Role of stem cells in aortic aneurysm
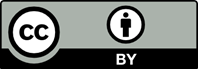
In recent years, aortic aneurysms (AAs) have attracted increasing attention due to their asymptomatic onset and high mortality. In clinic, surgery and anti-hypertensive or lipid-lowering medicine is usually applied in the treatments of AA. However, AA is prone to relapse and sudden rupture may happen. Therefore, more effective prevention and treatment methods are urgently needed. Stem cells are believed to play a crucial role in vascular formation and regeneration of damaged tissues during vascular disease progression. With the development of single-cell RNA sequencing analysis, many populations of stem cells have been discovered in AA tissues. Recent studies have demonstrated that they may participate in the occurrence or development of AAs. Besides, there is a prospect in clinical treatment for AAs when regenerative medicine with stem cells comes into the picture. This review mainly discusses the latest findings on the crucial roles of stem cells in AAs as well as their potential therapeutic strategies of stem cells, which provides some references for the prevention, diagnosis, and treatment of AAs. Further studies are needed to explore the novel application of stem cell therapies for AAs.
Liu B, Granville DJ, Golledge J, et al., 2020, Pathogenic mechanisms and the potential of drug therapies for aortic aneurysm. Am J Physiol Heart Circ Physiol, 318: H652– H670. https://doi.org/10.1152/ajpheart.00621.2019
Shen YH, LeMaire SA, Webb NR, et al., 2020, Aortic aneurysms and dissections series. Arterioscler Thromb Vasc Biol, 40: e37–e46. https://doi.org/10.1161/atvbaha.120.313991
Zhang L, Bhaloo SI, Chen T, et al., 2018, Role of resident stem cells in vessel formation and arteriosclerosis. Circ Res, 122: 1608–1624. https://doi.org/10.1161/circresaha.118.313058
Petsophonsakul P, Furmanik M, Forsythe R, et al., 2019, Role of vascular smooth muscle cell phenotypic switching and calcification in aortic aneurysm formation. Arterioscler Thromb Vasc Biol, 39: 1351–1368. https://doi.org/10.1161/atvbaha.119.312787
Clancy K, Wong J, Spicher A, 2019, Abdominal aortic aneurysm: A case report and literature review. Perm J, 23: 18.218. https://doi.org/10.7812/tpp/18.218
Quintana RA, Taylor WR, 2019, Introduction to the compendium on aortic aneurysms. Circ Res, 124: 470–471. https://doi.org/10.1161/circresaha.119.314765
Quintana RA, Taylor WR, 2019, Cellular mechanisms of aortic aneurysm formation. Circ Res, 124: 607–618. https://doi.org/10.1161/circresaha.118.313187
Hellawell HN, Mostafa AM, Kyriacou H, et al., 2021, Abdominal aortic aneurysms part one: Epidemiology, presentation and preoperative considerations. J Perioper Pract, 31: 274–280. https://doi.org/10.1177/1750458920954014
Boczar KE, Cheung K, Boodhwani M, et al., 2019, Sex differences in thoracic aortic aneurysm growth. Hypertension, 73: 190–196. https://doi.org/10.1161/hypertensionaha.118.11851
Wesche J, 2009, Epidemiology, investigation and treatment indication in aortic aneurysm. Tidsskr Nor Laegeforen, 129: 2124–2126. https://doi.org/10.4045/tidsskr.09.0160
Zhou Z, Cecchi AC, Prakash SK, et al., 2022, Risk factors for thoracic aortic dissection. Genes (Basel), 13: 1814. https://doi.org/10.3390/genes13101814
Sayed A, Munir M, Bahbah EI, 2021, Aortic dissection: A review of the pathophysiology, management and prospective advances. Curr Cardiol Rev, 17: e230421186875. https://doi.org/10.2174/1573403x16666201014142930
Gurung R, Choong AM, Woo CC, et al., 2020, Genetic and epigenetic mechanisms underlying vascular smooth muscle cell phenotypic modulation in abdominal aortic aneurysm. Int J Mol Sci, 21: 6334. https://doi.org/10.3390/ijms21176334
