Heparan sulfate proteoglycans in pathological protein aggregation and brain functionality
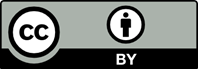
Proteoglycans are highly specialized proteins with specific interactive modules in their core proteins, allowing them to engage with a diverse range of cellular and structural proteins, contributing to many varied roles in cell processes. This review examines the roles of heparan sulfate (HS) proteoglycans (HSPGs) in the assembly of pathological protein aggregates in brain tissues, which negatively impact cognitive brain function. While HSPGs play roles in normal brain development, they also contribute to the abnormal accumulation and persistence of protein aggregates in Alzheimer’s and Parkinson’s diseases (AD and PD), prolonging their neurotoxic effects. HSPG-mediated effects on the innate immune system may also contribute to the development of neuroinflammation and pathogenesis of neurodegenerative changes in the central nervous system. These pathological developments lead to impaired cognition, permanent memory loss in dementia and AD, and an inability of the brain to exert normal neuromuscular control, affecting motor functions and body movement in PD. A deeper understanding of the properties of HS in these degenerative processes is essential for the development of therapeutic measures to treat these conditions. Significant improvements in neuroimaging, next-generation glycosaminoglycan analytical techniques, and functional glycomics hold great promise for elucidating the complexities of HS structure and function. These advancements may significantly aid in the development of HS biotherapeutics to treat these debilitating conditions, which are increasingly impacting the aging global population. While a number of promising therapeutic candidates have already emerged, further research is required to optimize their biotherapeutic applications in this challenging area of pathobiology, bioregulation, and the recovery of neural function.

- Xie C, Schaefer L, Iozzo V. Global impact of proteoglycan science on human diseases. iScience. 2023;26(11):108095. doi: 10.1016/j.isci.2023.108095
- Arikawa-Hirasawa E. Impact of the heparan sulfate proteoglycan perlecan on human disease and health. Am J Physiol Cell Physiol. 2022;322(6):C1117-C1122. doi: 10.1152/ajpcell.00113.2022
- Farrugia B, Melrose J. The glycosaminoglycan side chains and modular core proteins of heparan sulphate proteoglycans and the varied ways they provide tissue protection by regulating physiological processes and cellular behaviour. Int J Mol Sci. 2023;24(18):14101. doi: 10.3390/ijms241814101
- Hayes A, Melrose J. HS, an ancient molecular recognition and information storage glycosaminoglycan, equips HS-proteoglycans with diverse matrix and cell-interactive properties operative in tissue development and tissue function in health and disease. Int J Mol Sci. 2023;24(2):1148. doi: 10.3390/ijms24021148
- Guilak F, Hayes AJ, Melrose J. Perlecan in pericellular mechanosensory cell-matrix communication, extracellular matrix stabilisation and mechanoregulation of load-bearing connective tissues. Int I Mol Sci. 2021;22(5):2716. doi: 10.3390/ijms22052716
- Yamada S, Sugahara K, Ozbek S. Evolution of glycosaminoglycans: Comparative biochemical study. Commun Integr Biol. 2011;4(2):150-158. doi: 10.4161/cib.4.2.14547
- Esko JD, Lindahl U. Molecular diversity of heparan sulfate. J Clin Invest. 2001;108(2):169-173. doi: 10.1172/JCI13530
- Esko J, Selleck SB. Order out of chaos: Assembly of ligand binding sites in heparan sulfate. Annu Rev Biochem. 2002;71:435-471. doi: 10.1146/annurev.biochem.71.110601.135458
