Healing, University College London, London, United Kingdom.
42. Schuhladen K, Stich L, Schmidt J, et al., 2020, Cu, Zn doped borate bioactive glasses: Antibacterial efficacy and dose-dependent in vitro modulation of murine dendritic cells. Biomater Sci, 8(8): 2143–2155.
43. Chebassier N, Ouijja EH, Viegas I, et al., 2004, Stimulatory effect of boron and manganese salts on keratinocyte migration. Acta Dermatovenereol, 84(3): 191–194.
44. Ege D, Zheng K, Boccaccini AR, 2022, Borate bioactive glasses (BBG): Bone regeneration, wound healing applications, and future directions. ACS Appl Biol Mater, 5(8): 3608–3622. https://doi.org/10.1021/acsabm.2c00384
45. Salvo J, Sandoval C, 2022, Role of copper nanoparticles in wound healing for chronic wounds: Literature review. Burns Trauma, 10: tkab047 (1–9).
46. Mutlu N, Kurtuldu F, Unalan I, et al., 2022, Effect of Zn and Ga doping on bioactivity, degradation, and antibacterial properties of borate 1393-B3 bioactive glass. Ceram Int, 48(11): 16404–16417.
47. Kermani F, Nazarnezhad S, Mollaei Z, et al., 2023, Zinc-and copper-doped mesoporous borate bioactive glasses: Promising additives for potential use in skin wound healing applications. Int J Mol Sci, 24(2): 1304.
48. Lin P-H, Sermersheim M, Li H, et al., 2017, Zinc in wound healing modulation. Nutrients, 10(1): 16.
49. Balasubramanian P, Grünewald A, Detsch R, et al., 2016, Ion release, hydroxyapatite conversion, and cytotoxicity of boron‐containing bioactive glass scaffolds. Int J Appl Glass Sci, 7(2): 206–215.
50. Chai Q, Jiao Y, Yu X, 2017, Hydrogels for biomedical applications: Their characteristics and the mechanisms behind them. Gels, 3(1): 6.
51. Fayyazbakhsh F, Amato M, Khayat MJ, et al., editors, 2022, In-vivo evaluation of 3D printed hydrogel wound dressings for burn wound healing. Tissue Eng Part A, 28(S1):S527–S538.
52. Zhu Y, Ma Z, Kong L, et al., 2020, Modulation of macrophages by bioactive glass/sodium alginate hydrogel is crucial in skin regeneration enhancement. Biomaterials, 256: 120216.
53. Manning JH, Stark JH, 1977, Diester crosslinked polyglucan hydrogels and reticulated sponges thereof. Google Patents.
54. Kamoun EA, Kenawy E-RS, Chen X, 2017, A review on polymeric hydrogel membranes for wound dressing applications: PVA-based hydrogel dressings. J Adv Res, 8(3): 217–233.
55. Li J, Mooney DJ, 2016, Designing hydrogels for controlled drug delivery. Nat Rev Mater, 1(12): 1–17.
56. Bruggeman KF, Williams RJ, Nisbet DR, 2018, Dynamic and responsive growth factor delivery from electrospun and hydrogel tissue engineering materials. Adv Healthc Mater, 7(1): 1700836.
57. Zhu J, Jiang G, Song G, et al., 2019, Incorporation of ZnO/bioactive glass nanoparticles into alginate/chitosan composite hydrogels for wound closure. ACS Appl Biol Mater, 2(11): 5042–5052.
58. Mehrabi A, Karimi A, Mashayekhan S, et al., 2022, In-situ forming hydrogel based on thiolated chitosan/ carboxymethyl cellulose (CMC) containing borate bioactive glass for wound healing. Int J Biol Macromol, 222: 620–635.
59. Yang Z, Ren X, Liu Y, 2021, Multifunctional 3D printed porous GelMA/xanthan gum based dressing with biofilm control and wound healing activity. Mater Sci Eng C, 131: 112493.
60. Pirayavaraporn C, Rades T, Tucker IG, 2012, Determination of moisture content in relation to thermal behaviour and plasticization of Eudragit RLPO. Int J Pharmaceut, 422(1-2): 68–74.
61. Martineau L, Shek PN, 2006, Evaluation of a bi-layer wound dressing for burn care: I. Cooling and wound healing properties. Burns, 32(1): 70–76. https://doi.org/10.1016/j. burns.2005.08.009.
62. Rice P, Orgill DP, 2008, Emergency care of moderate and severe thermal burns in adults. UpToDate, Wolters Kluwer Marx JA, Grayzel J, eds. https://www.uptodate. com/contents/emergency-care-of-moderate-and-severe-thermal-burns-in-adults
63. Schaefer TJ, Lopez ON, 2020, Burn resuscitation and management. StatPearls [Internet].
64. Singhvi G, Singh M, 2011, In-vitro drug release characterization models. Int J Pharm Stud Res, 2(1): 77–84.
65. Altavilla D, Saitta A, Cucinotta D, et al., 2001, Inhibition of lipid peroxidation restores impaired vascular endothelial growth factor expression and stimulates wound healing and angiogenesis in the genetically diabetic mouse. Diabetes, 50(3): 667–674.