Chakraborty R, Saddouk FZ, Carrao AC, et al., 2019, Promoters to study vascular smooth muscle. Arterioscler Thromb Vasc Biol, 39: 603–612. https://doi.org/10.1161/atvbaha.119.312449
Ferruzzi J, Murtada SI, Li G, et al., 2016, Pharmacologically improved contractility protects against aortic dissection in mice with disrupted transforming growth factor-β signaling despite compromised extracellular matrix properties. Arterioscler Thromb Vasc Biol, 36: 919–927. https://doi.org/10.1161/atvbaha.116.307436
Clément M, Chappell J, Raffort J, et al., 2019, Vascular smooth muscle cell plasticity and autophagy in dissecting aortic aneurysms. Arterioscler Thromb Vasc Biol, 39: 1149– 1159. https://doi.org/10.1161/atvbaha.118.311727
Furmaniak-Kazmierczak E, Crawley SW, Carter RL, et al., 2007, Formation of extracellular matrix-digesting invadopodia by primary aortic smooth muscle cells. Circ Res, 100: 1328–1336. https://doi.org/10.1161/circresaha.106.147744
Duca L, Blaise S, Romier B, et al., 2016, Matrix ageing and vascular impacts: Focus on elastin fragmentation. Cardiovasc Res, 110: 298–308. https://doi.org/10.1093/cvr/cvw061
Halushka MK, Angelini A, Bartoloni G, et al., 2016, Consensus statement on surgical pathology of the aorta from the society for cardiovascular pathology and the association for European cardiovascular pathology: II. Noninflammatory degenerative diseases-nomenclature and diagnostic criteria. Cardiovasc Pathol, 25: 247–257. https://doi.org/10.1016/j.carpath.2016.03.002
Qin Y, Cao X, Guo J, et al., 2012, Deficiency of cathepsin S attenuates angiotensin II-induced abdominal aortic aneurysm formation in apolipoprotein E-deficient mice. Cardiovasc Res, 96: 401–410. https://doi.org/10.1093/cvr/cvs263
Jana S, Hu M, Shen M, et al., 2019, Extracellular matrix, regional heterogeneity of the aorta, and aortic aneurysm. Exp Mol Med, 51: 1–15. https://doi.org/10.1038/s12276-019-0286-3
Sun J, Sukhova GK, Zhang J, et al., 2012, Cathepsin K deficiency reduces elastase perfusion-induced abdominal aortic aneurysms in mice. Arterioscler Thromb Vasc Biol, 32: 15–23. https://doi.org/10.1161/atvbaha.111.235002
Sun J, Sukhova GK, Zhang J, et al., 2011, Cathepsin L activity is essential to elastase perfusion-induced abdominal aortic aneurysms in mice. Arterioscler Thromb Vasc Biol, 31: 2500-2508. https://doi.org/10.1161/atvbaha.111.230201
Infantes D, Nus M, Navas-Madroñal M, et al., 2021, Oxidative stress and inflammatory markers in abdominal aortic aneurysm. Antioxidants (Basel), 10: 602. https://doi.org/10.3390/antiox10040602
Emeto TI, Moxon JV, Au M, et al., 2016, Oxidative stress and abdominal aortic aneurysm: Potential treatment targets. Clin Sci (Lond), 130: 301–315. https://doi.org/10.1042/cs20150547
Li Z, Kong W, 2020, Cellular signaling in abdominal aortic aneurysm. Cell Signal, 70: 109575. https://doi.org/10.1016/j.cellsig.2020.109575
Wiernicki I, Parafiniuk M, Kolasa-Wołosiuk A, et al., 2019, Relationship between aortic wall oxidative stress/proteolytic enzyme expression and intraluminal thrombus thickness indicates a novel pathomechanism in the progression of human abdominal aortic aneurysm. FASEB J, 33: 885–895. https://doi.org/10.1096/fj.201800633R
Lu H, Daugherty A, 2017, Aortic aneurysms. Arterioscler Thromb Vasc Biol, 37: e59–e65. https://doi.org/10.1161/atvbaha.117.309578
Davis FM, Daugherty A, Lu HS, 2019, Updates of recent aortic aneurysm research. Arterioscler Thromb Vasc Biol, 39: e83–e90. https://doi.org/10.1161/atvbaha.119.312000
