Fusion genes as diagnostic and predictive biomarkers for tumor
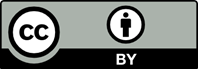
Structural variants, including chromosomal rearrangements and translocations, may result in the formation of fusion genes. Glioma is the most frequent brain tumor among adults. Due to complex tumor classifications, characterization of recurrence, inadequate sample size and controversial mechanisms of tumor malignancy, clinical strategies have not been developed for almost 30 years. Fusion gene is one of the strong driver events in glioma tumorigenesis and has provided fundamental insights into the disease mechanisms. This review elucidates the literature on the discovery of fusion genes, the development of detection techniques, and their clinical implementations. In conclusion, fusion genes are important diagnostic and predictive biomarkers for brain tumors.
Siegel RL, Miller KD, Jemal A, 2019, Cancer statistics, 2019. CA Cancer J Clin, 69(1): 7–34. https://doi.org/10.3322/caac.21551
Jiang B, Liu H, Sun D, et al., 2021, Mortality due to primary brain tumours in China and detection rate in people with suspected symptoms: A nationally representative cross-sectional survey. World J Surg Oncol, 19(1): 71. https://doi.org/10.1186/s12957-021-02179-5
Miller KD, Ostrom QT, Kruchko C, et al., 2021, Brain and other central nervous system tumor statistics, 2021. CA Cancer J Clin, 71(5): 381–406. https://doi.org/10.3322/caac.21693
Vickers AJ, 2011, Prediction models in cancer care. CA Cancer J Clin, 61(5): 315–326.
Wang Y, Jiang T, 2013, Understanding high grade glioma: Molecular mechanism, therapy and comprehensive management. Cancer Lett, 331(2): 139–146. https://doi.org/10.1016/j.canlet.2012.12.024
Louis DN, Ohgaki H, Wiestler OD, et al., 2007, The 2007 WHO classification of tumours of the central nervous system. Acta Neuropathol, 114(2): 97–109. https://doi.org/10.1007/s00401-007-0243-4
Louis DN, Perry A, Reifenberger G, et al., 2016, The 2016 World Health Organization classification of tumors of the central nervous system: A summary. Acta Neuropathol, 131(6): 803–820. https://doi.org/10.1007/s00401-016-1545-1
Stupp R, Mason WP, van den Bent MJ, et al., 2005, Radiotherapy plus concomitant and adjuvant temozolomide for glioblastoma. N Engl J Med, 352(10): 987–996. https://doi.org/10.1097/01.cot.0000289242.47980.f9
Agarwala SS, Kirkwood JM, 2000, Temozolomide, a novel alkylating agent with activity in the central nervous system, may improve the treatment of advanced metastatic melanoma. Oncologist, 5(2): 144–151. https://doi.org/10.1634/theoncologist.5-2-144
National Comprehensive Cancer Network, 2019, CNS Cancers (Version1. 2019). National Comprehensive Cancer Network.
Jiang T, Mao Y, Ma W, et al., 2016, CGCG clinical practice guidelines for the management of adult diffuse gliomas. Cancer Lett, 375(2): 263–273.