- Bishop J, Schuksz M, Esko JD. Heparan sulphate proteoglycans fine-tune mammalian physiology. Nature. 2007;446(7139):1030-1037. doi: 10.1038/nature05817
- Kreuger J, Kjellén L. Heparan sulfate biosynthesis: Regulation and variability. J Histochem Cytochem. 2012;60(12):898-907. doi: 10.1369/0022155412464972
- Basu A, Patel NG, Nicholson ED, Weiss RJ. Spatiotemporal diversity and regulation of glycosaminoglycans in cell homeostasis and human disease. Am J Physiol Cell Physiol. 2022;322(5):C849-C864. doi: 10.1152/ajpcell.00085.2022
- Cummings R. The repertoire of glycan determinants in the human glycome. Mol Biosyst. 2009;5(10):1087-1104. doi: 10.1039/b907931a
- Sasisekharan R, Venkataraman G. Heparin and heparan sulfate: Biosynthesis, structure and function. Curr Opin Chem Biol. 2000;4(6):626-631. doi: 10.1016/s1367-5931(00)00145-9
- Corti F, Wang Y, Rhodes JM, et al. N-terminal syndecan-2 domain selectively enhances 6-O heparan sulfate chains sulfation and promotes VEGFA165-dependent neovascularization. Nat Commun. 2019;10(1):1562. doi: 10.1038/s41467-019-09605-z
- Holmes S, Nagarajan B, Desai UR. 3-O-Sulfation induces sequence-specific compact topologies in heparan sulfate that encode a dynamic sulfation code. Comput Struct Biotechnol J. 2022;20:3884-3898. doi: 10.1016/j.csbj.2022.07.013
- Thacker B, Xu D, Lawrence R, Esko JD. Heparan sulfate 3-O-sulfation: A rare modification in search of a function. Matrix Biol. 2014;35:60-72. doi: 10.1016/j.matbio.2013.12.001
- Zhao J, Zhu Y, Song X, et al. 3-O-Sulfation of heparan sulfate enhances tau interaction and cellular uptake. Angew Chem Int Ed Engl. 2020;59(5):1818-1827. doi: 10.1002/anie.201913029
- Hayashida K, Aquino RS, Park PW. Coreceptor functions of cell surface heparan sulfate proteoglycans. Am J Physiol Cell Physiol. 2022;322(5):C896-C912. doi: 10.1152/ajpcell.00050.2022
- Mah D, Zhao J, Liu X, et al. The sulfation code of tauopathies: Heparan sulfate proteoglycans in the prion like spread of tau pathology. Front Mol Biosci. 2021;8:671458. doi: 10.3389/fmolb.2021.671458
- Stopschinski B, Holmes BB, Miller GM, et al. Specific glycosaminoglycan chain length and sulfation patterns are required for cell uptake of tau versus α-synuclein and β-amyloid aggregates. J Biol Chem. 2018;293(27):10826-10840. doi: 10.1074/jbc.RA117.000378
- Hudák A, Kusz E, Domonkos I, et al. Contribution of syndecans to cellular uptake and fibrillation of α-synuclein and tau. Sci Rep. 2019;9(1):16543. doi: 10.1038/s41598-019-53038-z
- Vasili E, Dominguez-Meijide A, Outeiro TF. Spreading of α-Synuclein and Tau: A systematic comparison of the mechanisms involved. Front Mol Neurosci. 2019;12:107. doi: 10.3389/fnmol.2019.00107
- Holmes B, DeVos SL, Kfoury N, et al. Heparan sulfate proteoglycans mediate internalization and propagation of specific proteopathic seeds. Proc Nat Acad Sci U S A. 2013;110(33):E3138-E3147. doi: 10.1073/pnas.1301440110
- Holmes B, Diamond MI. Prion-like properties of Tau protein: The importance of extracellular Tau as a therapeutic target. J Biol Chem. 2014;289(29):19855-19861. doi: 10.1074/jbc.R114.549295
- Valko M, Jomova K, Rhodes CJ, Kuča K, Musílek K. Redox-and non-redox-metal-induced formation of free radicals and their role in human disease. Arch Toxicol. 2016;90(1):1-37. doi: 10.1007/s00204-015-1579-5
- Melrose J. The potential of flavonoids and flavonoid metabolites in the treatment of neurodegenerative pathology in disorders of cognitive decline. Antioxidants (Basel). 2023;12(3):663. doi: 10.3390/antiox12030663