Dale MA, Ruhlman MK, Baxter BT, 2015, Inflammatory cell phenotypes in AAAs: Their role and potential as targets for therapy. Arterioscler Thromb Vasc Biol, 35: 1746–1755. https://doi.org/10.1161/atvbaha.115.305269
Meher AK, Spinosa M, Davis JP, et al., 2018, Novel role of IL (Interleukin)-1/10. neutrophil extracellular trap formation and abdominal aortic aneurysms. Arterioscler Thromb Vasc Biol, 38: 843–853. https://doi.org/10.1161/atvbaha.117.309897
Pedroza AJ, Tashima Y, Shad R, et al., 2020, Single-cell transcriptomic profiling of vascular smooth muscle cell phenotype modulation in Marfan syndrome aortic aneurysm. Arterioscler Thromb Vasc Biol, 40: 2195–2211. https://doi.org/10.1161/atvbaha.120.314670
Wu D, Ren P, Zheng Y, et al., 2017, NLRP3 (Nucleotide oligomerization domain-like receptor family, Pyrin domain containing 3)-caspase-1 inflammasome degrades contractile proteins: Implications for aortic biomechanical dysfunction and aneurysm and dissection formation. Arterioscler Thromb Vasc Biol, 37: 694–706. https://doi.org/10.1161/atvbaha.116.307648
Portelli SS, Hambly BD, Jeremy RW, et al., 2021, Oxidative stress in genetically triggered thoracic aortic aneurysm: Role in pathogenesis and therapeutic opportunities. Redox Rep, 26: 45–52. https://doi.org/10.1080/13510002.2021.1899473
Irace FG, Cammisotto V, Valenti V, et al., 2021, Role of oxidative stress and autophagy in thoracic aortic aneurysms. JACC Basic Transl Sci, 6: 719–730. https://doi.org/10.1016/j.jacbts.2021.08.002
Isselbacher EM, Lino Cardenas CL, Lindsay ME, 2016, Hereditary influence in thoracic aortic aneurysm and dissection. Circulation, 133: 2516–2528. https://doi.org/10.1161/circulationaha.116.009762
Milewicz DM, Trybus KM, Guo DC, et al., 2017, Hereditary influence in thoracic aortic aneurysm and dissection. Circulation, 133: 2516–2528. https://doi.org/10.1161/circulationaha.116.009762
Yang H, Zhou T, Stranz A, et al., 2021, Single-Cell RNA sequencing reveals heterogeneity of vascular cells in early stage murine abdominal aortic aneurysm-brief report. Arterioscler Thromb Vasc Biol, 41: 1158–1166. https://doi.org/10.1161/atvbaha.120.315607
Zhao G, Lu H, Chang Z, et al., 2021, Single-cell RNA sequencing reveals the cellular heterogeneity of aneurysmal infrarenal abdominal aorta. Cardiovasc Res, 117: 1402–1416. https://doi.org/10.1093/cvr/cvaa214
Qian W, Hadi T, Silvestro M, et al., 2022, Microskeletal stiffness promotes aortic aneurysm by sustaining pathological vascular smooth muscle cell mechanosensation via Piezo1. Nat Commun, 13: 512. https://doi.org/10.1038/s41467-021-27874-5
Hadi T, Boytard L, Silvestro M, et al., 2018, Macrophage-derived netrin-1 promotes abdominal aortic aneurysm formation by activating MMP3 in vascular smooth muscle cells. Nat Commun, 9: 5022. https://doi.org/10.1038/s41467-018-07495-1
Li B, Song X, Guo W, et al., 2021, Single-cell transcriptome profiles reveal fibrocytes as potential targets of cell therapies for abdominal aortic aneurysm. Front Cardiovasc Med, 8: 753711. https://doi.org/10.3389/fcvm.2021.753711
Weng Y, Lou J, Bao Y, et al., 2022, Single-cell RNA sequencing technology revealed the pivotal role of fibroblast heterogeneity in angiotensin ii-induced abdominal aortic aneurysms. DNA Cell Biol, 41: 498–520. https://doi.org/10.1089/dna.2021.0923
Liu X, Chen W, Zhu G, et al., 2022, Single-cell RNA sequencing identifies an Il1rn(+)/Trem1(+) macrophage subpopulation as a cellular target for mitigating the progression of thoracic aortic aneurysm and dissection. Cell Discov, 8: 11. https://doi.org/10.1038/s41421-021-00362-2
Luo W, Wang Y, Zhang L, et al., 2020, Critical role of cytosolic DNA and its sensing adaptor STING in aortic degeneration, dissection, and rupture. Circulation, 141: 42–66. https://doi.org/10.1161/circulationaha.119.041460