Mertens F, Johansson B, Fioretos T, et al., 2015, The emerging complexity of gene fusions in cancer. Nat Rev Cancer, 15(6): 371–381. https://doi.org/10.1038/nrc3947
Singh D, Chan JM, Zoppoli P, et al., 2012, Transforming fusions of FGFR and TACC genes in human glioblastoma. Science, 337(6099): 1231–1235. https://doi.org/10.1126/science.1220834
Bandopadhayay P, Ramkissoon LA, Jain P, et al., 2016, MYB-QKI rearrangements in angiocentric glioma drive tumorigenicity through a tripartite mechanism. Nat Genet, 48(3): 273–282. https://doi.org/10.1158/1538-7445.am2016-4372
Bao ZS, Chen HM, Yang MY, et al., 201, RNA-seq of 272 gliomas revealed a novel, recurrent PTPRZ1-MET fusion transcript in secondary glioblastomas. Genome Res, 24(11): 1765–1773. https://doi.org/10.1101/gr.165126.113
Mitelman F, Johansson B, Mertens F, 200, Fusion genes and rearranged genes as a linear function of chromosome aberrations in cancer. Nat Genet, 36(4): 331–334. https://doi.org/10.1038/ng1335
Mani RS, Chinnaiyan AM, 2010, Triggers for genomic rearrangements: insights into genomic, cellular and environmental influences. Nat Rev Genet, 11(12): 819–829. https://doi.org/10.1038/nrg2883
Hakim O, Resch W, Yamane A, et al., 2012, DNA damage defines sites of recurrent chromosomal translocations in B lymphocytes. Nature, 484(7392): 69–74. https://doi.org/10.1038/nature10909
Zhang Y, McCord RP, Ho YJ, et al., 2012, Spatial organization of the mouse genome and its role in recurrent chromosomal translocations. Cell, 148(5): 908–921. https://doi.org/10.1016/j.cell.2012.02.002
Nowell PC, 1962, The minute chromosome (Phl) in chronic granulocytic leukemia. Blut, 8: 65–66. https://doi.org/10.1007/bf01630378
Caspersson T, Zech L, Johansson C, 1970, Differential binding of alkylating fluorochromes in human chromosomes. Exp Cell Res, 60(3): 315–319. https://doi.org/10.1016/0014-4827(70)90523-9
Rowley JD, 1973, Letter: A new consistent chromosomal abnormality in chronic myelogenous leukaemia identified by quinacrine fluorescence and Giemsa staining. Nature, 243(5405): 290–293. https://doi.org/10.1038/243290a0
Miyoshi I, Hiraki S, Kimura I, et al., 1979, 2/8 translocation in a Japanese Burkitt’s lymphoma. Experientia, 35(6): 742–743. https://doi.org/10.1007/bf01968217
Rowley JD, Golomb HM, Dougherty C, 1977, 15/17 translocation, a consistent chromosomal change in acute promyelocytic leukaemia. Lancet, 1(8010): 549–550. https://doi.org/10.1016/s0140-6736(77)91415-5
Wachtel M, Dettling M, Koscielniak E, et al., 200, Gene expression signatures identify rhabdomyosarcoma subtypes and detect a novel t(2;2)(q35;p23) translocation fusing PAX3 to NCOA1. Cancer Res, 64(16): 5539–5545. https://doi.org/10.1158/0008-5472.can-04-0844
Sinclair PB, Nacheva EP, Leversha M, et al., 2000, Large deletions at the t(9;22) breakpoint are common and may identify a poor-prognosis subgroup of patients with chronic myeloid leukemia. Blood, 95(3): 738–743. https://doi.org/10.1182/blood.v95.3.738.003k21_738_743
Soda M, Choi YL, Enomoto M, et al., 2007, Identification of the transforming EML4-ALK fusion gene in non-small-cell lung cancer. Nature, 448(7153): 561–566. https://doi.org/10.1038/nature05945
Eck MJ, Manley PW, 2009, The interplay of structural information and functional studies in kinase drug design: Insights from BCR-Abl. Curr Opin Cell Biol, 21(2): 288–295. https://doi.org/10.1016/j.ceb.2009.01.014