- Vargas J, Grudina C, Zurzolo C. The prion-like spreading of α-synuclein: From in vitro to in vivo models of Parkinson’s disease. Ageing Res Rev. 2019;50:89-101. doi: 10.1016/j.arr.2019.01.012
- Zhu Y, Gandy L, Zhang F, et al. Heparan sulfate proteoglycans in tauopathy. Biomolecules. 2022;12(12):1792. doi: 10.3390/biom12121792
- Nguyen DLB, Okolicsanyi RK, Haupt SLM. Heparan sulfate proteoglycans: Mediators of cellular and molecular Alzheimer’s disease pathogenic factors via tunnelling nanotubes? Mol Cell Neurosci. 2024;129:103936. doi: 10.1016/j.mcn.2024.103936
- Snow AD, Cummings JA, Lake T. The unifying hypothesis of Alzheimer’s disease: Heparan sulfate proteoglycans/ glycosaminoglycans are key as first hypothesized over 30 years ago. Front Aging Neurosci. 2021;13:710683. doi: 10.3389/fnagi.2021.710683
- Kim SY, Jin W, Sood A, et al. Characterization of heparin and severe acute respiratory syndrome-related coronavirus 2 (SARS-CoV-2) spike glycoprotein binding interactions. Antiviral Res. 2020;181:104873. doi: 10.1016/j.antiviral.2020.104873
- Chen X, Nakada S, Donahue JE, et al. Neuroprotective effects of inter-alpha inhibitor proteins after hypoxic-ischemic brain injury in neonatal rats. Exp Neurol. 2019;317:244-259. doi: 10.1016/j.expneurol.2019.03.013
- Takano M, Mori Y, Shiraki H, et al. Detection of bikunin mRNA in limited portions of rat brain. Life Sci. 1999;65(8):757-762. doi: 10.1016/s0024-3205(99)00302-1
- Maroney SA, Westrick RJ, Cleuren AC, et al. Tissue factor pathway inhibitor is required for cerebrovascular development in mice. Blood. 2021;137(2):258-268. doi: 10.1182/blood.2020006054
- Eigenbrot C, Ganesan R, Kirchhofer D. Hepatocyte growth factor activator (HGFA): Molecular structure and interactions with HGFA inhibitor-1 (HAI-1). FEBS J. 2010;277(10):2215-2222. doi: 10.1111/j.1742-4658.2010.07638.x
- Salameh MA, Robinson JL, Navaneetham D, et al. The amyloid precursor protein/protease nexin 2 Kunitz inhibitor domain is a highly specific substrate of mesotrypsin. J Biol Chem. 2010;285(3):1939-1949. doi: 10.1074/jbc.M109.057216
- Dar NJ, Glazner GW. Deciphering the neuroprotective and neurogenic potential of soluble amyloid precursor protein alpha (sAPPα). Cell Mol Life Sci. 2020;77(12):2315-2330. doi: 10.1007/s00018-019-03404-x
- Zhang Z, Tian Y, Ye K. δ-secretase in neurodegenerative diseases: Mechanisms, regulators and therapeutic opportunities. Transl Neurodegener. 2020;9:1. doi: 10.1186/s40035-019-0179-3
- Marr HS, Basalamah MA, Bouldin TW, Duncan AW, Edgell CJ. Distribution of testican expression in human brain. Cell Tissue Res. 2000;302(2):139-144. doi: 10.1007/s004410000277
- Barrera-Ocampo A, Arlt S, Matschke J, et al. Amyloid-beta precursor protein modulates the sorting of testican-1 and contributes to its accumulation in brain tissue and cerebrospinal fluid from patients with Alzheimer disease. J Neuropathol Exp Neurol. 2016;75(9):903-916. doi: 10.1093/jnen/nlw065
- Smith SM, Melrose J. A retrospective analysis of the cartilage kunitz protease inhibitory proteins identifies these as members of the inter-α-trypsin inhibitor superfamily with potential roles in the protection of the articulatory surface. Int J Mol Sci. 2019;20(3):497. doi: 10.3390/ijms20030497
- Qian Y, Yang L, Chen J, et al. SRGN amplifies microglia-mediated neuroinflammation and exacerbates ischemic brain injury. J Neuroinflammation. 2024;21(1):35. doi: 10.1186/s12974-024-03026-6
- Liu P, Li HQ, Dong MQ, et al. Infiltrating myeloid cell-derived properdin markedly promotes microglia-mediated neuroinflammation after ischemic stroke. J Neuroinflammation. 2023;20(1):260. doi: 10.1186/s12974-023-02946-z
