3D bioprinting for vascular grafts and microvasculature
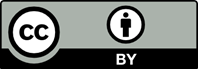
Cardiovascular disease is the world’s leading cause of death, and there is a substantial clinical need for transplantable blood vessels. Through tissue vascular engineering technology, large blood vessel grafts with significant clinical effects have been synthesized. However, synthesizing vascular valves, small vessels up to 6 mm in diameter, and capillary networks up to 500 μm in diameter remains challenging due to the lack of precise manufacturing techniques. In particular, constructing a microvascular network in thick tissue is the technical bottleneck of organ transplantation. Three-dimensional (3D) bioprinting is a computer-assisted layer-by-layer deposition method that can deposit cells and biomaterials at a predetermined location, according to an accurate digital 3D model, to build a delicate and complex bionic structure. This review discusses the progress and limitations of 3D bioprinting in preparing large vessels and valves, small-diameter vessels, and microvascular networks. This paper focuses on improved printing technology and innovative bio-ink materials. The future application of 3D bioprinting is prospected in generating artificial blood vessel grafts and vascularized organs with full biological function.
- Fazal F, Raghav S, Callanan A, et al., 2021, Recent advancements in the bioprinting of vascular grafts. Biofabrication, 13(032003): 032003–032021. https://doi.org/10.1088/1758-5090/ac0963
- Nienaber C, Clough R, Sakalihasan N, 2016, Aortic dissection. Nat Rev Dis Primers, 2: 16053–16070. https://doi.org/10.1038/nrdp.2016.53
- Devillard CD, Marquette CA, 2021, Vascular tissue engineering: Challenges and requirements for an ideal large scale blood vessel. Front Bioeng Biotechnol, 9(October): 1–21. https://doi.org/10.3389/fbioe.2021.721843
- Stout KK, Otto CM, 2007, Indications for aortic valve replacement in aortic stenosis. J Intens Care Med, 22(1): 14–25. https://doi.org/10.1177/0885066606295298
- Maselli D, Minzioni G, 2006, A technique to reposition sinotubular junction in aortic valve reimplantation procedures with the de Paulis Valsalva graft. Eur J Cardiothorac Surg, 29(1): 107–109. https://doi.org/10.1016/j.ejcts.2005.10.028
- Kehl D, Weber B, Hoerstrup SP, 2016, Bioengineered living cardiac and venous valve replacements: Current status and future prospects. Cardiovasc Pathol, 25(4): 300–305. https://doi.org/10.1016/j.carpath.2016.03.001
- Mallis P, Kostakis A, Stavropoulos-giokas C, 2020, Future perspectives in small-diameter vascular graft engineering. Bioengineering, 7(4): 1–40. https://doi.org/10.3390/bioengineering7040160
- Kannan RY, Salacinski HJ, Butler PE, et al., 2005, Current status of prosthetic bypass grafts: A review. J Biomed Mater Res - Part B Appl Biomater, 74(1): 570–581. https://doi.org/10.1002/jbm.b.30247
- Gemelli M, Gallo M, Addonizio M, 2023, Venous external support in coronary artery bypass surgery: A systematic review and meta-analysis. Curr Probl Cardiol, 101687. https://doi.org/10.1016/j.cpcardiol.2023.101687
- Pashneh-Tala S, MacNeil S, Claeyssens F, 2016, The tissue-engineered vascular graft - Past, present, and future. Tissue Eng - Part B: Rev, 22(1): 68–100. https://doi.org/10.1089/ten.teb.2015.0100
- Weekes A, Bartnikowski N, Pinto N, et al., Biofabrication of small diameter tissue-engineered vascular grafts. Acta Biomater, 138(15): 92–111. https://doi.org/10.1016/j.actbio.2021.11.012