66. Koehler J, Brandl FP, Goepferich AM, 2018, Hydrogel wound dressings for bioactive treatment of acute and chronic wounds. Eur Polym J, 100: 1–11.
67. Pawlaczyk M, Lelonkiewicz M, Wieczorowski M, 2013, Age-dependent biomechanical properties of the skin. Postepy Dermatol Alergol, 30(5): 302–306. https://doi.org/10.5114/ pdia.2013.38359
68. Li C, Guan G, Reif R, et al., 2012, Determining elastic properties of skin by measuring surface waves from an impulse mechanical stimulus using phase-sensitive optical coherence tomography. J R Soc Interface, 9(70): 831–841.
69. Peretiatko CDS, Hupalo EA, Da Rocha Campos JR, et al., 2018, Efficiency of zinc and calcium ion crosslinking in alginate-coated nitrogen fertilizer. Orbital Electron J Chem, 10: 218–225.
70. Swamy BJRS, Vijay R, Babu PR, et al., 2016, Influence of γ-ray-induced effects on dielectric dispersion of CuO-doped calcium fluoroborophosphate glass system. Ionics, 22: 1625–1634.
71. Zhang P, Pu Y, Wu Y, et al., 2016, Influence of replacement of B2O3 by SiO2 on the structure and magnetic properties of BaO-Fe2O3-SiO2-B2O3-CeO2 glass-ceramics. J Superconduct Novel Magn, 29: 1557–1560.
72. Fayyazbakhsh F, Solati-Hashjin M, Keshtkar A, et al., 2017, Novel layered double hydroxides-hydroxyapatite/gelatin bone tissue engineering scaffolds: Fabrication, characterization, and in vivo study. Mater Sci Eng C, 76: 701–714.
73. Fayyazbakhsh F, Solati-Hashjin M, Keshtkar A, et al., 2017, Release behavior and signaling effect of vitamin D3 in layered double hydroxides-hydroxyapatite/gelatin bone tissue engineering scaffold: An in vitro evaluation. Colloids Surfaces B Biointerfaces, 158: 697–708.
74. Köse D, Necefoğlu H, 2008, Synthesis and characterization of bis (nicotinamide) m-hydroxybenzoate complexes of Co (II), Ni (II), Cu (II) and Zn (II). J Therm Anal Calorim, 93: 509–514.
75. Visser H, Dubé CE, Armstrong WH, et al., 2002, FTIR spectra and normal-mode analysis of a tetranuclear manganese adamantane-like complex in two electrochemically prepared oxidation states: Relevance to the oxygen-evolving complex of photosystem II. J Am Chem Soc, 124(37): 11008–11017.
76. Zahir MH, Rahman MM, Irshad K, et al., 2019, Shape-stabilized phase change materials for solar energy storage: MgO and Mg(OH)2 mixed with polyethylene glycol. Nanomaterials, 9(12): 1773.
77. Łabowska MB, Skrodzka M, Sicińska H, et al., 2023, Influence of cross-linking conditions on drying kinetics of alginate hydrogel. Gels, 9(1): 63.
78. Paul D, 2011, Elaborations on the Higuchi model for drug delivery. Int J Pharmaceut, 418(1): 13–17.
79. Siepmann J, Peppas NA, 2011, Higuchi equation: Derivation, applications, use and misuse. Int J Pharm, 418(1): 6–12. https://doi.org/10.1016/j.ijpharm.2011.03.051
80. Klimek K, Ginalska G, 2020, Proteins and peptides as important modifiers of the polymer scaffolds for tissue engineering applications: A review. Polymers (Basel), 12(4): 1–38. https://doi.org/10.3390/polym12040844
81. Frew Q, Rennekampff H-O, Dziewulski P, et al., 2019, Betulin wound gel accelerated healing of superficial partial thickness burns: Results of a randomized, intra‐individually controlled, phase III trial with 12‐months follow‐up. Burns, 45(4): 876–890. https://doi.org/10.1016/j.burns.2018.10.019
82. Singer AJ, Boyce ST, 2017, Burn wound healing and tissue engineering. J Burn Care Res, 38(3): e605–e613. https://doi.org/10.1097/bcr.0000000000000538
83. Benson A, Dickson WA, Boyce DE, 2006, ABC of wound healing: Burns. BMJ, 333(Suppl S3): 283–339.
84. Jones K, 2015, Fibrotic Response to Biomaterials and All Associated Sequence of Fibrosis. Host Response to Biomaterials. Elsevier, Amsterdam, Netherlands, 189–237.
85. Kolimi P, Narala S, Nyavanandi D, et al., 2022, Innovative treatment strategies to accelerate wound healing: Trajectory and recent advancements. Cells; 11(15): 1–46. https://doi.org/10.3390/cells11152439