Takahashi M, Ritz J, Cooper GM, 1985, Activation of a novel human transforming gene, ret, by DNA rearrangement. Cell, 42(2): 581–588. https://doi.org/10.1016/0092-8674(85)90115-1
Tognon C, Knezevich SR, Huntsman D, et al., 2002, Expression of the ETV6-NTRK3 gene fusion as a primary event in human secretory breast carcinoma. Cancer Cell, 2(5): 367–376. https://doi.org/10.1016/s1535-6108(02)00180-0
Zinszner H, Albalat R, Ron D, 1994, A novel effector domain from the RNA-binding protein TLS or EWS is required for oncogenic transformation by CHOP. Genes Dev, 8(21): 2513–2526. https://doi.org/10.1101/gad.8.21.2513
Linden M, Thomsen C, Grundevik P, et al., 2019, FET family fusion oncoproteins target the SWI/SNF chromatin remodeling complex. EMBO Rep, 20(5): e45766. https://doi.org/10.15252/embr.201845766
Linden M, Vannas C, Osterlund T, et al., 2022, FET fusion oncoproteins interact with BRD4 and SWI/SNF chromatin remodelling complex subtypes in sarcoma. Mol Oncol, Online ahead of print. https://doi.org/10.1002/1878-0261.13195/v2/review2
Chong S, Graham TG, Dugast-Darzacq C, et al., 2022, Tuning levels of low-complexity domain interactions to modulate endogenous oncogenic transcription. Mol Cell, 2022: 00318–5. https://doi.org/10.1016/j.molcel.2022.04.007
Hondele M, Heinrich S, De Los Rios P, et al., 2020, Membraneless organelles: Phasing out of equilibrium. Emerg Top Life Sci, 4(3): 331–342. https://doi.org/10.1042/etls20190190
Davis RB, Moosa MM, Banerjee PR, 2022, Ectopic biomolecular phase transitions: fusion proteins in cancer pathologies. Trends Cell Biol, 2022: 00077–0. https://doi.org/10.1016/j.tcb.2022.03.005
Saraon P, Pathmanathan S, Snider J, et al., 2021, Receptor tyrosine kinases and cancer: Oncogenic mechanisms and therapeutic approaches. Oncogene, 40(24): 4079–4093. https://doi.org/10.1038/s41388-021-01841-2
Fawal M, Espinos E, Jean-Jean O, et al., 2011, Looking for the functions of RNA granules in ALK–transformed cells. Bioarchitecture, 1(2): 91–95. https://doi.org/10.4161/bioa.1.2.16269
Hrustanovic G, Olivas V, Pazarentzos E, et al., 2015, RAS-MAPK dependence underlies a rational polytherapy strategy in EML4-ALK-positive lung cancer. Nat Med, 21(9): 1038–1047. https://doi.org/10.1038/nm.3930
Richards MW, O’Regan L, Roth D, et al., 2015, Microtubule association of EML proteins and the EML4-ALK variant 3 oncoprotein require an N-terminal trimerization domain. Biochem J, 467(3): 529–536. https://doi.org/10.1042/bj20150039
Sampson J, Richards MW, Choi J, et al., 2021, Phase-separated foci of EML4-ALK facilitate signalling and depend upon an active kinase conformation. EMBO Rep, 22(12): e53693. https://doi.org/10.15252/embr.202153693
Tulpule A, Guan J, Neel DS, et al., 2021, Kinase-mediated RAS signaling via membraneless cytoplasmic protein granules. Cell, 184(10): 2649–2664.e2618. https://doi.org/10.1016/j.cell.2021.03.031
Zhang JZ, Lu TW, Stolerman LM, et al., 2020, Phase separation of a PKA regulatory subunit controls camp compartmentation and oncogenic signaling. Cell, 182(6): 1531–1544.e1515. https://doi.org/10.1016/j.cell.2020.07.043
Honeyman JN, Simon EP, Robine N, et al., 2014, Detection of a recurrent DNAJB1-PRKACA chimeric transcript in fibrolamellar hepatocellular carcinoma. Science, 343(6174): 1010–1014. https://doi.org/10.1126/science.1249484