- Barrs RW, Jia J, Silver SE, et al., 2020, Biomaterials for bioprinting microvasculature. Chem Rev, 120(19): 10887– 10949. https://doi.org/10.1021/acs.chemrev.0c00027
- Li X, Liu L, Zhang X, et al., 2018, Research and development of 3D printed vasculature constructs. Biofabrication, 10(032002): 032002–032014. https://doi.org/10.1088/1758-5090/aabd56
- Wang P, Sun Y, Shi X, et al., 2021, 3D printing of tissue engineering scaffolds: A focus on vascular regeneration. Biodes Manuf, 4(2):. 344. https://doi.org/10.1007/S42242-020-00109-0
- Li H, Tan C, Li L, 2018, Review of 3D printable hydrogels and constructs. Mater Des, 159(5): 20–38. https://doi.org/10.1016/j.matdes.2018.08.023
- Zieliński PS, Gudeti PKR, Rikmanspoel T, et al., 2023, 3D printing of bio-instructive materials: Toward directing the cell. Bioact Mater, 19(1): 292–327. https://doi.org/10.1016/j.bioactmat.2022.04.008
- Decante G, Costa JB, Silva-Correia J, et al., 2021, Engineering bioinks for 3D bioprinting. Biofabrication, 13(032001): 032001–032031. https://doi.org/10.1088/1758-5090/abec2c
- Chen YH, Chang HH, Kuo CC, et al., 2022, Impact of prosthesis-patient mismatch after transcatheter aortic valve replacement in Asian patients. Annal Thorac Surg, 114(5): 1612–1619. https://doi.org/10.1016/j.athoracsur.2021.09.016
- Kucukgul C, Ozler S, Inci I, et al., 2015, 3D bioprinting of biomimetic aortic vascular constructs with self-supporting cells. Biotechnol Bioeng, 112(4): 811–821. https://doi.org/10.1002/bit.25493
- Kucukgul C, Ozler B, Karakas HE, et al., 2013, 3D hybrid bioprinting of macrovascular structures. Procedia Eng, 59: 183–192. https://doi.org/10.1016/j.proeng.2013.05.109
- Oropeza BP, Adams JR, Furth ME, et al., 2022, Bioprinting of decellularized porcine cardiac tissue for large-scale aortic models. Front Bioeng Biotechnol, 10(March): 1–9. https://doi.org/10.3389/fbioe.2022.855186
- Potere F, Belgio B, Croci G, 2022, 3D bioprinting of multi-layered segments of a vessel-like structurewith ECM and novel derived bioink. Front Bioeng Biotechnol, 10(August): 1–13. https://doi.org/10.3389/fbioe.2022.918690
- Hockaday LA, Kang K, Colangelo N, 2012, Rapid 3D printing of anatomically accurate and mechanically heterogeneous aortic valve hydrogel scaffolds. Biofabrication, 4(3): 035005–035017. https://doi.org/10.1088/1758-5082/4/3/035005
- Vukicevic M, Mehta SM, Grande-Allen KJ, et al., 2022, Development of 3D printed mitral valve constructs for transcatheter device modeling of tissue and device deformation. Ann Biomed Eng, 50(4): 426–439. https://doi.org/10.1007/s10439-022-02927-y
- Duan B, Hockaday LA, Kang KH, et al., 2013, 3D bioprinting of heterogeneous aortic valve conduits with alginate/gelatin hydrogels. 101(5): 1255–1264. https://doi.org/10.1002/jbm.a.34420.3D
- Rioux Y, Fradette J, Maciel Y, et al., 2022, Biofabrication of sodium alginate hydrogel scaffolds for heart valve tissue engineering. Int J Mol Sci, 23(8567): 8567–8581. https://doi.org/10.3390/ijms23158567
- Zhou X, Nowicki M, Sun H, et al., 2020, 3D bioprinting-tunable small-diameter blood vessels with biomimetic biphasic cell layers. Appl Mater Interfaces, 12(41): 45904– 45915. https://doi.org/10.1021/acsami.0c14871
- Kamaraj M, Giri PS, Mahapatra S, et al., 2022, Bioengineering strategies for 3D bioprinting of tubular construct using tissue-specific decellularized extracellular matrix. Int J Biol Macromol, 223(PA): 1405–1419. https://doi.org/10.1016/j.ijbiomac.2022.11.064
- Gold KA, Saha B, Krishna N, 2021, 3D bioprinted multi-cellular vascular models. Adv Healthc Mater, 10(21): e2101141. https://doi.org/10.1002/ADHM.202101141