- Michels MAH, Volokhina EB, van de Kar NCA, van den Heuvel LPW. The role of properdin in complement-mediated renal diseases: A new player in complement-inhibiting therapy? Pediatr Nephrol. 2019;34(8):1349-1367. doi: 10.1007/s00467-018-4042-z
- Kurz C, Walker L, Rauchmann BS, Perneczky R. Dysfunction of the blood-brain barrier in Alzheimer’s disease: Evidence from human studies. Neuropathol Appl Neurobiol. 2022;48:e12782. doi: 10.1111/nan.12782
- Saitoh M, Takayama K, Hitachi K, et al. Discovery of a follistatin-derived myostatin inhibitory peptide. Bioorg Med Chem Lett. 2020;30(3):126892. doi: 10.1016/j.bmcl.2019.126892
- Stetefeld J, Jenny M, Schulthess T, et al. The laminin-binding domain of agrin is structurally related to N-TIMP-1. Nat Struct Biol. 2001;8(8):705-709. doi: 10.1038/90422
- Song L, Oseid DE, Wells EA, Coaston T, Robinson AS. Heparan sulfate proteoglycans (HSPGs) serve as the mediator between monomeric tau and its subsequent intracellular ERK1/2 pathway activation. J Mol Neurosci. 2022;72(4):772-791. doi: 10.1007/s12031-021-01943-2
- Rauch J, Chen JJ, Sorum AW, et al. Tau internalization is regulated by 6-O sulfation on heparan sulfate proteoglycans (HSPGs). Sci Rep. 2018;8(1):6382. doi: 10.1038/s41598-018-24904-z
- Sikorska B, Liberski PP. Human prion diseases: From kuru to variant Creutzfeldt-Jakob disease. Subcell Biochem. 2012;65:457-496. doi: 10.1007/978-94-007-5416-4_17
- Hara H, Chida J, Uchiyama K, et al. Neurotropic influenza A virus infection causes prion protein misfolding into infectious prions in neuroblastoma cells. Sci Rep. 2021;11(1):10109. doi: 10.1038/s41598-021-89586-6
- Lukiw W, Jaber VR, Pogue AI, Zhao Y. SARS-CoV-2 invasion and pathological links to prion disease. Biomolecules. 2022;12(9):1253. doi: 10.3390/biom12091253
- Yamashita S, Kamatari YO, Honda R, et al. Monomeric a-synuclein (aS) inhibits amyloidogenesis of human prion protein (hPrP) by forming a stable aS-hPrP hetero-dimer. Prion. 2021;15(1):37-43. doi: 10.1080/19336896.2021.1910176
- Wakhloo D, Oberhauser J, Madira A, Mahajani S. From cradle to grave: Neurogenesis, neuroregeneration and neurodegeneration in Alzheimer’s and Parkinson’s diseases. Neural Regen Res. 2022;17:2606-2614. doi: 10.4103/1673-5374.336138
- Iwahashi N, Ikezaki M, Nishikawa T, et al. Sulfated glycosaminoglycans mediate prion-like behavior of p53 aggregates. Proc Natl Acad Sci U S A. 2020;117(52):33225-33234. doi: 10.1073/pnas.2009931117
- Zhang Q, Ma C, Chin LS, Li L. Integrative glycoproteomics reveals protein N-glycosylation aberrations and glycoproteomic network alterations in Alzheimer’s disease. Sci Adv. 2020;6(40):eabc5802. doi: 10.1126/sciadv.abc5802
- Castillo GM, Lukito W, Wight TN, Snow AD. The sulfate moieties of glycosaminoglycans are critical for the enhancement of beta-amyloid protein fibril formation. J Neurochem. 1999;72(4):1681-1687. doi: 10.1046/j.1471-4159.1999.721681.x
- Parham C, Auckland L, Rachwal J, Clarke D, Bix G. Perlecan domain V inhibits amyloid-β induced brain endothelial cell toxicity and restores angiogenic function. J Alzheimers Dis. 2014;38(2):415-423. doi: 10.3233/JAD-130683
- Parham CL, Shaw C, Auckland LD, Dickeson SK, Griswold- Prenner I, Bix G. Perlecan domain V inhibits amyloid-β induced activation of the α2β1 integrin-mediated neurotoxic signaling cascade. J Alzheimers Dis. 2016;54(4):1629-1647. doi: 10.3233/JAD-160290
- Trout A, Kahle MP, Roberts JM, et al. Perlecan domain-V enhances neurogenic brain repair after stroke in mice. Transl Stroke Res. 2021;12(1):72-86. doi: 10.1007/s12975-020-00800-5
- Lee B, Clarke D, Al Ahmad A, et al. Perlecan domain V is neuroprotective and proangiogenic following ischemic stroke in rodents. J Clin Invest. 2011;121(8):3005-3023. doi: 10.1172/JCI46358
