Ferroptosis in neonatal hypoxic-ischemic brain injury and implications for therapeutic development
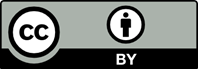
The morbidity and mortality associated with neonatal hypoxic-ischemic brain injury (HIBI) and the related clinical syndrome, hypoxic-ischemic encephalopathy (HIE), remain substantial. Consequently, treatment alternatives to therapeutic hypothermia, the current standard of care, are urgently needed. Therefore, unique aspects of the complex mechanistic underpinnings of neonatal HIBI and HIE must be studied. This review focuses on ferroptosis, a unique form of cell death, which was first described in 2012 and is characterized by iron-dependent lipid peroxidation, leading to lethal reactive oxygen species buildup. The role of ferroptosis in neonatal HIBI has been indirectly supported by decades of research and directly demonstrated using in vivo and in vitro models in recent years. Molecular targets, including nuclear factor erythroid 2-related factor 2, cystine/glutamate antiporter (system xc–), and glutathione peroxidase-4, have been identified as key mediators of ferroptosis in neonatal HIBI. In preliminary experiments, agents modulating the activity of these and other targets effectively suppress ferroptosis and attenuate the structural and functional consequences of neonatal HIBI. While considerable work is still required to establish the underlying mechanisms and therapeutic importance of these effects, the foundational studies show that antiferroptotic agents may reduce the lasting burden of neonatal HIBI and HIE.

- Kurinczuk JJ, White-Koning M, Badawi N. Epidemiology of neonatal encephalopathy and hypoxic-ischaemic encephalopathy. Early Hum Dev. 2010;86(6):329-338. doi: 10.1016/j.earlhumdev.2010.05.010
- American College of Obstetricians and Gynecologists’ Task Force on Neonatal Encephalopathy. Executive summary: Neonatal encephalopathy and neurologic outcome, second edition. Obstet Gynecol. 2014;123(4):896-901. doi: 10.1097/01.AOG.0000445580.65983.d2
- Hypoxic Ischemic Encephalopathy. National Institute of Neurological Disorders and Stroke. Available from: https:// www.ninds.nih.gov/health-information/disorders/hypoxic-ischemic-encephalopathy [Last accessed on 2024 Oct 10].
- Basfar W, Jabbour E. 153 Cost of hospitalization in infants with hypoxic ischemic encephalopathy treated with therapeutic hypothermia in a Quebec tertiary neonatal intensive care unit and validation of the Canadian neonatal network costing algorithm. Paediatr Child Health. 2021;26(S1):e105-e107. doi: 10.1093/pch/pxab061.121
- Eunson P. The long-term health, social, and financial burden of hypoxic-ischaemic encephalopathy. Dev Med Child Neurol. 2015;57(S3):48-50. doi: 10.1111/dmcn.12727
- Massaro AN, Murthy K, Zaniletti I, et al. Intercenter cost variation for perinatal hypoxic-ischemic encephalopathy in the era of therapeutic hypothermia. J Pediatr. 2016;173:76-83.e1. doi: 10.1016/j.jpeds.2016.02.033
- Lemyre B, Chau V. Hypothermia for newborns with hypoxic-ischemic encephalopathy. Paediatr Child Health. 2018;23(4):285-291. doi: 10.1093/pch/pxy028
- Ma H, Sinha B, Pandya RS, et al. Therapeutic hypothermia as a neuroprotective strategy in neonatal hypoxic-ischemic brain injury and traumatic brain injury. Curr Mol Med. 2012;12(10):1282-1296. doi: 10.2174/156652412803833517
- Natarajan G, Pappas A, Shankaran S. Outcomes in childhood following therapeutic hypothermia for neonatal hypoxic-ischemic encephalopathy (HIE). Semin Perinatol. 2016;40(8):549-555. doi: 10.1053/j.semperi.2016.09.007