- Li L, Qin S, Peng J, 2020, Engineering gelatin-based alginate/ carbon nanotubes blend bioink for direct 3D printing of vessel constructs. Int J Biol Macromol, 145(15): 262–271. https://doi.org/10.1016/j.ijbiomac.2019.12.174
- Liu Y, Zhang Y, An Z, et al., 2021, Slide-ring structure-based double-network hydrogel with enhanced stretchability and toughness for 3d-bio-printing and its potential application as artificial small-diameter blood vessels. Appl Bio Mater, 4(12): 8597–8606. https://doi.org/10.1021/acsabm.1c01052
- Freeman S, Ramos R, Alexis Chando P, 2019, A bioink blend for rotary 3D bioprinting tissue engineered small-diameter vascular constructs. Acta Biomater, 95: 152–164. https://doi.org/10.1016/j.actbio.2019.06.052
- Zhou Y, Gui Q, Yu W, 2019, Interfacial diffusion printing: An efficient manufacturing technique for artificial tubular grafts. ACS Biomater Sci Eng, 5(11): 6311–6318. https://doi.org/10.1021/acsbiomaterials.9b01293
- Jin Q, Jin G, Ju J, 2022, Bioprinting small-diameter vascular vessel with endothelium and smooth muscle by the approach of two-step crosslinking process. Biotechnol Bioeng, 119(6): 1673–1684. https://doi.org/10.1002/bit.28075
- Zhang Z, Wu C, Dai C, 202, A multi-axis robot-based bioprinting system supporting natural cell function preservation and cardiac tissue fabrication. Bioact Mater, 18(1): 138. https://doi.org/10.1016/J.BIOACTMAT.2022.02.009
- Fazal F, Diaz Sanchez FJ, Waqas M, et al., 2021, A modified 3D printer as a hybrid bioprinting-electrospinning system for use in vascular tissue engineering applications. Med Eng Phys, 94: 52–60.https://doi.org/10.1016/j.medengphy.2021.06.005
- Jin Q, Fu Y, Zhang G, 2022, Nanofiber electrospinning combined with rotary bioprinting for fabricating small-diameter vessels with endothelium and smooth muscle. Compos B Eng, 234(1): 109691–109700. https://doi.org/10.1016/j.compositesb.2022.109691
- Größbacher G, Bartolf-Kopp M, Gergely C, 2023, Volumetric printing across melt electrowritten scaffolds fabricates multi‐material living constructs with tunable architecture and mechanics. Adv Mater, 2300756–2300773. https://doi.org/10.1002/adma.202300756
- Cao P, Tao L, Gong J, 2021, 4D printing of a sodium alginate hydrogel with step-wise shape deformation based on variation of crosslinking density. ACS Appl Polym Mater, 3(12): 6167–6175. https://doi.org/10.1021/acsapm.1c01034
- Kitana W, Apsite I, Hazur J, et al., 2023, 4D biofabrication of t-shaped vascular bifurcation. Adv Mater Technol, 8(1): 2200429–2200439. https://doi.org/10.1002/admt.202200429
- Kirillova A, Maxson R, Stoychev G, et al., 2017, 4D biofabrication using shape-morphing hydrogels. Adv Mater, 29(46): 1703443–1703450. https://doi.org/10.1002/adma.201703443
- Wu Z, Cai H, Ao Z, et al., 2021, Microfluidic printing of tunable hollow microfibers for vascular tissue engineering. Adv Mater Technol, 6(8): 2000683–2000691. https://doi.org/10.1002/admt.202000683
- Muthusamy S, Kannan S, Lee M, 2021, 3D bioprinting and microscale organization of vascularized tissue constructs using collagen-based bioink. Biotechnol Bioeng, 118(8): 3150–3163. https://doi.org/10.1002/bit.27838
- Cidonio G, Glinka M, Kim Y, 2020, Nanoclay-based 3D printed scaffolds promote vascular ingrowth ex vivo and generate bone mineral tissue in vitro and in vivo. Biofabrication, 12(3): 035010–035025. https://doi.org/10.1088/1758-5090/ab8753