Li Y, Ren P, Dawson A, et al., 2020, Single-cell transcriptome analysis reveals dynamic cell populations and differential gene expression patterns in control and aneurysmal human aortic tissue. Circulation, 142: 1374–1388. https://doi.org/10.1161/circulationaha.120.046528
Davis FM, Tsoi LC, Melvin WJ, et al., 2021, Inhibition of macrophage histone demethylase JMJD3 protects against abdominal aortic aneurysms. J Exp Med, 218: e20201839. https://doi.org/10.1084/jem.20201839
Dawson A, Li Y, Li Y, et al., 2021, Single-cell analysis of aneurysmal aortic tissue in patients with Marfan syndrome reveals dysfunctional TGF-rosignaling. Genes (Basel), 13: 95. https://doi.org/10.3390/genes13010095
Yu B, Chen Q, Le Bras A, et al., 2018, Vascular stem/ progenitor cell migration and differentiation in atherosclerosis. Antioxid Redox Signal, 29: 219–235. https://doi.org/10.1089/ars.2017.7171
Skurikhin EG, Krupin VA, Pershina OV, et al., 2018, Endothelial progenitor cells and notch-1 signaling as markers of alveolar endothelium regeneration in pulmonary emphysema. Bull Exp Biol Med, 166: 201–206. https://doi.org/10.1007/s10517-018-4314-4
Chambers SE, Pathak V, Pedrini E, et al., 2021, Current concepts on endothelial stem cells definition, location, and markers. Stem Cells Transl Med, 10 Suppl 2(Suppl 2): S54– S61. https://doi.org/10.1002/sctm.21-0022
Sung SH, Wu TC, Chen JS, et al., 2013, Reduced number and impaired function of circulating endothelial progenitor cells in patients with abdominal aortic aneurysm. Int J Cardiol, 168: 1070–1077. https://doi.org/10.1016/j.ijcard.2012.11.002
Bingol B, Elcik D, Kutuk S, et al., 2022, Endothelial progenitor cells and NADPH oxidase enzyme activity in the development of an aortic aneurysm. Braz J Cardiovasc Surg, 37: 501–510. https://doi.org/10.21470/1678-9741-2020-0458
Eizawa T, Ikeda U, Murakami Y, et al., 2004, Increase in circulating endothelial progenitor cells after aortic aneurysm repair. Heart Vessels, 19: 107–110. https://doi.org/10.1007/s00380-003-0751-7
Wu W, Zhang J, Shao L, et al., 2022, Evaluation of circulating endothelial progenitor cells in abdominal aortic aneurysms after endovascular aneurysm repair. Int J Stem Cells, 15: 136–143. https://doi.org/10.15283/ijsc21027
Shi C, Shen C, Liu G, et al., 2021, NEAT1 promotes the repair of abdominal aortic aneurysms of endothelial progenitor cells via regulating miR-204-5p/Ang-1. Am J Transl Res, 13: 2111–2126.
Li X, Wei Z, Chen Y, 2022, CXCL12 regulates bone marrow-derived endothelial progenitor cells to promote aortic aneurysm recovery. Tissue Cell, 77: 101810. https://doi.org/10.1016/j.tice.2022.101810
Kavyasudha C, Joseph JP, Jayaraj R, et al., 2021, Conventional and emerging markers in stem cell isolation and characterization. Adv Exp Med Biol, 1341: 1–14. https://doi.org/10.1007/5584_2019_475
Aggarwal S, Pittenger MF, 2005, Human mesenchymal stem cells modulate allogeneic immune cell responses. Blood, 105: 1815–1822. https://doi.org/10.1182/blood-2004-04-1559
Zhou YZ, Cheng Z, Wu Y, et al., 2019, Mesenchymal stem cell-derived conditioned medium attenuate angiotensin II-induced aortic aneurysm growth by modulating macrophage polarization. J Cell Mol Med, 23: 8233–8245. https://doi.org/10.1111/jcmm.14694
Sharma AK, Lu G, Jester A, et al., 2012, Experimental abdominal aortic aneurysm formation is mediated by IL-17 and attenuated by mesenchymal stem cell treatment. Circulation, 126(11 Suppl 1): S38–S45.https://doi.org/10.1161/circulationaha.111.083451