- Groenendaal F, Shadid M, McGowan JE, Mishra OP, van Bel F. Effects of deferoxamine, a chelator of free iron, on NA(+), K(+)-ATPase activity of cortical brain cell membrane during early reperfusion after hypoxia-ischemia in newborn lambs. Pediatr Res. 2000;48(4):560-564. doi: 10.1203/00006450-200010000-00023
- Ferriero DM. Oxidant mechanisms in neonatal hypoxia-ischemia. Dev Neurosci. 2001;23(3):198-202. doi: 10.1159/000046143
- Li Y, Wang T, Sun P, et al. Farrerol alleviates hypoxic-ischemic encephalopathy by inhibiting ferroptosis in neonatal rats via the Nrf2 pathway. Physiol Res. 2023;72(4):511-520. doi: 10.33549/physiolres.935040
- Palmer C, Roberts RL, Bero C. Deferoxamine posttreatment reduces ischemic brain injury in neonatal rats. Stroke. 1994;25(5):1039-1045. doi: 10.1161/01.str.25.5.1039
- Zhu K, Zhu X, Sun S, et al. Inhibition of TLR4 prevents hippocampal hypoxic-ischemic injury by regulating ferroptosis in neonatal rats. Exp Neurol. 2021;345:113828. doi: 10.1016/j.expneurol.2021.113828
- Busl KM, Greer DM. Hypoxic-ischemic brain injury: Pathophysiology, neuropathology and mechanisms. NeuroRehabilitation. 2010;26(1):5-13. doi: 10.3233/NRE-2010-0531
- Johnston MV, Trescher WH, Ishida A, Nakajima W. Neurobiology of hypoxic-ischemic injury in the developing brain. Pediatr Res. 2001;49(6):735-741. doi: 10.1203/00006450-200106000-00003
- Li B, Concepcion K, Meng X, Zhang L. Brain-immune interactions in perinatal hypoxic-ischemic brain injury. Prog Neurobiol. 2017;159:50-68. doi: 10.1016/j.pneurobio.2017.10.006
- Ayata C. Monitoring anoxic depolarization at the bedside: A step closer to the 24th century. J Cereb Blood Flow Metab. 2018;38(7):1123-1124. doi: 10.1177/0271678X18774999
- Kaminogo M, Suyama K, Ichikura A, Onizuka M, Shibata S. Anoxic depolarization determines ischemic brain injury. Neurol Res. 1998;20(4):343-348. doi: 10.1080/01616412.1998.11740529
- Lipton SA, Rosenberg PA. Excitatory amino acids as a final common pathway for neurologic disorders. N Engl J Med. 1994;330(9):613-622. doi: 10.1056/NEJM199403033300907
- Tremblay E, Roisin MP, Represa A, Charriaut-Marlangue C, Ben-Ari Y. Transient increased density of NMDA binding sites in the developing rat hippocampus. Brain Res. 1988;461(2):393-396. doi: 10.1016/0006-8993(88)90275-2
- Represa A, Tremblay E, Ben-Ari Y. Transient increase of NMDA-binding sites in human hippocampus during development. Neurosci Lett. 1989;99(1-2):61-66. doi: 10.1016/0304-3940(89)90265-6
- Liu F, McCullough LD. Inflammatory responses in hypoxic ischemic encephalopathy. Acta Pharmacol Sin. 2013;34(9):1121-1130. doi: 10.1038/aps.2013.89
- Lacerte M, Hays Shapshak A, Mesfin FB. Hypoxic Brain Injury. StatPearls Publishing; 2023. Available from: https:// www.ncbi.nlm.nih.gov/books/NBK537310 [Last accessed on 2024 Oct 10].
- Elmore S. Apoptosis: A review of programmed cell death. Toxicol Pathol. 2007;35(4):495-516. doi: 10.1080/01926230701320337
- Lipton P. Ischemic cell death in brain neurons. Physiol Rev. 1999;79(4):1431-1568. doi: 10.1152/physrev.1999.79.4.1431
- Tang S, Gao P, Chen H, Zhou X, Ou Y, He Y. The role of iron, its metabolism and ferroptosis in traumatic brain injury. Front Cell Neurosci. 2020;14:590789. doi: 10.3389/fncel.2020.590789
- Khalid N, Azimpouran M. Necrosis. StatPearls Publishing; 2023. Available from: https://www.ncbi.nlm.nih.gov/books/ NBK557627 [Last accessed on 2024 Oct 10].
