Cognition damage due to disruption of cyclic adenosine monophosphate-related signaling pathway in melatonin receptor 2 knockout mice
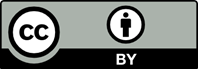
Alzheimer’s disease (AD) was characterized by the presence of neurofibrillary tangles and senile plaques. Although melatonin plays an important role in AD, its mechanism is still unknown. In this study, we found obvious cognition damage in melatonin receptor 2 knockout mice (MT2KO) and double knockout mice (DKO), but not in melatonin receptor 1 knockout mice (MT1KO). To explore the mechanism in-depth, we attempted to determine the levels of metabolites and amyloid-β peptide (Aβ). A high level of Cho/tCr (choline/total creatine) was detected in MT2KO and MT1KO by nuclear magnetic resonance (NMR), while a high level of myo-inositol/total creatine (mI/tCr) was only detected in MT1KO. A higher ratio of Aβ42/40 was found in MT2KO, but not in MT1KO and DKO. We also found an abnormal increase of plaque detected by the A3981 antibody in MT2KO and DKO. Furthermore, a huge decrease of postsynapses was confirmed in MT2KO and DKO, but not MT1KO, accompanied by a low level of phosphorylated cyclic adenosine monophosphate (cAMP) response element-binding protein (CREB) at the site of serine 133 and a low activity of protein kinase A. Finally, the cAMP, cyclic guanosine monophosphate, and cAMP-regulated guanine nucleotide exchange factor (EPAC) were detected. We observed a significant decrease of cAMP and EPAC2 in MT2KO, but not MT1KO. Thus, we identified that cAMP-related signaling pathway was disturbed in MT2KO and was critical for normal cognitive function.
- Dubocovich ML, 2007, Melatonin receptors: Role on sleep and circadian rhythm regulation. Sleep Med, 8: 34–42. https://doi.org/10.1016/j.sleep.2007.10.007
- Masana MI, Dubocovich ML, 2001, Melatonin receptor signaling: Finding the path through the dark. Sci STKE, 2001: pe39. https://doi.org/10.1126/stke.2001.107.pe39
- Brydon L, Roka F, Petit L, et al., 1999, Dual signaling of human Mel1a melatonin receptors via G(i2), G(i3), and G(q/11) proteins. Mol Endocrinol, 13: 2025–2038. https://doi.org/10.1210/mend.13.12.0390
- Huete-Toral F, Crooke A, Martinez-Aguila A, et al., 2015, Melatonin receptors trigger cAMP production and inhibit chloride movements in nonpigmented ciliary epithelial cells. J Pharmacol Exp Ther, 352: 119–128. https://doi.org/10.1124/jpet.114.218263
- Liu Y, Ni C, Li Z, et al., 2017, Prophylactic melatonin attenuates isoflurane-induced cognitive impairment in aged rats through hippocampal melatonin receptor 2-cAMP response element binding signalling. Basic Clin Pharmacol Toxicol, 120: 219–226. https://doi.org/10.1111/bcpt.12652
- Palmer AM, Burns MA, 1994, Selective increase in lipid peroxidation in the inferior temporal cortex in Alzheimer’s disease. Brain Res, 645: 338–342. https://doi.org/10.1016/0006-8993(94)91670-5
- Roy J, Tsui KC, Ng J, et al., 2021, Regulation of melatonin and neurotransmission in Alzheimer’s disease. Int J Mol Sci, 22: 6841. https://doi.org/10.3390/ijms22136841
- Gagnon K, Godbout R, 2018, Melatonin and comorbidities in children with autism spectrum disorder. Curr Dev Disord Rep, 5: 197–206. https://doi.org/10.1007/s40474-018-0147-0
- Savaskan E, Jockers R, Ayoub M, et al., 2007, The MT2 melatonin receptor subtype is present in human retina and decreases in Alzheimer’s disease. Curr Alzheimer Res, 4: 47–51. https://doi.org/10.2174/156720507779939823
- Congdon EE, Sigurdsson EM, 2018, Tau-targeting therapies for Alzheimer disease. Nat Rev Neurol, 14: 399–415. https://doi.org/10.1038/s41582-018-0013-z
- Villemagne VL, Dore V, Burnham SC, et al., 2018, Imaging tau and amyloid-β proteinopathies in Alzheimer disease and other conditions. Nat Rev Neurol, 14: 225–236. https://doi.org/10.1038/nrneurol.2018.9
- Liu CH, Chang HM, Yang YS, et al., 2020, Melatonin promotes nerve regeneration following end-to-side neurorrhaphy by accelerating cytoskeletal remodeling via the melatonin receptor-dependent pathway. Neuroscience, 429: 282–292. https://doi.org/10.1016/j.neuroscience.2019.09.009
- Liu D, Wei N, Man HY, et al., 2015, The MT2 receptor stimulates axonogenesis and enhances synaptic transmission by activating Akt signaling. Cell Death Differ, 22: 583–596. https://doi.org/10.1038/cdd.2014.195
- Deng YQ, Xu GG, Duan P, et al., 2005, Effects of melatonin on wortmannin-induced tau hyperphosphorylation. Acta Pharmacol Sin, 26: 519–526. https://doi.org/10.1111/j.1745-7254.2005.00102.x
- Li XC, Wang ZF, Zhang JX, et al., 2005, Effect of melatonin on calyculin A-induced tau hyperphosphorylation. Eur J Pharmacol, 510: 25–30. https://doi.org/10.1016/j.ejphar.2005.01.023
- Li SP, Deng YQ, Wang XC, et al., 2004, Melatonin protects SH-SY5Y neuroblastoma cells from calyculin A-induced neurofilament impairment and neurotoxicity. J Pineal Res, 36: 186–191. https://doi.org/10.1111/j.1600-079x.2004.00116.x
- Wang YP, Li XT, Liu SJ, et al., 2004, Melatonin ameliorated okadaic-acid induced Alzheimer-like lesions. Acta Pharmacol Sin, 25: 276–280.
