Ineffective voluntary motor improvement through non-invasive BCI-FES with static magnetic field in complete spinal cord injury: A pilot study
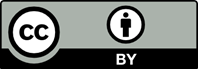
In response to the challenge of spinal cord injury (SCI) rehabilitation, this study aimed to investigate the effect of applying a non-invasive interface of surface neuroelectrical signals and functional electrical stimulation (sNES-sFES) with a static magnetic field on the motor outcome of the quadriceps femoris muscle in an individual with a complete SCI. The participant, who had a complete SCI in the chronic stage, was evaluated before (pre) and after nine (post) interventions using surface electromyography (sEMG). In addition, spasticity (modified Ashworth scale [MAS]) was observed in all sessions. Moreover, the user learning curve process (classifier percentage and correct success of the sFES hits) was evaluated. In general, we observed: (i) No voluntary muscle contraction (pre- and post-root mean square of sEMG = 0%) improvement; (ii) spasticity decrease (average one point in MAS); (iii) gradual improvement in the user learning effect on the interface, reaching 84% in classifier accuracy and a maximum percentage of correct sFES activation of 53%. In conclusion, no improvement in voluntary muscular contraction was observed within 9 weeks of the intervention (1 session/day; 1 h/week). However, our study demonstrates the safety and feasibility of our methodology for future research involving continuous physical rehabilitation training and the implementation of assistive technology.
- Brown AR, Martinez M. From cortex to cord: Motor circuit plasticity after spinal cord injury. Neural Regen Res. 2019;14(12):2054-2062. doi: 10.4103/1673-5374.262572
- Yang T, Dai Y, Chen G, Cui, S. Dissecting the dual role of the glial scar and scar-forming astrocytes in spinal cord injury. Front Cell Neurosci. 2020;14:78. doi: 10.3389/fncel.2020.00078
- Aggarwal S, Chugh, N. Signal processing techniques for motor imagery brain computer interface: A review. Array. 2019;1-2:100003. doi: 10.1016/j.array.2019.100003
- Barroso FO, Pascual-Valdunciel A, Torricelli D, et al. Noninvasive modalities used in spinal cord injury rehabilitation. In: Spinal Cord Injury Therapy. London: IntechOpen; 2019. doi: 10.5772/intechopen.83654
- Chédotal A. Roles of axon guidance molecules in neuronal wiring in the developing spinal cord. Nat Rev Neurosci. 2019;20(7):380-396. doi: 10.1038/s41583-019-0168-7
- Courtine G, Sofroniew MV. Spinal cord repair: Advances in biology and technology. Nat Med. 2019;25(6):898-908. doi: 10.1038/s41591-019-0475-6
- Friedrich P, Waschke J. Sobotta; Editora Guanabara Koogan LTDA. United States: Bloomberg; 2012.
- Krueger-Beck E, Scheeren EM, Neto GNN, Button VL, Nohama P. Effects Of Functional Electrical Stimulation In Artificial Neuromuscular Control. Rev Neurociên. 2011;19:530-541. doi: 10.4181/RNC.2010.06ip.11
- Mukhopadhyay R, Lenka PK, Biswas A, Mahadevappa M. Evaluation of functional mobility outcomes following electrical stimulation in children with spastic cerebral palsy. J Child Neurol. 2017;32(7):650-656. doi: 10.1177/0883073817700604
- Selfslagh A, Shokur S, Campos DS, et al. Non-invasive, Brain-controlled functional electrical stimulation for locomotion rehabilitation in individuals with Paraplegia. Sci Rep. 2019;9(1):6782. doi: 10.1038/s41598-019-43041-9
- Abdalsalam ME, Yusoff MZ, Kamel N, et al. Mental Task Motor Imagery Classifications for Noninvasive Brain Computer Interface. In: Proceedings of the 2014 5th International Conference on Intelligent and Advanced Systems: Technological Convergence for Sustainable Future, ICIAS 2014 (ICIAS). United States: IEEE. 2014. p. 1-5. doi: 10.1109/ICIAS.2014.6869531
- Hedayatzadeh M, Tehranipour M, Kobravi HR. Motor neuron recovery in rats after incomplete spinal cord injury using intra-spinal electrical stimulation and stem cell transfusion: A prelude to human applications. Med Sci. 2020;24:706-716.