- Wu L, Chang E, Zhao H, Ma D. Regulated cell death in hypoxic-ischaemic encephalopathy: Recent development and mechanistic overview. Cell Death Discov. 2024;10(1):277-216. doi: 10.1038/s41420-024-02014-2
- Yang Z, Klionsky DJ. An overview of the molecular mechanism of autophagy. Curr Top Microbiol Immunol. 2009;335:1-32. doi: 10.1007/978-3-642-00302-8_1
- Dixon SJ, Lemberg KM, Lamprecht MR, et al. Ferroptosis: An iron-dependent form of nonapoptotic cell death. Cell. 2012;149(5):1060-1072. doi: 10.1016/j.cell.2012.03.042
- Johnson DS, Chen YH. Ras family of small GTPases in immunity and inflammation. Curr Opin Pharmacol. 2012;12(4):458-463. doi: 10.1016/j.coph.2012.02.003
- Dolma S, Lessnick SL, Hahn WC, Stockwell BR. Identification of genotype-selective antitumor agents using synthetic lethal chemical screening in engineered human tumor cells. Cancer Cell. 2003;3(3):285-296. doi: 10.1016/s1535-6108(03)00050-3
- Yagoda N, von Rechenberg M, Zaganjor E, et al. RAS-RAF-MEK-dependent oxidative cell death involving voltage-dependent anion channels. Nature. 2007;447(7146):864-868. doi: 10.1038/nature05859
- Yang WS, Stockwell BR. Synthetic lethal screening identifies compounds activating iron-dependent, nonapoptotic cell death in oncogenic-RAS-harboring cancer cells. Chem Biol. 2008;15(3):234-245. doi: 10.1016/j.chembiol.2008.02.010
- Deng L, He S, Guo N, Tian W, Zhang W, Luo L. Molecular mechanisms of ferroptosis and relevance to inflammation. Inflamm Res. 2023;72(2):281-299. doi: 10.1007/s00011-022-01672-1
- Liu Y, Gu W. p53 in ferroptosis regulation: The new weapon for the old guardian. Cell Death Differ. 2022;29(5):895-910. doi: 10.1038/s41418-022-00943-y
- Zheng H, Jiang L, Tsuduki T, Conrad M, Toyokuni S. Embryonal erythropoiesis and aging exploit ferroptosis. Redox Biol. 2021;48:102175. doi: 10.1016/j.redox.2021.102175
- Cai W, Liu L, Shi X, et al. Alox15/15-HpETE aggravates myocardial ischemia-reperfusion injury by promoting cardiomyocyte ferroptosis. Circulation. 2023;147(19):1444-1460. doi: 10.1161/CIRCULATIONAHA.122.060257
- Chen L, Hambright WS, Na R, Ran Q. Ablation of the ferroptosis inhibitor glutathione peroxidase 4 in neurons results in rapid motor neuron degeneration and paralysis. J Biol Chem. 2015;290(47):28097-28106. doi: 10.1074/jbc.M115.680090
- Friedmann Angeli JP, Schneider M, Proneth B, et al. Inactivation of the ferroptosis regulator Gpx4 triggers acute renal failure in mice. Nat Cell Biol. 2014;16(12):1180-1191. doi: 10.1038/ncb3064
- Li Y, Feng D, Wang Z, et al. Ischemia-induced ACSL4 activation contributes to ferroptosis-mediated tissue injury in intestinal ischemia/reperfusion. Cell Death Differ. 2019;26(11):2284-2299. doi: 10.1038/s41418-019-0299-4
- Li C, Wu Z, Xue H, et al. Ferroptosis contributes to hypoxic-ischemic brain injury in neonatal rats: Role of the SIRT1/Nrf2/GPx4 signaling pathway. CNS Neurosci Ther. 2022;28(12):2268-2280. doi: 10.1111/cns.13973