- Lorente-Gea, L, Garcia B, Martin C, et al. Heparan sulfate proteoglycans undergo differential expression alterations in Alzheimer disease brains. J Neuropathol Exp Neurol. 2020;79(5):474-483. doi: 10.1093/jnen/nlaa016
- Doan R, Bae BI, Cubelos B, et al. Mutations in human accelerated regions disrupt cognition and social behavior. Cell. 2016;167(2):341-354.e12. doi: 10.1016/j.cell.2016.08.071
- Letoha T, Hudak A, Kusz E, et al. Contribution of syndecans to cellular internalization and fibrillation of amyloid-β(1-42). Sci Rep. 2019;9(1):1393. doi: 10.1038/s41598-018-37476-9
- Lauri SE, Kaukinen S, Kinnunen T, et al. Regulatory role and molecular interactions of a cell-surface heparan sulfate proteoglycan (N-syndecan) in hippocampal long-term potentiation. J Neurosci. 1999;19(4):1226-1235. doi: 10.1523/JNEUROSCI.19-04-01226.1999
- Kranenburg O, Kroon-Batenburg LM, Reijerkerk A, Wu YP, Voest EE, Gebbink MF. Recombinant endostatin forms amyloid fibrils that bind and are cytotoxic to murine neuroblastoma cells in vitro. FEBS Lett. 2003;539(1-3):149-155. doi: 10.1016/s0014-5793(03)00218-7
- Van Horssen J, Wilhelmus MM, Heljasvaara R, et al. Collagen XVIII: A novel heparan sulfate proteoglycan associated with vascular amyloid depositions and senile plaques in Alzheimer’s disease brains. Brain Pathol. 2002;12(4):456-462. doi: 10.1111/j.1750-3639.2002.tb00462.x
- Tanase C, Enciu AM, Codrici E, et al. Fatty acids, CD36, thrombospondin-1, and CD47 in glioblastoma: Together and/or separately? Int J Mol Sci. 2022;23(2):604. doi: 10.3390/ijms23020604
- Cuttler K, Hassan M, Carr J, Cloete R, Bardien S. Emerging evidence implicating a role for neurexins in neurodegenerative and neuropsychiatric disorders. Open Biol. 2021;11(10):210091. doi: 10.1098/rsob.210091
- Hudák A, Letoha A, Vizler C, Letoha T. Syndecan-3 as a novel biomarker in Alzheimer’s disease. Int J Mol Sci. 2022;23(6):3407. doi: 10.3390/ijms23063407
- Saroja SR, Gorbachev K, Julia T, Goate AM, Pereira AC. Astrocyte-secreted glypican-4 drives APOE4-dependent tau hyperphosphorylation. Proc Natl Acad Sci U S A. 2022;119(34):e2108870119. doi: 10.1073/pnas.2108870119
- García-Prieto IID, Lopez-Martín S, Albert J, et al. Mutations in the COL18A1 gen associated with knobloch syndrome and structural brain anomalies: A novel case report and literature review of neuroimaging findings. Neurocase. 2022;28(1):11-18. doi: 10.1080/13554794.2021.1928228
- Liu I, Uversky VN, Munishkina LA, Fink AL, Halfter W, Cole GJ. Agrin binds alpha-synuclein and modulates alpha-synuclein fibrillation. Glycobiology. 2005;15(12):1320-1331. doi: 10.1093/glycob/cwj014
- Miller J, Cummings J, Maresh GA, et al. Localization of perlecan (or a perlecan-related macromolecule) to isolated microglia in vitro and to microglia/macrophages following infusion of beta-amyloid protein into rodent hippocampus. Glia. 1997;21(2):228-243. doi: 10.1002/(sici)1098-1136(199710)21:2<228:aid-glia6>3.0.co;2-2
- Wu B, McDonald AJ, Markham K, et al. The N-terminus of the prion protein is a toxic effector regulated by the C-terminus. Elife. 2017;6:e23473. doi: 10.7554/eLife.23473
- Jouanne M, Rault S, Voisin-Chiret AS. Tau protein aggregation in Alzheimer’s disease: An attractive target for the development of novel therapeutic agents. Eur J Med Chem. 2017;139:153-167. doi: 10.1016/j.ejmech.2017.07.070
- Fields CR, Bengoa-Vergniory N, Wade-Martins R. Targeting Alpha-synuclein as a therapy for Parkinson’s disease. Front Mol Neurosci. 2019;12:299. doi: 10.3389/fnmol.2019.00299