- Krueger E, Magri LM, Botelho AS, et al. Effects of low-intensity electrical stimulation and adipose derived stem cells transplantation on the time-domain analysis-based electromyographic signals in dogs with SCI. Neurosci Lett. 2019;696:38-45. doi: 10.1016/j.neulet.2018.12.004
- Farrell K, Detloff MR, Houle JD. Plastic changes after spinal cord injury. In: Oxford Research Encyclopedia of Neuroscience. Oxford: Oxford University Press; 2019. doi: 10.1093/acrefore/9780190264086.013.241
- Leemhuis E, De Gennaro L, Pazzaglia AM. Disconnected body representation: Neuroplasticity following spinal cord injury. J Clin Med. 2019;8(12):2144. doi: 10.3390/jcm8122144
- Spieker EL, Wiesener C, Niedeggen A, Wenger N Schauer T. Motor and sensor recovery in a paraplegic by transcutaneous spinal cord stimulation in water. In: Proceedings on Automation in Medical Engineering. Vol. 1; 2020. p. 22. doi: 10.18416/AUTOMED.2020
- Chaudhary U, Birbaumer N, Ramos-Murguialday, A. Brain-computer interfaces for communication and rehabilitation. Nat Rev Neurol. 2016;12(9):513-525. doi: 10.1038/nrneurol.2016.113
- Osuagwu BC, Wallace L, Fraser M, Vuckovic A. Rehabilitation of hand in subacute tetraplegic patients based on brain computer interface and functional electrical stimulation: A randomised pilot study. J Neural Eng. 2016;13(6):065002. doi: 10.1088/1741-2560/13/6/065002
- Macias MY, Battocletti JH, Sutton CH, Pintar FA, Maiman DJ. Directed and enhanced neurite growth with pulsed magnetic field stimulation. Bioelectromagnetics. 2000;21:272-286.
- Fujioka Y, Tanaka N, Nakanishi K, et al. Magnetic field-based delivery of human CD1331 cells promotes functional recovery after rat spinal cord injury. Spine. 2012;7(13):E768-E777. doi: 10.1097/BRS.0b013e318246d59c
- Silvestro S, Bramanti P, Trubiani O, Mazzon E. Stem cells therapy for spinal cord injury: An overview of clinical trials. Int J Mol Sci. 2020;21:659. doi: 10.3390/ijms21020659
- Fukuda TY, Echeimberg JO, Pompeu JE, et al. Root mean square value of the electromyographic signal in the isometric torque of the quadriceps, hamstrings and brachial biceps muscles in female subjects. J Appl Res. 2010;10:32-39.
- Júnior PB, Campos DP, Lazzaretti AE, et al. Influence of EEG channel reduction on lower limb motor imagery during electrical stimulation in healthy and paraplegic subjects. Res Biomed Eng. 2022;38:1-11. doi: 10.1007/s42600-021-00189-6
- Broniera Junior PB, Campos DP, Lazzaretti AE, et al. EEG-FES-Force-MMG closed-loop control systems of a volunteer with paraplegia considering motor imagery with fatigue recognition and automatic shut-off. Biomed Signal Process Control. 2021;68,102662. doi: 10.1016/j.bspc.2021.102662
- Silva CR, De Araújo RS, Albuquerque G, Moioli RC, Brasil FL. Interfacing brains to robotic devices-A VRPN communication application. In: Proceedings of the XXVI Brazilian Congress on Biomedical Engineering. Germany: Springer; 2019. p. 597-603.
- Nogueira-Neto G, Scheeren E, Krueger E, et al. The influence of window length analysis on the time and frequency domain of mechanomyographic and electromyographic signals of submaximal fatiguing contractions. Open J Biophys. 2013;3(3):178-190. doi: 0.4236/ojbiphy.2013.33021
- International Electrotechnical Commission. IEC 60601-2- 10: 2012-Medical Electrical Equipment-Part 2-10: Particular Requirements for the Basic Safety and Essential Performance of Nerve and Muscle Stimulators. United Kingdom: International Electrotechnical Commission; 2012.
