Lipid droplets in neurodegenerative disorders: Molecular insights and therapeutic intervention
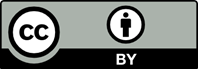
Lipid droplets (LDs) are dynamic lipid storage organelles, derived from phospholipid monolayer membrane of the endoplasmic reticulum. LDs are composed of neutral lipids, mainly including triglycerides, sterol esters, and other non-polar lipids like cholesterol. The functions of LDs differ from different cell types in the central nervous system. The major role of LDs is to maintain lipid homeostasis, provide energy supply, and play a protective role in response to intracellular oxidative stress in the brain. Oxidative stress can lead to lipid dyshomeostasis, particularly the accumulation of LDs, followed by abnormal myelination, neuroinflammation, and cognitive decline. Neuroinflammation, aging, and age-related neurodegenerative disorders (NDDs) are characterized by the excessive accumulation of LDs in the brain. Although the LDs accumulation in the brain of the NDDs mouse model has been reported as early as a century ago, the role of LDs in the brain remains largely unknown. It is still unclear regarding the involvement of LDs accumulation in the pathogenesis of NDDs. In this review, we provide an overview of LDs biogenesis, the characteristics and functions of LDs from a cell-type perspective, and the pathological roles of LDs in NDDs on recent advances. In addition, we discuss the emerging LDs-based therapeutic strategies for NDDs, which may provide promising clues to develop novel therapeutic approaches for NDDs.
- Ralhan I, Chang CL, Lippincott-Schwartz J, Ioannou MS. Lipid droplets in the nervous system. J Cell Biol. 2021;220(7):e202102136. doi: 10.1083/jcb.202102136
- Smolic T, Zorec R, Vardjan N. Pathophysiology of lipid droplets in neuroglia. Antioxidants (Basel). 2021;11(1):22. doi: 10.3390/antiox11010022
- Bailey AP, Koster G, Guillermier C, et al. Antioxidant role for lipid droplets in a stem cell niche of Drosophila. Cell. 2015;163(2):340-353. doi: 10.1016/j.cell.2015.09.020
- Liu L, Zhang K, Sandoval H, et al. Glial lipid droplets and ROS induced by mitochondrial defects promote neurodegeneration. Cell. 2015;160(1-2):177-190. doi: 10.1016/j.cell.2014.12.019
- Zhang Y, Chen Y, Zhuang C, et al. Lipid droplets in the nervous system: Involvement in cell metabolic homeostasis. Neural Regen Res. 2025;20(3):740-750. doi: 10.4103/NRR.NRR-D-23-01401
- Garcia K, Alves A, Ribeiro-Rodrigues TM, Reis F, Viana S. Analysis of fluorescent-stained lipid droplets with 3D reconstruction for hepatic steatosis assessment. J Vis Exp. 2023;19(6):e65206. doi: 10.3791/65206
- Hsia JZ, Liu D, Haynes L, Cruz-Cosme R, Tang Q. Lipid droplets: Formation, degradation, and their role in cellular responses to flavivirus infections. Microorganisms. 2024;12(4):647. doi: 10.3390/microorganisms12040647
- Thiam AR, Farese RV Jr., Walther TC. The biophysics and cell biology of lipid droplets. Nat Rev Mol Cell Biol. 2013;14(12):775-786. doi: 10.1038/nrm3699
- Zhang Z, Yu Z, Liang D, et al. Roles of lipid droplets and related proteins in metabolic diseases. Lipids Health Dis. 2024;23(1):218. doi: 10.1186/s12944-024-02212-y
- Thiam AR, Ikonen E. Lipid droplet nucleation. Trends Cell Biol. 2021;31(2):108-118. doi: 10.1016/j.tcb.2020.11.006
