Influence of variations in photon beam quality index on tissue inhomogeneity correction factors in radiation therapy treatment planning
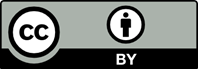
This study was carried out to investigate how variations in the beam quality index (QI)-so-called tissue phantom ratio (TPR20,10) affect tissue inhomogeneity correction factors (ICFs) in external beam radiotherapy treatment plans. A total of 90 three-dimensional conformal radiotherapy (3DCRT) treatment plans for lung, gynecological, and prostate cancers and 15 stereotactic body radiation therapy (SBRT) plans for lung cancer were analyzed. For the 3DCRT plans, ICFs were evaluated across a range of beam QI values. For 6 MV photon beams, the TPR20,10 values were set at 0.670 ± 3%, while for 15 MV photon beams, the range was 0.760 ± 3%. SBRT plans were generated using two 6 MV photon beam configurations from a Varian TrueBeam accelerator – one employing a flattening filter (6 MV, TPR20,10 = 0.688) and the other operating in flattening filter-free (FFF) mode (6 MV FFF, TPR20,10 = 0.632). All dose calculations were performed using the Eclipse treatment planning system with the anisotropic analytical algorithm. For the calculations for 3DCRT plans, a 6.0% variation in the beam QI resulted in maximum differences in ICFs of 16.3% for lung cases with 6 MV beams and 12.5% for 15 MV beams. In gynecological and prostate cases, the ICF differences remained below 2.0% and 1.0%, respectively. In addition, for lung SBRT plans, a 5.6% discrepancy in TPR20,10 between 6 MV and 6 MV FFF beams led to ICF variations of <3.0%. These findings suggest that while variations in the beam QI significantly influence ICFs in lung cancer 3DCRT plans, their impact is less pronounced in gynecological and prostate treatments. This underscores the necessity of carefully accounting for beam quality variations during radiotherapy treatment planning to ensure accurate dose delivery and optimal patient outcomes.
- Li J, Zhang T. Implementation of convolution/superposition model of photon dose calculation. In: 7th Asian-Pacific Conference on Medical and Biological Engineering. 2008. p. 442-446. doi: 10.1007/978-3-540-79039-6_110
- Wieslander E. Verification of Dose Calculation Algorithms in Treatment Planning Systems for External Radiation Therapy: A Monte Carlo Approach [doctoral thesis]. Sweden: Lund University; 2006.
- Robinson D. Inhomogeneity correction and the analytic anisotropic algorithm. J Appl Clin Med Phys. 2008;9(2): 112-122. doi: 10.1120/jacmp.v9i2.2801.
- International Commission on Radiation Units and Measurements (ICRU). Prescribing, Recording, and Reporting Photon Beam Therapy. ICRU Report No 50; 1993.
- International Commission on Radiation Units and Measurements (ICRU). Prescribing, Recording, and Reporting Photon Beam Therapy (Supplement to ICRU Report 50). ICRU Report No 62; 1999.
- Cheng CW, Das IJ, Tang W, et al. Dosimetric comparison of treatment planning systems in irradiation of breast with tangential fields. Int J Radiat Oncol Biol Phys. 1997;38(4):835-842. doi: 10.1016/S0360-3016(97)00048-8
- Paelinck L, Reynaert N, Thierens H, et al. Experimental verification of lung dose with radiochromic film: Comparison with Monte Carlo simulations and commercially available treatment planning systems. Phys Med Biol. 2005;50(9): 2055-2059. doi: 10.1088/0031-9155/50/9/009.
- Mesbahi A, Zergoug I. Dose calculations for lung inhomogeneity in high-energy photon beams and small beamlets: A comparison between XiO and TiGRT treatment planning systems and MCNPX Monte Carlo code. Iran J Med Phys. 2015;12(3):167-177. doi: 10.22038/ijmp.2015.6218
- International Atomic Energy Agency (IAEA). Commissioning and Quality Assurance of Computerized Planning Systems for Radiation Treatment of Cancer. Technical Report Series No. 430; 2004.
- Papanikolaou N, Battista JJ, Boyer AL, et al. Tissue Inhomogeneity Corrections for Megavoltage Photon Beams. Report of the AAPM Task Group No. 85. Madison, WI: Medical Physics Publishing; 2004.
- Hunt MA, Desobry GE, Fowble B, Coia LR. Effect of low-density lateral interfaces on soft-tissue doses. Int J Radiat Oncol Biol Phys. 1997;37(2):475-482. doi: 10.1016/S0360-3016(96)00477-5
- Stathakis S, Kappas C, Theodorou K, et al. An inhomogeneity correction algorithm for irregular fields of high-energy photon beams based on Clarkson integration and the 3D beam subtraction method. J Appl Clin Med Phys. 2006;7(1):1-13. doi: 10.1120/jacmp.v7i1.2188
- Ono K, Endo S, Tanaka K, et al. Dosimetric verification of the anisotropic analytical algorithm in lung equivalent heterogeneities with and without bone equivalent heterogeneities. Med Phys. 2010;37(8):4456-4463. doi: 10.1118/1.3455709
- Akhtaruzzaman M, Kukolowicz P. Dependence of tissue inhomogeneity correction factors on nominal photon beam energy. Nukleonika. 2018;63(1):3-7. doi: 10.1515/nuka-2018-0001
- Gerbi BJ. A mathematical expression for percentage depth dose accurate from Co-60 to 24 MV. Med Phys. 1991;18(4):724-726. doi: 10.1118/1.596695
- Podgorsak EB. Radiation Oncology Physics: A Handbook for Teachers and Students. Vienna: International Atomic Energy Agency (IAEA); 2005.
- Akhtaruzzaman M, Kukolowicz P. The dependence of inhomogeneity correction factors on photon beam quality index performed with the anisotropic analytical algorithm. Pol J Med Phys Eng. 2020;26(3):127-134. doi: 10.2478/pjmpe-2020-0016
- Wong JW, Purdy JA, Harms WB, et al. Role of inhomogeneity corrections in three-dimensional photon treatment planning. Int J Radiat Oncol Biol Phys. 1991;21(1):59-69. doi: 10.1016/0360-3016(91)90132-6.
- Murphy R, Hill T, Rapley P, et al. The influence of the inhomogeneity correction factor on dose distributions in lung cancer radiation therapy treatment plans: A retrospective study. Int J Radiat Oncol Biol Phys. 2012;84(3):S600. doi: 10.1016/j.ijrobp.2012.07.1498.
- Mah K, Van Dyk J. On the impact of tissue inhomogeneity corrections in clinical thoracic radiation therapy. Int J Radiat Oncol Biol Phys. 1991;21(5):1257-1267. doi: 10.1016/0360-3016(91)90232-7.
- Han Y, Park W, Huh SJ. The impact of tissue inhomogeneity corrections in the treatment of prostate cancer with intensity-modulated radiation therapy. Korean J Med Phys. 2004;15(3):149-155.
- Ding GX, Duggan DM, Lu B, et al. Impact of inhomogeneity corrections on dose coverage in the treatment of lung cancer using stereotactic body radiation therapy. Med Phys. 2007;34(7):2985-2994. doi: 10.1118/1.2745923