- Liu C, Campbell S, Li J, 2022, High throughput omnidirectional printing of tubular microstructures from elastomeric polymers. Adv Healthc Mater, 11(23): 2201346– 2201359. https://doi.org/10.1002/adhm.202201346
- Barrs RW, Jia J, Ward M, 2021, Engineering a chemically defined hydrogel bioink for direct bioprinting of microvasculature. Biomacromolecules, 22(2): 275–288. https://doi.org/10.1021/acs.biomac.0c00947
- Yu H, Gong W, Mei J, 2022, The efficacy of a paeoniflorin-sodium alginate-gelatin skin scaffold for the treatment of diabetic wound: An in vivo study in a rat model. Biomed Pharmacotherap, 151: 113165–113171. https://doi.org/10.1016/j.biopha.2022.113165
- Cheng F, Cao X, Li H, 2019, Generation of cost-effective paper-based tissue models through matrix-assisted sacrificial 3D printing. Nano Lett, 19(6): 3603–3611. https://doi.org/10.1021/acs.nanolett.9b00583
- Zheng J, Liu Y, Hou C, 2022, Ovary-derived decellularized extracellular matrix-based bioink for fabricating 3D primary ovarian cells-laden structures for mouse ovarian failure correction. Int J Bioprint, 8(3): 269–282. https://doi.org/10.18063/IJB.V8I3.597
- Oliveira H, Medina C, Labrunie G, 2022, Cell-assembled extracellular matrix (CAM): A human biopaper for the biofabrication of pre-vascularized tissues able to connect to the host circulation in vivo. Biofabrication, 14(1): 015005–015019. https://doi.org/10.1088/1758-5090/ac2f81
- Li S, Wang W, Li W, 2021, Fabrication of thermoresponsive hydrogel scaffolds with engineered microscale vasculatures. Adv Funct Mater, 31(27): 2102685–2102699. https://doi.org/10.1002/adfm.202102685
- Thomas A, Orellano I, Lam T, 2020, Vascular bioprinting with enzymatically degradable bioinks via multi-material projection-based stereolithography. Acta Biomater, 117: 121–132. https://doi.org/10.1016/j.actbio.2020.09.033
- Zeng X, Meng Z, He J, 2022, Embedded bioprinting for designer 3D tissue constructs with complex structural organization. Acta Biomater, 140: 1–22. https://doi.org/10.1016/j.actbio.2021.11.048
- Zhang S, Qi C, Zhang W, 2022, In‐situ endothelialization of freeform 3D network of interconnected tubular channels via interfacial coacervation by aqueous‐in‐aqueous embedded bioprinting. Adv Mater, 35(7): 2209263–2209269. https://doi.org/10.1002/adma.202209263
- Hinton TJ, Jallerat Q, Palchesko R, 2015, Three-dimensional printing of complex biological structures by freeform reversible embedding of suspended hydrogels. Sci Adv, 1(9): e1500758–e1500767. https://doi.org/10.1126/sciadv.1500758
- Lee A, Hudson A, Shiwarski D, 2019, 3D bioprinting of collagen to rebuild components of the human heart. 365(6452): 482–487. https://doi.org/10.1126/science.aav9051
- Zhu W, Ma X, Gou M, et al., 2016, 3D printing of functional biomaterials for tissue engineering. Curr Opin Biotechnol, 40: 103–112.https://doi.org/10.1016/j.copbio.2016.03.014
- Dobos A, Gantner F, Markovic M, 2020, On-chip high-definition bioprinting of microvascular structures. Biofabrication, 13(1). https://doi.org/10.1088/1758-5090/abb063
- Xue D, Wang Y, Zhang J, et al., 2018, Projection-based 3D printing of cell patterning scaffolds with multi-scale channels. ACS Appl Mater Interfaces, 10(23): 19428–19435. https://doi.org/10.1021/acsami.8b03867
- Anada T, Pan C, Stahl A, 2019, Vascularized bone-mimetic hydrogel constructs by 3D bioprinting to promote osteogenesis and angiogenesis. Int J Mol Sci, 20(5): 1096–1104. https://doi.org/10.3390/ijms20051096
- Liu Y, Zhang Y, Mei T, 2022, hESCs-derived early vascular cell spheroids for cardiac tissue vascular engineering and myocardial infarction treatment. Adv Sci, 9(9): 2104299–2104311. https://doi.org/10.1002/advs.202104299