- Santinho A, Salo VT, Chorlay A, et al. Membrane curvature catalyzes lipid droplet assembly. Curr Biol. 2020;30(13): 2481-2494.e6. doi: 10.1016/j.cub.2020.04.066
- Han S, Binns DD, Chang YF, Goodman JM. Dissecting seipin function: The localized accumulation of phosphatidic acid at ER/LD junctions in the absence of seipin is suppressed by Sei1p(DeltaNterm) only in combination with Ldb16p. BMC Cell Biol. 2015;16:29. doi: 10.1186/s12860-015-0075-3
- Xu H, Li M, Ma D, Gao J, Tao J, Meng J. Identification of key genes for triacylglycerol biosynthesis and storage in herbaceous peony (Paeonia lactifolra Pall.) seeds based on full-length transcriptome. BMC Genomics. 2024;25(1):601. doi: 10.1186/s12864-024-10513-w
- Madsen S, Delgado AC, Cadilhac C, et al. A fluorescent perilipin 2 knock-in mouse model reveals a high abundance of lipid droplets in the developing and adult brain. Nat Commun. 2024;15(1):5489. doi: 10.1038/s41467-024-49449-w
- Ramosaj M, Madsen S, Maillard V, et al. Lipid droplet availability affects neural stem/progenitor cell metabolism and proliferation. Nat Commun. 2021;12(1):7362. doi: 10.1038/s41467-021-27365-7
- Liu L, MacKenzie KR, Putluri N, Maletic-Savatic M, Bellen HJ. The glia-neuron lactate shuttle and elevated ROS promote lipid synthesis in neurons and lipid droplet accumulation in glia via APOE/D. Cell Metab. 2017;26(5):719-737.e6. doi: 10.1016/j.cmet.2017.08.024
- Lee JA, Hall B, Allsop J, Alqarni R, Allen SP. Lipid metabolism in astrocytic structure and function. Semin Cell Dev Biol. 2021;112:123-136. doi: 10.1016/j.semcdb.2020.07.017
- Mauch DH, Nagler K, Schumacher S, et al. CNS synaptogenesis promoted by glia-derived cholesterol. Science. 2001;294(5545):1354-1357. doi: 10.1126/science.294.5545.1354
- Lipke K, Kubis-Kubiak A, Piwowar A. Molecular mechanism of lipotoxicity as an interesting aspect in the development of pathological states-current view of knowledge. Cells. 2022;11(5):844. doi: 10.3390/cells11050844
- Prinz M, Jung S, Priller J. Microglia biology: One century of evolving concepts. Cell. 2019;179(2):292-311. doi: 10.1016/j.cell.2019.08.053
- Nugent AA, Lin K, van Lengerich B, et al. TREM2 regulates microglial cholesterol metabolism upon chronic phagocytic challenge. Neuron. 2020;105(5):837-854.e9. doi: 10.1016/j.neuron.2019.12.007
- Kunjathoor VV, Tseng AA, Medeiros LA, Khan T, Moore KJ. beta-Amyloid promotes accumulation of lipid peroxides by inhibiting CD36-mediated clearance of oxidized lipoproteins. J Neuroinflammation. 2004;1(1):23. doi: 10.1186/1742-2094-1-23
- Cantuti-Castelvetri L, Fitzner D, Bosch-Queralt M, et al. Defective cholesterol clearance limits remyelination in the aged central nervous system. Science. 2018;359(6376): 684-688. doi: 10.1126/science.aan4183
- Krasemann S, Madore C, Cialic R, et al. The TREM2- APOE pathway drives the transcriptional phenotype of dysfunctional microglia in neurodegenerative diseases. Immunity. 2017;47(3):566-581.e9. doi: 10.1016/j.immuni.2017.08.008
- Marschallinger J, Iram T, Zardeneta M, et al. Lipid-droplet-accumulating microglia represent a dysfunctional and proinflammatory state in the aging brain. Nat Neurosci. 2020;23(2):194-208. doi: 10.1038/s41593-019-0566-1
- Haney MS, Palovics R, Munson CN, et al. APOE4/4 is linked to damaging lipid droplets in Alzheimer’s disease microglia. Nature. 2024;628(8006):154-161. doi: 10.1038/s41586-024-07185-7