Corpet A, Kleijwegt C, Roubille S, et al., 2020, PML nuclear bodies and chromatin dynamics: catch me if you can! Nucleic Acids Res, 48(21): 11890–11912. https://doi.org/10.1093/nar/gkaa828
Dyck JA, Maul GG, Miller WH Jr., et al., 1994, A novel macromolecular structure is a target of the promyelocyte-retinoic acid receptor oncoprotein. Cell, 76(2): 333–343. https://doi.org/10.1016/0092-8674(94)90340-9
di Masi A, Cilli D, Berardinelli F, et al., 2016, PML nuclear body disruption impairs DNA double-strand break sensing and repair in APL. Cell Death Dis, 7: e2308. https://doi.org/10.1038/cddis.2016.115
Salesse S, Verfaillie CM, 2002, BCR/ABL: From molecular mechanisms of leukemia induction to treatment of chronic myelogenous leukemia. Oncogene, 21(56): 8547–8559. https://doi.org/10.1038/sj.onc.1206082
Kashiwagi S, Fujioka Y, Kondo T, et al., 2019, Localization of BCR-ABL to stress granules contributes to its oncogenic function. Cell Struct Funct, 44(2): 195–204. https://doi.org/10.1247/csf.19033
Tomlins SA, Rhodes DR, Perner S, et al., 2005, Recurrent fusion of TMPRSS2 and ETS transcription factor genes in prostate cancer. Science, 310(5748): 644–648. https://doi.org/10.1126/science.1117679
Wang X, Qiao Y, Asangani IA, et al., 2017, Development of peptidomimetic inhibitors of the ERG gene fusion product in prostate cancer. Cancer Cell, 31(4): 532–548.e537. https://doi.org/10.1016/j.ccell.2017.02.017
Camidge DR, Dziadziuszko R, Peters S, et al., 2019, Updated efficacy and safety data and impact of the EML4-ALK fusion variant on the efficacy of alectinib in untreated ALK-positive advanced non-small cell lung cancer in the global phase III ALEX study. J Thorac Oncol, 14(7): 1233–1243. https://doi.org/10.1016/j.jtho.2019.03.007
Gong J, Gregg JP, Ma W, et al., 2019, Squamous cell transformation of primary lung adenocarcinoma in a patient with EML4-ALK fusion variant 5 refractory to ALK inhibitors. J Natl Compr Canc Netw, 17(4): 297–301. https://doi.org/10.6004/jnccn.2019.7291
Kohsaka S, Hayashi T, Nagano M, et al., 2020, Identification of Novel CD74-NRG2alpha fusion from comprehensive profiling of lung adenocarcinoma in Japanese never or light smokers. J Thorac Oncol, 15(6): 948–961. https://doi.org/10.1016/j.jtho.2020.01.021
Jerby-Arnon L, Neftel C, Shore ME, et al., 2021, Opposing immune and genetic mechanisms shape oncogenic programs in synovial sarcoma. Nat Med, 27: 289–300.
Karajannis MA, Legault G, Fisher MJ, et al., 201, Phase II study of sorafenib in children with recurrent or progressive low-grade astrocytomas. Neuro Oncol, 16(10): 1408–1416. https://doi.org/10.1093/neuonc/nou059
Helgager J, Lidov HG, Mahadevan NR, et al., 2017, A novel GIT2-BRAF fusion in pilocytic astrocytoma. Diagn Pathol, 12(1): 82. https://doi.org/10.1186/s13000-017-0669-5
Jones DT, Hutter B, Jager N, et al., 2013, Recurrent somatic alterations of FGFR1 and NTRK2 in pilocytic astrocytoma. Nat Genet, 45(8): 927–932.
Qaddoumi I, Orisme W, Wen J, et al., 2016, Genetic alterations in uncommon low-grade neuroepithelial tumors: BRAF, FGFR1, and MYB mutations occur at high frequency and align with morphology. Acta Neuropathol, 131(6): 833–845. https://doi.org/10.1093/neuonc/now075.10
Ryall S, Zapotocky M, Fukuoka K, et al., 2020, Integrated molecular and clinical analysis of 1,000 pediatric low-grade gliomas. Cancer Cell, 37(4): 569–583.e565.