Hawkins RB, Salmon M, Su G, et al., 2021, Mesenchymal stem cells alter microRNA expression and attenuate thoracic aortic aneurysm formation. J Surg Res, 268: 221–231. https://doi.org/10.1016/j.jss.2021.06.057
Zhang H, Wang Y, Bian X, et al., 2021, MicroRNA-194 acts as a suppressor during abdominal aortic aneurysm via inhibition of KDM3A-mediated BNIP3. Life Sci, 277: 119309. https://doi.org/10.1016/j.lfs.2021.119309
Spinosa M, Lu G, Su G, et al., 2018, Human mesenchymal stromal cell-derived extracellular vesicles attenuate aortic aneurysm formation and macrophage activation via microRNA-147. FASEB J, 32: fj201701138RR. https://doi.org/10.1096/fj.201701138RR
Swaminathan G, Stoilov I, Broekelmann T, et al., 2018, Phenotype-based selection of bone marrow mesenchymal stem cell-derived smooth muscle cells for elastic matrix regenerative repair in abdominal aortic aneurysms. J Tissue Eng Regen Med, 12: e60–e70. https://doi.org/10.1002/term.2349
Mildmay-White A, Khan W, 2017, Cell surface markers on adipose-derived stem cells: A systematic review. Curr Stem Cell Res Ther, 12: 484–492. https://doi.org/10.2174/1574888x11666160429122133
Xie J, Jones TJ, Feng D, et al., 2017, Human adipose-derived stem cells suppress elastase-induced murine abdominal aortic inflammation and aneurysm expansion through paracrine factors. Cell Transplant, 26: 173–189. https://doi.org/10.3727/096368916x692212
Hu J, Jiang Y, Wu X, et al., 2022, Exosomal miR-17-5p from adipose-derived mesenchymal stem cells inhibits abdominal aortic aneurysm by suppressing TXNIP-NLRP3 inflammasome. Stem Cell Res Ther, 13: 349. https://doi.org/10.1186/s13287-022-03037-1
Huang X, Zhang H, Liang X, et al., 2019, Adipose-derived mesenchymal stem cells isolated from patients with abdominal aortic aneurysm exhibit senescence phenomena. Oxid Med Cell Longev, 2019: 1305049. https://doi.org/10.1155/2019/1305049
Zou S, Ren P, Zhang L, et al., 2020, Activation of bone marrow-derived cells and resident aortic cells during aortic injury. J Surg Res, 245: 1–12. https://doi.org/10.1016/j.jss.2019.07.013
Sho E, Sho M, Nanjo H, et al., 2004, Hemodynamic regulation of CD34+ cell localization and differentiation in experimental aneurysms. Arterioscler Thromb Vasc Biol, 24: 1916–1921. https://doi.org/10.1161/01.Atv.0000142805.20398.74
Gong J, Zhou D, Jiang L, et al., 2020, In vitro lineage-specific differentiation of vascular smooth muscle cells in response to SMAD3 deficiency: Implications for SMAD3-related thoracic aortic aneurysm. Arterioscler Thromb Vasc Biol, 40: 1651–1663. https://doi.org/10.1161/atvbaha.120.313033
Wang G, Jacquet L, Karamariti E, et al., 2015, Origin and differentiation of vascular smooth muscle cells. J Physiol, 593: 3013–3030. https://doi.org/10.1113/jp270033
Pan H, Xue C, Auerbach BJ, et al., 2020, Single-cell genomics reveals a novel cell state during smooth muscle cell phenotypic switching and potential therapeutic targets for atherosclerosis in mouse and human. Circulation, 142: 2060–2075. https://doi.org/10.1161/circulationaha.120.048378
Chen PY, Qin L, Li G, et al., 2020, Smooth muscle cell reprogramming in aortic aneurysms. Cell Stem Cell, 26: 542–557.e511. https://doi.org/10.1016/j.stem.2020.02.013
Lu S, Jolly AJ, Strand KA, et al., 2020, Smooth muscle-derived progenitor cell myofibroblast differentiation through KLF4 downregulation promotes arterial remodeling and fibrosis. JCI Insight, 5: e139445. https://doi.org/10.1172/jci.insight.139445
Cao J, Yang Z, Xiao R, et al., 2020, Regenerative potential of pluripotent nontumorgenetic stem cells: Multilineage differentiating stress enduring cells (Muse cells). Regen Ther, 15: 92–96. https://doi.org/10.1016/j.reth.2020.04.011