- Litvinchuk A, Suh JH, Guo JL, et al. Amelioration of Tau and ApoE4-linked glial lipid accumulation and neurodegeneration with an LXR agonist. Neuron. 2024;112(3):384-403.e388. doi: 10.1016/j.neuron.2023.10.023
- Huang H, Xiang R, Yan R. Linking APOE4/4 genotype to microglial lipid droplets and neurotoxicity in Alzheimer’s disease. Transl Neurodegener. 2024;13(1):38. doi: 10.1186/s40035-024-00433-w
- Garcia Corrales AV, Haidar M, Bogie JFJ, Hendriks JJA. Fatty acid synthesis in glial cells of the CNS. Int J Mol Sci. 2021;22(15):8159. doi: 10.3390/ijms22158159
- Vicente-Gutierrez C, Jimenez-Blasco D, Quintana-Cabrera R. Intertwined ROS and metabolic signaling at the neuron-astrocyte interface. Neurochem Res. 2021;46(1):23-33. doi: 10.1007/s11064-020-02965-9
- Bak LK, Walls AB, Schousboe A, Waagepetersen HS. Astrocytic glycogen metabolism in the healthy and diseased brain. J Biol Chem. 2018;293(19):7108-7116. doi: 10.1074/jbc.R117.803239
- Smolic T, Tavcar P, Horvat A, et al. Astrocytes in stress accumulate lipid droplets. Glia. 2021;69(6):1540-1562. doi: 10.1002/glia.23978
- Pacheco P, Bozza FA, Gomes RN, et al. Lipopolysaccharide-induced leukocyte lipid body formation in vivo: Innate immunity elicited intracellular Loci involved in eicosanoid metabolism. J Immunol. 2002;169(11):6498-6506. doi: 10.4049/jimmunol.169.11.6498
- Khatchadourian A, Bourque SD, Richard VR, Titorenko VI, Maysinger D. Dynamics and regulation of lipid droplet formation in lipopolysaccharide (LPS)-stimulated microglia. Biochim Biophys Acta. 2012;1821(4):607-617. doi: 10.1016/j.bbalip.2012.01.007
- Buchanan MM, Hutchinson M, Watkins LR, Yin H. Toll-like receptor 4 in CNS pathologies. J Neurochem. 2010;114(1): 13-27. doi: 10.1111/j.1471-4159.2010.06736.x
- Deczkowska A, Keren-Shaul H, Weiner A, et al. Disease-associated microglia: A universal immune sensor of neurodegeneration. Cell. 2018;173(5):1073-1081. doi: 10.1016/j.cell.2018.05.003
- Simpson DSA, Oliver PL. ROS generation in microglia: Understanding oxidative stress and inflammation in neurodegenerative disease. Antioxidants (Basel). 2020;9(8):743. doi: 10.3390/antiox9080743
- Chausse B, Kakimoto PA, Kann O. Microglia and lipids: How metabolism controls brain innate immunity. Semin Cell Dev Biol. 2021;112:137-144. doi: 10.1016/j.semcdb.2020.08.001
- Gotz M, Huttner WB. The cell biology of neurogenesis. Nat Rev Mol Cell Biol. 2005;6(10):777-788. doi: 10.1038/nrm1739
- Bairos JA, Njoku U, Zafar M, et al. Sterol O-acyltransferase (SOAT/ACAT) activity is required to form cholesterol crystals in hepatocyte lipid droplets. Biochim Biophys Acta Mol Cell Biol Lipids. 2024;1869(6):159512. doi: 10.1016/j.bbalip.2024.159512
- Chang TY, Chang CC, Lin S, et al. Roles of acyl-coenzyme A: cholesterol acyltransferase-1 and -2. Curr Opin Lipidol. 2001;12(3):289-296. doi: 10.1097/00041433-200106000-00008
- Melton EM, Li H, Benson J, et al. Myeloid Acat1/Soat1 KO attenuates pro-inflammatory responses in macrophages and protects against atherosclerosis in a model of advanced lesions. J Biol Chem. 2019;294(43):15836-15849. doi: 10.1074/jbc.RA119.010564