Tomic TT, Olausson J, Wilzen A, et al., 2017, A new GTF2I-BRAF fusion mediating MAPK pathway activation in pilocytic astrocytoma. PLoS One, 12(4): e0175638. https://doi.org/10.1371/journal.pone.0175638
Zhang J, Wu G, Miller CP, et al., 2013, Whole-genome sequencing identifies genetic alterations in pediatric low-grade gliomas. Nat Genet, 45(6): 602–612. https://doi.org/10.1038/ng.2611
Weinberg F, Griffin R, Frohlich M, et al., 2020, Identification and characterization of a BRAF fusion oncoprotein with retained autoinhibitory domains. Oncogene, 39(4): 814–832. https://doi.org/10.1038/s41388-019-1021-1
Fangusaro J, Onar-Thomas A, Poussaint TY, et al., 2019, Selumetinib in paediatric patients with BRAF-aberrant or neurofibromatosis Type 1-associated recurrent, refractory, or progressive low-grade glioma: A multicentre, phase 2 trial. Lancet Oncol, 20(7): 1011–1022. https://doi.org/10.1016/s1470-2045(19)30277-3
Sievert AJ, Lang SS, Boucher KL, et al., 2013, Paradoxical activation and RAF inhibitor resistance of BRAF protein kinase fusions characterizing pediatric astrocytomas. Proc Natl Acad Sci U S A, 110(15): 5957–5962. https://doi.org/10.1073/pnas.1307863110
Lawrence MS, Stojanov P, Mermel CH, et al., 2014, Discovery and saturation analysis of cancer genes across 21 tumour types. Nature, 505(7484): 495–501. https://doi.org/10.1038/nature12912
Hallberg B, Palmer RH, 2016, The role of the ALK receptor in cancer biology. Ann Oncol, 27 Suppl 3: iii4–iii15. https://doi.org/10.1093/annonc/mdw301
Childress MA, Himmelberg SM, Chen H, et al., 2018, ALK fusion partners impact response to ALK inhibition: Differential effects on sensitivity, cellular phenotypes, and biochemical properties. Mol Cancer Res, 16(11): 1724–1736. https://doi.org/10.1158/1541-7786.mcr-18-0171
Soda M, Takada S, Takeuchi K, et al., 2008, A mouse model for EML4-ALK-positive lung cancer. Proc Natl Acad Sci U S A, 105(50): 19893–19897. https://doi.org/10.1073/pnas.0805381105
Clarke M, Mackay A, Ismer B, et al., 2020, Infant high-grade gliomas comprise multiple subgroups characterized by novel targetable gene fusions and favorable outcomes. Cancer Discov, 10(7): 942–963.
Nguyen KT, Zong CS, Uttamsingh S, et al., 2002, The role of phosphatidylinositol 3-kinase, rho family GTPases, and STAT3 in Ros-induced cell transformation. J Biol Chem, 277(13): 11107–11115. https://doi.org/10.1074/jbc.m108166200
Charest A, Kheifets V, Park J, et al., Oncogenic targeting of an activated tyrosine kinase to the Golgi apparatus in a glioblastoma. Proc Natl Acad Sci U S A, 100(3): 916–921. https://doi.org/10.1073/pnas.242741799
Uguen A, De Braekeleer M, 2016, ROS1 fusions in cancer: A review. Future Oncol, 12(16): 1911–1928. https://doi.org/10.2217/fon-2016-0050
Wu G, Diaz AK, Paugh BS, et al., 2014, The genomic landscape of diffuse intrinsic pontine glioma and pediatric non-brainstem high-grade glioma. Nat Genet, 46(5): 444–450. https://doi.org/10.1038/ng.2938
Solomon JP, Benayed R, Hechtman JF, et al., 2019, Identifying patients with NTRK fusion cancer. Ann Oncol, 30 Suppl 8: viii16–viii22. https://doi.org/10.1093/annonc/mdz384
Klein R, Smeyne RJ, Wurst W, et al., 1993, Targeted disruption of the trkB neurotrophin receptor gene results in nervous system lesions and neonatal death. Cell, 75(1): 113–122. https://doi.org/10.1016/s0092-8674(05)80088-1
International Cancer Genome Consortium PedBrain Tumor P, 2016, Recurrent MET fusion genes represent a drug target in pediatric glioblastoma. Nat Med, 22(11): 1314–1320. https://doi.org/10.1038/nm.4204
Johnson A, Severson E, Gay L, et al., 2017, Comprehensive genomic profiling of 282 pediatric low-and high-grade gliomas reveals genomic drivers, tumor mutational burden, and hypermutation signatures. Oncologist, 22(12): 1478–1490. https://doi.org/10.1634/theoncologist.2017-0242
Morrison KB, Tognon CE, Garnett MJ, et al., 2002, ETV6- NTRK3 transformation requires insulin-like growth factor 1 receptor signaling and is associated with constitutive IRS-1 tyrosine phosphorylation. Oncogene, 21(37): 5684–5695. https://doi.org/10.1038/sj.onc.1205669
Nelson KN, Meyer AN, Siari A, et al., 2016, Oncogenic gene fusion FGFR3-TACC3 is regulated by tyrosine phosphorylation. Mol Cancer Res, 14(5): 458–469. https://doi.org/10.1158/1541-7786.mcr-15-0497
Parker BC, Annala MJ, Cogdell DE, et al., 2013, The tumorigenic FGFR3-TACC3 gene fusion escapes miR-99a regulation in glioblastoma. J Clin Invest, 123(2): 855–865. https://doi.org/10.1158/1538-7445.am2013-5311
Frattini V, Pagnotta SM, Tala, et al., 2018, A metabolic function of FGFR3-TACC3 gene fusions in cancer. Nature, 553(7687): 222–227. https://doi.org/10.1038/nature25171
Hu H, Mu Q, Bao Z, et al., 2018, Mutational landscape of secondary glioblastoma guides MET-targeted trial in brain tumor. Cell, 175(6): 1665–1678.e1618.