Peng GY, Lin Y, Li JJ, et al., 2019, The application of induced pluripotent stem cells in pathogenesis study and gene therapy for vascular disorders: Current progress and future challenges. Stem Cells Int, 2019: 9613258. https://doi.org/10.1155/2019/9613258
Granata A, Serrano F, Bernard WG, et al., 2017, An iPSC-derived vascular model of Marfan syndrome identifies key mediators of smooth muscle cell death. Nat Genet, 49: 97–109. https://doi.org/10.1038/ng.3723
Wang Y, Yin P, Bian GL, et al., 2017, The combination of stem cells and tissue engineering: An advanced strategy for blood vessels regeneration and vascular disease treatment. Stem Cell Res Ther, 8: 194. https://doi.org/10.1186/s13287-017-0642-y
Rurali E, Perrucci GL, Pilato CA, et al., 2018, Precise therapy for thoracic aortic aneurysm in Marfan syndrome: A puzzle nearing its solution. Prog Cardiovasc Dis, 61: 328–335. https://doi.org/10.1016/j.pcad.2018.07.020
Hu K, Li J, Zhu K, et al., 2017, Generation of an induced pluripotent stem cell line from a Loeys-Dietz syndrome patient with transforming growth factor-beta receptor-2 gene mutation. Stem Cell Res, 20: 115–117. https://doi.org/10.1016/j.scr.2017.03.012
Williams IM, Wu JC, 2019, Generation of endothelial cells from human pluripotent stem cells. Arterioscler Thromb Vasc Biol, 39: 1317–1329. https://doi.org/10.1161/atvbaha.119.312265
Iida Y, Sawa S, Shimizu H, 2018, Optimal time for pharmacological treatment of abdominal aortic aneurysm. Curr Drug Targets, 19: 1297–1301. https://doi.org/10.2174/1389450119666171227225008
Hashizume R, Yamawaki-Ogata A, Ueda Y, et al., 2011, Mesenchymal stem cells attenuate angiotensin II-induced aortic aneurysm growth in apolipoprotein E-deficient mice. J Vasc Surg, 54: 1743–1752. https://doi.org/10.1016/j.jvs.2011.06.109
Li X, Wen H, Lv J, et al., 2022, Therapeutic efficacy of mesenchymal stem cells for abdominal aortic aneurysm: A meta-analysis of preclinical studies. Stem Cell Res Ther, 13: 81. https://doi.org/10.1186/s13287-022-02755-w
Li K, Vela D, Migliati E, et al., 2021, Pilot study of endovascular delivery of mesenchymal stromal cells in the aortic wall in a pig model. Cell Transplant, 30: 9636897211010652. https://doi.org/10.1177/09636897211010652
Wen H, Wang M, Gong S, et al., 2020, Human umbilical cord mesenchymal stem cells attenuate abdominal aortic aneurysm progression in Sprague-Dawley rats: Implication of vascular smooth muscle cell phenotypic modulation. Stem Cells Dev, 29: 981–993. https://doi.org/10.1089/scd.2020.0058
Dahal S, Swaminathan G, Carney S, et al., 2020, Pro-elastogenic effects of mesenchymal stem cell derived smooth muscle cells in a 3D collagenous milieu. Acta Biomater, 105: 180–190. https://doi.org/10.1016/j.actbio.2020.01.030
Sivaraman S, Hedrick J, Ismail S, et al., 2021, Generation and characterization of human mesenchymal stem cell-derived smooth muscle cells. Int J Mol Sci, 22: 10335. https://doi.org/10.3390/ijms221910335
Kozakai M, Narita Y, Yamawaki-Ogata A, et al., 2022, Alternative therapeutic strategy for existing aortic aneurysms using mesenchymal stem cell-derived exosomes. Expert Opin Biol Ther, 22: 95–104. https://doi.org/10.1080/14712598.2022.2005575
Hosoyama K, Wakao S, Kushida Y, et al., 2018, Intravenously injected human multilineage-differentiating stress-enduring cells selectively engraft into mouse aortic aneurysms and attenuate dilatation by differentiating into multiple cell types. J Thorac Cardiovasc Surg, 155: 2301–2313.e4. https://doi.org/10.1016/j.jtcvs.2018.01.098
Hosoyama K, Saiki Y, 2018, Muse cells and aortic aneurysm. Adv Exp Med Biol, 1103: 273–291. https://doi.org/10.1007/978-4-431-56847-6_15