- Bryleva EY, Rogers MA, Chang CC, et al. ACAT1 gene ablation increases 24(S)-hydroxycholesterol content in the brain and ameliorates amyloid pathology in mice with AD. Proc Natl Acad Sci U S A. 2010;107(7):3081-3086. doi: 10.1073/pnas.0913828107
- Sun G, Fu C, Shen C, Shi Y. Histone deacetylases in neural stem cells and induced pluripotent stem cells. J Biomed Biotechnol. 2011;2011:835968. doi: 10.1155/2011/835968
- Stephenson RA, Thomalla JM, Chen L, et al. Sequestration to lipid droplets promotes histone availability by preventing turnover of excess histones. Development. 2021;148(15):dev199381. doi: 10.1242/dev.199381
- Li Z, Thiel K, Thul PJ, et al. Lipid droplets control the maternal histone supply of Drosophila embryos. Curr Biol. 2012;22(22):2104-2113. doi: 10.1016/j.cub.2012.09.018
- Shimabukuro MK, Langhi LGP, Cordeiro I, et al. Lipid-laden cells differentially distributed in the aging brain are functionally active and correspond to distinct phenotypes. Sci Rep. 2016;6:23795. doi: 10.1038/srep23795
- Yousef H, Czupalla CJ, Lee D, et al. Aged blood impairs hippocampal neural precursor activity and activates microglia via brain endothelial cell VCAM1. Nat Med. 2019;25(6):988-1000. doi: 10.1038/s41591-019-0440-4
- Scheltens P, De Strooper B, Kivipelto M, et al. Alzheimer’s disease. Lancet. 2021;397(10284):1577-1590. doi: 10.1016/S0140-6736(20)32205-4
- Swerdlow RH. The neurodegenerative mitochondriopathies. J Alzheimers Dis. 2009;17(4):737-751. doi: 10.3233/JAD-2009-1095
- Alzheimer A, Stelzmann RA, Schnitzlein HN, Murtagh FR. An English translation of Alzheimer’s 1907 paper, “Uber eine eigenartige Erkankung der Hirnrinde”. Clin Anat. 1995;8(6):429-431. doi: 10.1002/ca.980080612
- Hu X, Ma YN, Xia Y. Association between abnormal lipid metabolism and Alzheimer’s disease: New research has revealed significant findings on the APOE4 genotype in microglia. Biosci Trends. 2024;18(2):195-197. doi: 10.5582/bst.2024.01092
- Roca-Agujetas V, Barbero-Camps E, de Dios C, et al. Cholesterol alters mitophagy by impairing optineurin recruitment and lysosomal clearance in Alzheimer’s disease. Mol Neurodegener. 2021;16(1):15. doi: 10.1186/s13024-021-00435-6
- van der Kant R, Langness VF, Herrera CM, et al. Cholesterol metabolism is a druggable axis that independently regulates tau and amyloid-beta in iPSC-derived Alzheimer’s disease neurons. Cell Stem Cell. 2019;24(3):363-375.e369. doi: 10.1016/j.stem.2018.12.013
- Malkki H. Alzheimer disease: Effects of the APOE epsilon4 allele on brain development. Nat Rev Neurol. 2014;10(1):4. doi: 10.1038/nrneurol.2013.258
- de-Almada BV, de-Almeida LD, Camporez D, et al. Protective effect of the APOE-e3 allele in Alzheimer’s disease. Braz J Med Biol Res. 2012;45(1):8-12. doi: 10.1590/s0100-879x2011007500151
- Velez JI, Lopera F, Sepulveda-Falla D, et al. APOE*E2 allele delays age of onset in PSEN1 E280A Alzheimer’s disease. Mol Psychiatry. 2016;21(7):916-924.doi: 10.1038/mp.2015.177
- Corder EH, Saunders AM, Strittmatter WJ, et al. Gene dose of apolipoprotein E type 4 allele and the risk of Alzheimer’s disease in late onset families. Science. 1993;261(5123):921-923. doi: 10.1126/science.8346443
- Husain MA, Laurent B, Plourde M. APOE and Alzheimer’s disease: From lipid transport to physiopathology and therapeutics. Front Neurosci. 2021;15:630502. doi: 10.3389/fnins.2021.630502