Davare MA, Henderson JJ, Agarwal A, et al., 2018, Rare but recurrent ROS1 fusions resulting from chromosome 6q22 microdeletions are targetable oncogenes in glioma. Clin Cancer Res, 24(24): 6471–6482. https://doi.org/10.1158/1078-0432.ccr-18-1052
Matjasic A, Zupan A, Bostjancic E, et al., 2002, A novel PTPRZ1– ETV1 fusion in gliomas. Brain Pathol, 30(2): 226–234. https://doi.org/10.1111/bpa.12776
Mak HH, Peschard P, Lin T, et al., 2007, Oncogenic activation of the Met receptor tyrosine kinase fusion protein, Tpr-Met, involves exclusion from the endocytic degradative pathway. Oncogene, 26(51): 7213–7221. https://doi.org/10.1038/sj.onc.1210522
Hernandez L, Pinyol M, Hernandez S, et al., 1999, TRK-fused gene (TFG) is a new partner of ALK in anaplastic large cell lymphoma producing two structurally different TFG-ALK translocations. Blood, 94(9): 3265–3268. https://doi.org/10.1182/blood.v94.9.3265
Slotkin EK, Diolaiti D, Shukla NN, et al., 2019, Patient-driven discovery, therapeutic targeting, and post-clinical validation of a novel AKT1 fusion-driven cancer. Cancer Discov, 9(5): 605–616. https://doi.org/10.1158/2159-8290.cd-18-0953
Choudhury NJ, Drilon A, 2020, Decade in review: A new era for RET-rearranged lung cancers. Transl Lung Cancer Res, 9(6): 2571–2580. https://doi.org/10.21037/tlcr-20-346
Ziegler DS, Wong M, Mayoh C, et al., 2018, Brief report: Potent clinical and radiological response to larotrectinib in TRK fusion-driven high-grade glioma. Br J Cancer, 119(6): 693–696. https://doi.org/10.1038/s41416-018-0251-2
Drilon A, 2019, TRK inhibitors in TRK fusion-positive cancers. Ann Oncol, 30 Suppl 8: viii23–viii30. https://doi.org/10.1093/annonc/mdz282
Li Z, Shen L, Ding D, et al., 2018, Efficacy of crizotinib among different types of ROS1 fusion partners in patients with ROS1-rearranged non-small cell lung cancer. J Thorac Oncol, 13(7): 987–995. https://doi.org/10.1016/j.jtho.2018.04.016
Forschner A, Forchhammer S, Bonzheim I, 2020, NTRK gene fusions in melanoma: Detection, prevalence and potential therapeutic implications. J Dtsch Dermatol Ges, 18(12): 1387–1392. https://doi.org/10.1111/ddg.14160
Goyal L, Saha SK, Liu LY, et al., 2017, Polyclonal secondary FGFR2 mutations drive acquired resistance to FGFR inhibition in patients with FGFR2 fusion-positive cholangiocarcinoma. Cancer Discov, 7(3): 252–263. https://doi.org/10.1158/1538-7445.am2017-4114
Rosen EY, Johnson ML, Clifford SE, et al., 2021, Overcoming MET-dependent resistance to selective RET inhibition in patients with RET fusion-positive lung cancer by combining selpercatinib with crizotinib. Clin Cancer Res, 27(1): 34–42. https://doi.org/10.1158/1078-0432.ccr-20-2278
Kulkarni A, Al-Hraishawi H, Simhadri S, et al., 2017, BRAF fusion as a novel mechanism of acquired resistance to vemurafenib in BRAF(V600E) mutant melanoma. Clin Cancer Res, 23(18): 5631–5638. https://doi.org/10.1158/1078-0432.ccr-16-0758
Available from: https://www.foundationmedicine.com/genomic-testing [Last accessed on 2019 Feb 01].