- Wang ZW, Yang LJ, Ding YX, Chang YZ, Cui H. Insights into the role of iron in immature rat model of hypoxic-ischemic brain injury. Exp Ther Med. 2016;12(3):1723-1731. doi: 10.3892/etm.2016.3550
- Yang WS, SriRamaratnam R, Welsch ME, et al. Regulation of ferroptotic cancer cell death by GPX4. Cell. 2014;156(1- 2):317-331. doi: 10.1016/j.cell.2013.12.010
- Yang Y, Tang H, Zheng J, Yang K. The PER1/HIF-1alpha negative feedback loop promotes ferroptosis and inhibits tumor progression in oral squamous cell carcinoma. Transl Oncol. 2022;18:101360. doi: 10.1016/j.tranon.2022.101360
- Zhang M, Lin W, Tao X, et al. Ginsenoside Rb1 inhibits ferroptosis to ameliorate hypoxic-ischemic brain damage in neonatal rats. Int Immunopharmacol. 2023;121:110503. doi: 10.1016/j.intimp.2023.110503
- Fotiadis D, Kanai Y, Palacín M. The SLC3 and SLC7 families of amino acid transporters. Mol Aspects Med. 2013;34(2- 3):139-158. doi: 10.1016/j.mam.2012.10.007
- Sato H, Tamba M, Ishii T, Bannai S. Cloning and expression of a plasma membrane cystine/glutamate exchange transporter composed of two distinct proteins. J Biol Chem. 1999;274(17):11455-11458. doi: 10.1074/jbc.274.17.11455
- Bridges RJ, Natale NR, Patel SA. System xc-cystine/glutamate antiporter: An update on molecular pharmacology and roles within the CNS. Br J Pharmacol. 2011;165(1):20-34. doi: 10.1111/j.1476-5381.2011.01480.x
- Tan X, Zhang T, Ding X, et al. Iron overload facilitates neonatal hypoxic-ischemic brain damage via SLC7A11- mediated ferroptosis. J Neurosci Res. 2023;101(7):1107-1124. doi: 10.1002/jnr.25184
- Kuang F, Liu J, Tang D, Kang R. Oxidative damage and antioxidant defense in ferroptosis. Front Cell Dev Biol. 2020;8:586578. doi: 10.3389/fcell.2020.586578
- Yang WS, Stockwell BR. Ferroptosis: Death by lipid peroxidation. Trends Cell Biol. 2016;26(3):165-176. doi: 10.1016/j.tcb.2015.10.014
- GPX4 Glutathione Peroxidase 4 [Homo Sapiens (human)]. National Center for Biotechnology Information. Available from: https://www.ncbi.nlm.nih.gov/ gene/2879#:~:text=Glutathione%20peroxidase%20is%20 a%20moonlighting [Last accessed on 2024 Oct 10].
- Zhang Y, Swanda RV, Nie L, et al. mTORC1 couples cyst(e) ine availability with GPX4 protein synthesis and ferroptosis regulation. Nat Commun. 2021;12(1):1589. doi: 10.1038/s41467-021-21841-w
- Song X, Long D. Nrf2 and ferroptosis: A new research direction for neurodegenerative diseases. Front Neurosci. 2020;14:267. doi: 10.3389/fnins.2020.00267
- Zhao T, Yu Z, Zhou L, et al. Regulating Nrf2-GPx4 axis by bicyclol can prevent ferroptosis in carbon tetrachloride-induced acute liver injury in mice. Cell Death Discov. 2022;8(1):380. doi: 10.1038/s41420-022-01173-4
- Hsieh CH, Lin YJ, Chen WL, et al. HIF-1alpha triggers long-lasting glutamate excitotoxicity via system x(c)(-) in cerebral ischaemia-reperfusion. J Pathol. 2017;241(3):337-349. doi: 10.1002/path.4838
- Yuan S, Wei C, Liu G, et al. Sorafenib attenuates liver fibrosis by triggering hepatic stellate cell ferroptosis via HIF-1alpha/ SLC7A11 pathway. Cell Prolif. 2022;55(1):e13158. doi: 10.1111/cpr.13158