- Griseti E, Bello AA, Bieth E, et al. Molecular mechanisms of perilipin protein function in lipid droplet metabolism. FEBS Lett. 2024;598(10):1170-1198. doi: 10.1002/1873-3468.14792
- Yin C, Ackermann S, Ma Z, et al. ApoE attenuates unresolvable inflammation by complex formation with activated C1q. Nat Med. 2019;25(3):496-506. doi: 10.1038/s41591-018-0336-8
- Parhizkar S, Arzberger T, Brendel M, et al. Loss of TREM2 function increases amyloid seeding but reduces plaque-associated ApoE. Nat Neurosci. 2019;22(2):191-204. doi: 10.1038/s41593-018-0296-9
- Heneka MT. ApoE4 makes microglia trem(2)bling. Neuron. 2023;111(2):142-144. doi: 10.1016/j.neuron.2022.12.032
- Qin Q, Wang M, Yin Y, Tang Y. The specific mechanism of TREM2 regulation of synaptic clearance in Alzheimer’s disease. Front Immunol. 2022;13:845897. doi: 10.3389/fimmu.2022.845897
- Fanning S, Selkoe D, Dettmer U. Parkinson’s disease: Proteinopathy or lipidopathy? NPJ Parkinsons Dis. 2020;6:3. doi: 10.1038/s41531-019-0103-7
- Fanning S, Selkoe D, Dettmer U. Vesicle trafficking and lipid metabolism in synucleinopathy. Acta Neuropathol. 2021;141(4):491-510. doi: 10.1007/s00401-020-02177-z
- Fanning S, Haque A, Imberdis T, et al. Lipidomic analysis of alpha-synuclein neurotoxicity identifies stearoyl CoA desaturase as a target for parkinson treatment. Mol Cell. 2019;73(5):1001-1014.e1008. doi: 10.1016/j.molcel.2018.11.028
- Cole NB, Murphy DD, Grider T, et al. Lipid droplet binding and oligomerization properties of the Parkinson’s disease protein alpha-synuclein. J Biol Chem. 2002;277(8):6344-6352. doi: 10.1074/jbc.M108414200
- Vincent BM, Tardiff DF, Piotrowski JS, et al. Inhibiting stearoyl-CoA desaturase ameliorates alpha-synuclein cytotoxicity. Cell Rep. 2018;25(10):2742-2754.e2731. doi: 10.1016/j.celrep.2018.11.028
- Ates G, Goldberg J, Currais A, Maher P. CMS121, a fatty acid synthase inhibitor, protects against excess lipid peroxidation and inflammation and alleviates cognitive loss in a transgenic mouse model of Alzheimer’s disease. Redox Biol. 2020;36:101648. doi: 10.1016/j.redox.2020.101648
- Li Y, Yang X, Peng L, et al. Role of seipin in human diseases and experimental animal models. Biomolecules. 2022;12(6):840. doi: 10.3390/biom12060840
- Bernier F, Kuhara T, Xiao J. Probiotic bifidobacterium breve MCC1274 protects against oxidative stress and neuronal lipid droplet formation via PLIN4 gene regulation. Microorganisms. 2023;11(3):791. doi: 10.3390/microorganisms11030791
- Han X, Zhao S, Song H, et al. Kaempferol alleviates LD-mitochondrial damage by promoting autophagy: Implications in Parkinson’s disease. Redox Biol. 2021;41:101911. doi: 10.1016/j.redox.2021.101911
- Murphy SR, Chang CC, Dogbevia G, et al. Acat1 knockdown gene therapy decreases amyloid-beta in a mouse model of Alzheimer’s disease. Mol Ther. 2013;21(8):1497-1506. doi: 10.1038/mt.2013.118
- Hutter-Paier B, Huttunen HJ, Puglielli L, et al. The ACAT inhibitor CP-113,818 markedly reduces amyloid pathology in a mouse model of Alzheimer’s disease. Neuron. 2004;44(2):227-238. doi: 10.1016/j.neuron.2004.08.043
- Huttunen HJ, Havas D, Peach C, et al. The acyl-coenzyme A: cholesterol acyltransferase inhibitor CI-1011 reverses diffuse brain amyloid pathology in aged amyloid precursor protein transgenic mice. J Neuropathol Exp Neurol. 2010;69(8): 777-788. doi: 10.1097/NEN.0b013e3181e77ed9