Parvizi M, Harmsen MC, 2015, Therapeutic prospect of adipose-derived stromal cells for the treatment of abdominal aortic aneurysm. Stem Cells Dev, 24: 1493–1505. https://doi.org/10.1089/scd.2014.0517
Blose KJ, Ennis TL, Arif B, et al., 2014, Periadventitial adipose-derived stem cell treatment halts elastase-induced abdominal aortic aneurysm progression. Regen Med, 9: 733– 741. https://doi.org/10.2217/rme.14.61
Zilberman B, Kooragayala K, Lou J, et al., 2022, Treatment of abdominal aortic aneurysm utilizing adipose-derived mesenchymal stem cells in a porcine model. J Surg Res, 278: 247–256. https://doi.org/10.1016/j.jss.2022.04.064
Mulorz J, Shayan M, Hu C, et al., 2021, peri-Adventitial delivery of smooth muscle cells in porous collagen scaffolds for treatment of experimental abdominal aortic aneurysm. Biomater Sci, 9: 6903–6914. https://doi.org/10.1039/d1bm00685a
Shen M, Liu C, Wu JC, 2022, Generation of embryonic origin-specific vascular smooth muscle cells from human induced pluripotent stem cells. Methods Mol Biol, 2429: 233–246. https://doi.org/10.1007/978-1-0716-1979-7_15
Doss MX, Sachinidis A, 2019, Current challenges of iPSC-based disease modeling and therapeutic implications. Cells, 8: 403. https://doi.org/10.3390/cells8050403
Davis JP, Salmon M, Pope NH, et al., 2015, Attenuation of aortic aneurysms with stem cells from different genders. J Surg Res, 199: 249–258. https://doi.org/10.1016/j.jss.2015.04.025
Sharma AK, Salmon MD, Lu G, et al., 2016, Mesenchymal stem cells attenuate NADPH oxidase-dependent high mobility group box 1 production and inhibit abdominal aortic aneurysms. Arterioscler Thromb Vasc Biol, 36: 908–918. https://doi.org/10.1161/atvbaha.116.307373
Schneider F, Saucy F, de Blic R, et al., 2013, Bone marrow mesenchymal stem cells stabilize already-formed aortic aneurysms more efficiently than vascular smooth muscle cells in a rat model. Eur J Vasc Endovasc Surg, 45: 666–672. https://doi.org/10.1016/j.ejvs.2013.03.007
Parvizi M, Petersen AH, van Spreuwel-Goossens CA, et al., 2018, Perivascular scaffolds loaded with adipose tissue-derived stromal cells attenuate development and progression of abdominal aortic aneurysm in rats. J Biomed Mater Res A, 106, 2494–2506. https://doi.org/10.1002/jbm.a.36445
Tian X, Fan J, Yu M, et al., 2014, Adipose stem cells promote smooth muscle cells to secrete elastin in rat abdominal aortic aneurysm. PLoS One, 9: e108105. https://doi.org/10.1371/journal.pone.0108105
Yamawaki-Ogata A, Fu X, Hashizume R, et al., 2014, Therapeutic potential of bone marrow-derived mesenchymal stem cells in formed aortic aneurysms of a mouse model. Eur J Cardiothorac Surg, 45: e156–e165. https://doi.org/10.1093/ejcts/ezu018
Fu XM, Yamawaki-Ogata A, Oshima H, et al., 2013, Intravenous administration of mesenchymal stem cells prevents angiotensin II-induced aortic aneurysm formation in apolipoprotein E-deficient mouse. J Transl Med, 11, 175. https://doi.org/10.1186/1479-5876-11-175
Yamawaki-Ogata A, Oshima H, Usui A, et al., 2017, Bone marrow-derived mesenchymal stromal cells regress aortic aneurysm via the NF-kB, Smad3 and Akt signaling pathways. Cytotherapy, 19: 1167–1175. https://doi.org/10.1016/j.jcyt.2017.07.010
Zidi M, Allaire E, 2015, Mechanical behavior of abdominal aorta aneurysm in rat model treated by cell therapy using mesenchymal stem cells. Biomech Model Mechanobiol, 14: 185–194. https://doi.org/10.1007/s10237-014-0586-4
Wang SK, Green LA, Gutwein AR, et al., 2018, Rationale and design of the ARREST trial investigating mesenchymal stem cells in the treatment of small abdominal aortic aneurysm. Ann Vasc Surg, 47: 230–237. https://doi.org/10.1016/j.avsg.2017.08.044