Song Z, Liu T, Shi L, et al., 2021, The deep learning model combining CT image and clinicopathological information for predicting ALK fusion status and response to ALK-TKI therapy in non-small cell lung cancer patients. Eur J Nucl Med Mol Imaging, 48(2): 361–371. https://doi.org/10.1007/s00259-020-04986-6
Zhang Y, Li J, Yi K, et al., 2019, Elevated signature of a gene module coexpressed with CDC20 marks genomic instability in glioma. Proc Natl Acad Sci U S A, 116(14): 6975–6984. https://doi.org/10.1073/pnas.1921769117
Drilon A, Rekhtman N, Arcila M, et al., 2016, Cabozantinib in patients with advanced RET-rearranged non-small-cell lung cancer: An open-label, single-centre, phase 2, single-arm trial. Lancet Oncol, 17(12): 1653–1660. https://doi.org/10.1016/s1470-2045(16)30562-9
Aggarwal C, Redman MW, Lara PN Jr., et al., 2019, SWOG S1400D (NCT02965378), a phase II study of the fibroblast growth factor receptor inhibitor AZD4547 in previously treated patients with fibroblast growth factor pathway-activated stage IV squamous cell lung cancer (lung-MAP substudy). J Thorac Oncol, 14(10): 1847–1852. https://doi.org/10.1016/j.jtho.2019.05.041
Drilon A, Siena S, Dziadziuszko R, et al., 2020, Entrectinib in ROS1 fusion-positive non-small-cell lung cancer: Integrated analysis of three phase 1-2 trials. Lancet Oncol, 21(2): 261–270.
Drilon A, Nagasubramanian R, Blake JF, et al., 2017, A next-generation TRK kinase inhibitor overcomes acquired resistance to prior TRK kinase inhibition in patients with TRK fusion-positive solid tumors. Cancer Discov, 7(9): 963–972. https://doi.org/10.1158/2159-8290.cd-17-0507
Drilon A, Laetsch TW, Kummar S, et al., 2018, Efficacy of larotrectinib in TRK fusion-positive cancers in adults and children. N Engl J Med, 378(8): 731–739.
Hong DS, Bauer TM, Lee JJ, et al., 2019, Larotrectinib in adult patients with solid tumours: A multi-centre, open-label, phase I dose-escalation study. Ann Oncol, 30(2): 325–331. https://doi.org/10.1093/annonc/mdy539
Hong DS, DuBois SG, Kummar S, et al., 2020, Larotrectinib in patients with TRK fusion-positive solid tumours: A pooled analysis of three phase 1/2 clinical trials. Lancet Oncol, 21(4): 531–540. https://doi.org/10.1016/s1470-2045(19)30856-3
Zhao Z, Chen J, Bao Z, et al., 2020, Extensive MET alterations confer clinical response to MET inhibitors in gliomas. BioRxiv, 2020: 364711.
Laetsch TW, DuBois SG, Mascarenhas L, et al., 2018, Larotrectinib for paediatric solid tumours harbouring NTRK gene fusions: Phase 1 results from a multicentre, open-label, phase 1/2 study. Lancet Oncol, 19(5): 705–714. https://doi.org/10.1016/s1470-2045(18)30119-0