- Benmeridja L, De Moor L, De Maere E, 2022, High-throughput fabrication of vascularized adipose microtissues for 3D bioprinting. J Tissue Eng Regen Med, 14(6): 840–854. https://doi.org/10.1002/term.3051
- de Moor L, Smet J, Plovyt M, 2021, Engineering microvasculature by 3D bioprinting of prevascularized spheroids in photo-crosslinkable gelatin. Biofabrication, 13(4): 045021–045041. https://doi.org/10.1088/1758-5090/ac24de
- Dogan L, Scheuring R, Wagner N, 2021, Human iPSC-derived mesodermal progenitor cells preserve their vasculogenesis potential after extrusion and form hierarchically organized blood vessels. Biofabrication, 13(4): 045028–045044. https://doi.org/10.1088/1758-5090/ac26ac
- O’Connor C, Brady E, Zheng Y, et al., 2022, Engineering the multi-scale complexity of vascular networks. Nat Rev Mater, 7(9): 702–716. https://doi.org/10.1038/s41578-022-00447-8
- Mirabella T, Macarthur J, Cheng D, 2017, 3D-printed vascular networks direct therapeutic angiogenesis in ischaemia. Nat Biomed Eng, 1(6): 0083–0090. https://doi.org/10.1038/s41551-017-0083
- Kérourédan O, Hakobyan D, Remy M, 2019, In situ prevascularization designed by laser-assisted bioprinting: Effect on bone regeneration. Biofabrication, 11(4): 045002–045019. https://doi.org/10.1088/1758-5090/ab2620
- Chansoria P, Asif S, Gupta N, et al., 2022, Multi-scale anisotropic tissue biofabrication via bulk acoustic patterning of cells and functional additives in hybrid bioinks. Adv Healthc Mater, 11(10): 2102351–2102367. https://doi.org/10.1002/adhm.202102351
- Maiullari F, Costantini M, Milan M, 2018, A multi-cellular 3D bioprinting approach for vascularized heart tissue engineering based on HUVECs and iPSC-derived cardiomyocytes. Sci Rep, 8(1): 13532–13547. https://doi.org/10.1038/s41598-018-31848-x
- Son J, Hong SJ, Lim JW, et al., 2021, Engineering tissue-specific, multi-scale microvasculature with a capillary network for prevascularized tissue. Small Methods, 5(10): 2100632–2100643. https://doi.org/10.1002/smtd.202100632
- Nie J, Gao Q, Xie C, 2020, Construction of multi-scale vascular chips and modelling of the interaction between tumours and blood vessels. Mater Horiz, 7(1): 82–92. https://doi.org/10.1039/c9mh01283d
- Szklanny AA, Machour M, Redenski I, 2021, 3D bioprinting of engineered tissue flaps with hierarchical vessel networks (VesselNet) for direct host-to-implant perfusion. Adv Mater, 33(42): 2102661–2102679. https://doi.org/10.1002/adma.202102661
- McFetridge PS, Abe K, Horrocks M, et al., 2007, Vascular tissue engineering: Bioreactor design considerations for extended culture of primary human vascular smooth muscle cells. ASAIO J, 53(5): 623–630. https://doi.org/10.1097/MAT.0b013e31812f3b7e
- Wang J, Kural M, Wu J, 2021, An ex vivo physiologic and hyperplastic vessel culture model to study intra-arterial stent therapies. Biomaterials, 275: 120911–120924. https://doi.org/10.1016/j.biomaterials.2021.120911
- Syedain ZH, Meier LA, Bjork JW, et al., 2011, Implantable arterial grafts from human fibroblasts and fibrin using a multi-graft pulsed flow-stretch bioreactor with non-invasive strength monitoring. Biomaterials, 32(3): 714–722. https://doi.org/10.1016/j.biomaterials.2010.09.019
- Kawecki F, L’Heureux N, 2023, Current biofabrication methods for vascular tissue engineering and an introduction to biological textiles. Biofabrication, 15(2): 022004–022025. https://doi.org/10.1088/1758-5090/acbf7a