- Li Y, Zhang J, Wan J, et al., 2020, Melatonin regulates Aβ production/clearance balance and Aβ neurotoxicity: A potential therapeutic molecule for Alzheimer’s disease. Biomed Pharmacother, 132: 110887. https://doi.org/10.1016/j.biopha.2020.110887
- Panmanee J, Nopparat C, Chavanich N, et al., 2015, Melatonin regulates the transcription of βAPP-cleaving secretases mediated through melatonin receptors in human neuroblastoma SH-SY5Y cells. J Pineal Res, 59: 308–320. https://doi.org/10.1111/jpi.12260
- Lahiri DK, 1999, Melatonin affects the metabolism of the beta-amyloid precursor protein in different cell types. J Pineal Res, 26: 137–146. https://doi.org/10.1111/j.1600-079x.1999.tb00575.x
- Song W, Lahiri DK, 1997, Melatonin alters the metabolism of the beta-amyloid precursor protein in the neuroendocrine cell line PC12. J Mol Neurosci, 9: 75–92. https://doi.org/10.1007/BF02736852
- Skribanek Z, Balaspiri L, Mak M, 2001, Interaction between synthetic amyloid-beta-peptide (1-40) and its aggregation inhibitors studied by electrospray ionization mass spectrometry. J Mass Spectrom, 36: 1226–1229. https://doi.org/10.1002/jms.243
- Zhang YC, Wang ZF, Wang Q, et al., 2004, Melatonin attenuates beta-amyloid-induced inhibition of neurofilament expression. Acta Pharmacol Sin, 25: 447–451.
- Ono K, Hasegawa K, Yoshiike Y, et al., 2002, Nordihydroguaiaretic acid potently breaks down pre-formed Alzheimer’s beta-amyloid fibrils in vitro. J Neurochem, 81: 434–440. https://doi.org/10.1046/j.1471-4159.2002.00904.x
- Rudnitskaya EA, Maksimova KY, Muraleva NA, et al., 2015, Beneficial effects of melatonin in a rat model of sporadic Alzheimer’s disease. Biogerontology, 16: 303–316. https://doi.org/10.1007/s10522-014-9547-7
- Lopez N, Tormo C, De Blas I, et al., 2013, Oxidative stress in Alzheimer’s disease and mild cognitive impairment with high sensitivity and specificity. J Alzheimers Dis, 33: 823–829. https://doi.org/10.3233/jad-2012-121528
- Wang X, Wang LP, Tang H, et al., 2014, Acetyl-L-carnitine rescues scopolamine-induced memory deficits by restoring insulin-like growth factor II via decreasing p53 oxidation. Neuropharmacology, 76: 80–87. https://doi.org/10.1016/j.neuropharm.2013.08.022
- Liu J, Ames BN, 2005, Reducing mitochondrial decay with mitochondrial nutrients to delay and treat cognitive dysfunction, Alzheimer’s disease, and Parkinson’s disease. Nutr Neurosci, 8: 67–89. https://doi.org/10.1080/10284150500047161
- Reddy PH, 2006, Mitochondrial oxidative damage in aging and Alzheimer’s disease: Implications for mitochondrially targeted antioxidant therapeutics. J Biomed Biotechnol, 2006: 31372. https://doi.org/10.1155/JBB/2006/31372
- Pena-Bautista C, Baquero M, Vento M, et al., 2019, Free radicals in Alzheimer’s disease: Lipid peroxidation biomarkers. Clin Chim Acta, 491: 85–90. https://doi.org/10.1016/j.cca.2019.01.021
- Savaskan E, Ayoub MA, Ravid R, et al., 2005, Reduced hippocampal MT2 melatonin receptor expression in Alzheimer’s disease. J Pineal Res, 38: 10–16. https://doi.org/10.1111/j.1600-079X.2004.00169.x
- Savaskan E, Olivieri G, Meier F, et al., 2002, Increased melatonin 1a-receptor immunoreactivity in the hippocampus of Alzheimer’s disease patients. J Pineal Res, 32: 59–62. https://doi.org/10.1034/j.1600-079x.2002.00841.x
- Block W, Jessen F, Traber F, et al., 2002, Regional N-acetylaspartate reduction in the hippocampus detected with fast proton magnetic resonance spectroscopic imaging in patients with Alzheimer disease. Arch Neurol, 59: 828–834. https://doi.org/10.1001/archneur.59.5.828
- Chang L, Munsaka SM, Kraft-Terry S, et al., 2013, Magnetic resonance spectroscopy to assess neuroinflammation and neuropathic pain. J Neuroimmune Pharmacol, 8: 576–593. https://doi.org/10.1007/s11481-013-9460-x
- Woolfrey KM, Srivastava DP, Photowala H, et al., 2009, Epac2 induces synapse remodeling and depression and its disease-associated forms alter spines. Nat Neurosci, 12: 1275–1284. https://doi.org/10.1038/nn.2386
- Jones KA, Sumiya M, Woolfrey KM, et al., 2019, Loss of EPAC2 alters dendritic spine morphology and inhibitory synapse density. Mol Cell Neurosci, 98: 19–31. https://doi.org/10.1016/j.mcn.2019.05.001