- Stoyanovsky DA, Tyurina YY, Shrivastava I, et al. Iron catalysis of lipid peroxidation in ferroptosis: Regulated enzymatic or random free radical reaction? Free Radic Biol Med. 2019;133:153-161. doi: 10.1016/j.freeradbiomed.2018.09.008
- Sun S, Shen J, Jiang J, Wang F, Min J. Targeting ferroptosis opens new avenues for the development of novel therapeutics. Signal Transduct Target Ther. 2023;8(1):372. doi: 10.1038/s41392-023-01606-1
- Doll S, Freitas FP, Shah R, et al. FSP1 is a glutathione-independent ferroptosis suppressor. Nature. 2019;575(7784):693-698. doi: 10.1038/s41586-019-1707-0
- Hu Q, Wei W, Wu D, et al. Blockade of GCH1/BH4 axis activates ferritinophagy to mitigate the resistance of colorectal cancer to erastin-induced ferroptosis. Front Cell Dev Biol. 2022;10:810327. doi: 10.3389/fcell.2022.810327
- Dixon SJ, Winter GE, Musavi LS, et al. Human haploid cell genetics reveals roles for lipid metabolism genes in nonapoptotic cell death. ACS Chem Biol. 2015;10(7):1604-1609. doi: 10.1021/acschembio.5b00245
- Doll S, Proneth B, Tyurina YY, et al. ACSL4 dictates ferroptosis sensitivity by shaping cellular lipid composition. Nat Chem Biol. 2017;13(1):91-98. doi: 10.1038/nchembio.2239
- Feng L, Yin X, Hua Q, Ren T, Ke J. Advancements in understanding the role of ferroptosis in hypxia-associated brain injury: A narrative review. Transl Pediatr. 2024;13(6):24-47. doi: 10.21037/tp-24-47
- Peeples ES, Genaro-Mattos TC. Ferroptosis: A promising therapeutic target for neonatal hypoxic-ischemic brain injury. Int J Mol Sci. 2022;23(13):7420. doi: 10.3390/ijms23137420
- Cheah JG, Kim SF, Hester LD, et al. NMDA receptor-nitric oxide transmission mediates neuronal iron homeostasis via the GTPase Dexras1. Neuron. 2006;51(4):431-440. doi: 10.1016/j.neuron.2006.07.011
- Yu J, Guo Y, Sun M, Li B, Zhang Y, Li C. Iron is a potential key mediator of glutamate excitotoxicity in spinal cord motor neurons. Brain Res. 2009;1257:102-107. doi: 10.1016/j.brainres.2008.12.030
- Parker D, Grillner S. Long-lasting substance-P-mediated modulation of NMDA-induced rhythmic activity in the lamprey locomotor network involves separate RNA-and protein-synthesis-dependent stages. Eur J Neurosci. 2008;11(5):1515-1522. doi: 10.1046/j.1460-9568.1999.00565.x
- Ferriero DM, Sheldon RA, Black SM, Chuai J. Selective destruction of nitric oxide synthase neurons with quisqualate reduces damage after hypoxia-ischemia in the neonatal rat. Pediatr Res. 1995;38(6):912-918. doi: 10.1203/00006450-199512000-00014
- Pulera MR, Adams LM, Liu H, et al. Apoptosis in a neonatal rat model of cerebral hypoxia-ischemia. Stroke. 1998;29(12):2622-2630. doi: 10.1161/01.str.29.12.2622
- Dorrepaal CA, Berger HM, Benders MJ, van Zoeren- Grobben D, Van de Bor M, Van Bel F. Nonprotein-bound iron in postasphyxial reperfusion injury of the newborn. Pediatrics. 1996;98(5):883-889.