- Krueger E, Scheeren EM, Nogueira-Neto GN, Nohama P. Mechanomyography energy decreases during muscular fatigue in paraplegics. In: Proceedings of the 2014 36th Annual International Conference of the IEEE Engineering in Medicine and Biology Society. United States: IEEE. 2014:5824-5827. doi: 10.1109/EMBC.2014.6944952
- Rabischong E. Surface action potentials related to torque output in paraplegics’ electrically stimulated quadriceps muscle. Med Eng Phys. 1996;18:538-547. doi: 10.1016/1350-4533(96)00001-x
- Schiefer MA, Triolo RJ, Tyler DJ. A model of selective activation of the femoral nerve with a flat interface nerve electrode for a lower extremity neuroprosthesis. IEEE Trans Neural Syst Rehabil Eng. 2008;16(2):195-204. doi: 10.1109/TNSRE.2008.918425
- Beros J, King E, Clarke D, Jaeschke-Angi L, Tang AD. Static magnetic stimulation induces structural plasticity at the axon initial segment of inhibitory cortical neurons. Sci Rep. 2024;14(1):1479. doi: 10.1038/s41598-024-51845-7
- Donati AR, Shokur S, Morya E, et al. Long-term training with a brain-machine interface-based gait protocol induces partial neurological recovery in paraplegic patients. Sci Rep. 2016;6:30383. doi: 10.1038/srep30383
- Bekhet AH, Bochkezanian V, Saab IM, Gorgey AS. The effects of electrical stimulation parameters in managing spasticity after spinal cord injury: A systematic review. Am J Phys Med Rehabil. 2019;98(6):484-499. doi: 10.1097/PHM.0000000000001064
- Bhattacharyya S, Sahu S, Kaur S, Jain S. Effect of low intensity magnetic field stimulation on calcium-mediated cytotoxicity after mild spinal cord contusion injury in Rats. Ann Neurosci. 2020;27:49-56. doi: 10.1177/0972753120950072
- Kirimoto H, Tamaki H, Matsumoto T, et al. Effect of transcranial static magnetic field stimulation over the sensorimotor cortex on somatosensory evoked potentials in humans. Brain Stimul. 2014;7:836-840. doi: 10.1016/j.brs.2014.09.016
- Carrasco-López C, Soto-León V, Céspedes V, et al. Static magnetic field stimulation over parietal cortex enhances somatosensory detection in humans. J Neurosci. 2017;37:3840-3847. doi: 10.1523/JNEUROSCI.2123-16.2017
- Oliviero A, Carrasco-López M, Campolo M, et al. Safety study of transcranial static magnetic field stimulation (tSMS) of the human cortex. Brain Stimul. 2015;8:481-485. doi: 10.1016/j.brs.2014.12.002
- Nakagawa K, Nakazawa K. Static magnetic field stimulation applied over the cervical spinal cord can decrease corticospinal excitability in finger muscle. Clin Neurophysiol Pract. 2018;3:49-53. doi: 10.1016/j.cnp.2018.02.001
- King CE, Wang PT, McCrimmon CM, Chou CC, Do AH, Nenadic Z. The feasibility of a rain-computer interface functional electrical stimulation system for the restoration of overground walking after paraplegia. J Neuroeng Rehabil. 2015;12:1-11. doi: 10.1186/s12984-015-0068-7
- Schalk G, Wolpaw JR, McFarland DJ, Pfurtscheller G. EEG-based communication: Presence of an error potential. Clin Neurophysiol. 2000;111(12):2138-2144. doi: 10.1016/s1388-2457(00)00457-0
- Liu Q, Zheng W, Chen K, Ma L, Ai Q. Online detection of class-imbalanced error-related potentials evoked by motor imagery. J Neural Eng. 2021;18(4):046032. doi: 10.1088/1741-2552/abf522