- Ogihara T, Hirano K, Ogihara H, et al. Non-protein-bound transition metals and hydroxyl radical generation in cerebrospinal fluid of newborn infants with hypoxic ischemic encephalopathy. Pediatr Res. 2003;53(4):594-599. doi: 10.1203/01.PDR.0000054685.87405.59
- Evans PJ, Evans R, Kovar IZ, Holton AF, Halliwell B. Bleomycin-detectable iron in the plasma of premature and full-term neonates. FEBS Lett. 1992;303(2-3):210-212. doi: 10.1016/0014-5793(92)80521-h
- Khan JY, Black SM. Developmental changes in murine brain antioxidant enzymes. Pediatr Res. 2003;54(1):77-82. doi: 10.1203/01.PDR.0000065736.69214.20
- Roskams AJ, Connor JR. Iron, transferrin, and ferritin in the rat brain during development and aging. J Neurochem. 1994;63(2):709-716. doi: 10.1046/j.1471-4159.1994.63020709.x
- Taylor EM, Morgan EH. Developmental changes in transferrin and iron uptake by the brain in the rat. Brain Res Dev Brain Res. 1990;55(1):35-42. doi: 10.1016/0165-3806(90)90103-6
- Lin W, Zhang T, Zheng J, Zhou Y, Lin Z, Fu X. Ferroptosis is involved in hypoxic-ischemic brain damage in neonatal rats. Neuroscience. 2022;487:131-142. doi: 10.1016/j.neuroscience.2022.02.013
- Rice JE 3rd, Vannucci RC, Brierley JB. The influence of immaturity on hypoxic-ischemic brain damage in the rat. Ann Neurol. 1981;9(2):131-141. doi: 10.1002/ana.410090206
- Huo L, Fu J, Wang S, Wang H, Liu X. Emerging ferroptosis inhibitors as a novel therapeutic strategy for the treatment of neonatal hypoxic-ischemic encephalopathy. Eur J Med Chem. 2024;271:116453. doi: 10.1016/j.ejmech.2024.116453
- Zhang X, Ding M, Zhu P, et al. New insights into the Nrf-2/HO-1 signaling axis and its application in pediatric respiratory diseases. Oxid Med Cell Longev. 2019;2019:3214196. doi: 10.1155/2019/3214196
- Cai Y, Li X, Tan X, Wang P, Zhao X, Zhang H, et al. Vitamin D suppresses ferroptosis and protects against neonatal hypoxic-ischemic encephalopathy by activating the Nrf2/ HO-1 pathway. Transl Pediatr. 2022;11(10):1633-1644. doi: 10.21037/tp-22-397
- Tang Z, Cheng S, Sun Y, et al. Early TLR4 inhibition reduces hippocampal injury at puberty in a rat model of neonatal hypoxic-ischemic brain damage via regulation of neuroimmunity and synaptic plasticity. Exp Neurol. 2019;321:113039. doi: 10.1016/j.expneurol.2019.113039
- Fang X, Cai Z, Wang H, et al. Loss of cardiac ferritin H facilitates cardiomyopathy via Slc7a11-mediated ferroptosis. Circ Res. 2020;127(4):486-501. doi: 10.1161/CIRCRESAHA.120.316509
- Miao J, Benomar Y, Al Rifai S, et al. Resistin inhibits neuronal autophagy through Toll-like receptor 4. J Endocrinol. 2018;238(1):77-89. doi: 10.1530/JOE-18-0096
- Wu F, Wang Z, Gu J, et al. p38MAPK/p53-Mediated Bax induction contributes to neurons degeneration in rotenone-induced cellular and rat models of Parkinson’s disease. Neurochem Int. 2013;63(3):133-140. doi: 10.1016/j.neuint.2013.05.006
- Genaro-Mattos TC, Korade Z, Sahar NE, Angeli JPF, Mirnics K, Peeples ES. Enhancing 7-dehydrocholesterol suppresses brain ferroptosis and tissue injury after neonatal hypoxia-ischemia. Sci Rep. 2024;14(1):7924. doi: 10.1038/s41598-024-58579-6
- Zheng J, Fang Y, Zhang M, et al. Mechanisms of ferroptosis in hypoxic-ischemic brain damage in neonatal rats. Exp Neurol. 2024;372:114641. doi: 10.1016/j.expneurol.2023.114641
- Scarpellini C, Klejborowska G, Lanthier C, Hassannia B, Vanden Berghe T, Augustyns K. Beyond ferrostatin-1: A comprehensive review of ferroptosis inhibitors. Trends Pharmacol Sci. 2023;44(12):902-916. doi: 10.1016/j.tips.2023.08.012
- Alim I, Caulfield JT, Chen Y, et al. Selenium drives a transcriptional adaptive program to block ferroptosis and treat stroke. Cell. 2019;177(5):1262-1279.e25. doi: 10.1016/j.cell.2019.03.032