- Vidović M, Rikalovic MG. Alpha-synuclein aggregation pathway in Parkinson’s disease: Current status and novel therapeutic approaches. Cells. 2022;11(11):1732. doi: 10.3390/cells11111732
- Pan L, Li C, Meng L, et al. Tau accelerates α-synuclein aggregation and spreading in Parkinson’s disease. Brain. 2022;145(10):3454-3471. doi: 10.1093/brain/awac171
- Javed H, Nagoor Meeran MF, Azimullah S, Adem A, Sadek B, Ojha SK. Plant extracts and phytochemicals targeting α-synuclein aggregation in Parkinson’s disease models. Front Pharmacol. 2019;9:1555. doi: 10.3389/fphar.2018.01555
- De Andrade Teles RB, Diniz TC, Costa Pinto TC, et al. Flavonoids as therapeutic agents in Alzheimer’s and Parkinson’s diseases: A systematic review of preclinical evidences. Oxid Med Cell Longev. 2018;2018:7043213. doi: 10.1155/2018/7043213
- Cryan JF, O’Riordan KJ, Cowan CSM, et al. The microbiota-gut-brain axis. Physiol Rev. 2019;99(4):1877-2013. doi: 10.1152/physrev.00018.2018
- Vincent AJ, Gasperini R, Foa L, Small DH. Astrocytes in Alzheimer’s disease: Emerging roles in calcium dysregulation and synaptic plasticity. J Alzheimers Dis. 2010;22(3):699-714. doi: 10.3233/JAD-2010-101089
- Melrose J, Smith MM. Natural and Semi-synthetic flavonoid anti-SARS-CoV-2 agents for the treatment of long COVID-19 disease and neurodegenerative disorders of cognitive decline. Front Biosci (Elite Ed). 2022;14(4):27. doi: 10.31083/j.fbe1404027
- Li HL, Zhang SY, Ren YS, et al. Identification of ellagic acid and urolithins as natural inhibitors of Aβ25-35-induced neurotoxicity and the mechanism predication using network pharmacology analysis and molecular docking. Front Nutr. 2022;9:966276. doi: 10.3389/fnut.2022.966276
- Kaufman AC, Salazar SV, Haas LT, et al. Fyn inhibition rescues established memory and synapse loss in Alzheimer mice. Ann Neurol. 2015;77(6):953-971. doi: 10.1002/ana.24394
- Uchimura K, Nishitsuji K, Chiu LT, et al. Design and synthesis of 6-O-phosphorylated heparan sulfate oligosaccharides to inhibit amyloid β aggregation. Chembiochem. 2022;23(15):e202200191. doi: 10.1002/cbic.202200191
- Liu CC, Zhao N, Yamaguchi Y, et al. Neuronal heparan sulfates promote amyloid pathology by modulating brain amyloid-β clearance and aggregation in Alzheimer’s disease. Sci Transl Med. 2016;8(332):332ra44. doi: 10.1126/scitranslmed.aad3650
- Patey SJ, Edwards EA, Yates EA, Turnbull JE. Engineered heparins: Novel beta-secretase inhibitors as potential Alzheimer’s disease therapeutics. Neurodegener Dis. 2008;5(3-4):197-199. doi: 10.1159/000113701
- Zhang J, Liang L, Yang W, et al. Expedient synthesis of a library of heparan sulfate-like “Head-to-Tail” linked multimers for structure and activity relationship studies. Angew Chem Int Ed Engl. 2022;61(48):e202209730. doi: 10.1002/anie.202209730
- Yang C, Deng Y, Wang Y, et al. Heparan sulfate glycomimetics via iterative assembly of “clickable” disaccharides. Chem Sci. 2023;14(13):3514-3522. doi: 10.1039/d3sc00260h
- Wakpal J, Pathiranage V, Walker AR, Nguyen HM. Rational design and expedient synthesis of heparan sulfate mimetics from natural aminoglycosides for structure and activity relationship studies. Angew Chem Int Ed Engl. 2023;62(32):e202304325. doi: 10.1002/anie.202304325
- Casu B, Naggi A, Torri G. Heparin-derived heparan sulfate mimics to modulate heparan sulfate-protein interaction in inflammation and cancer. Matrix Biol. 2010;29(6):442-452. doi: 10.1016/j.matbio.2010.04.003
- Kepp K, Robakis NK, Høilund-Carlsen PF, Sensi SL, Vissel B. The amyloid cascade hypothesis: An updated critical review. Brain. 2023;146(10):3969-3990. doi: 10.1093/brain/awad159
- Michaels TCT, Qian D, Šarić A, Vendruscolo M, Linse S, Knowles TPJ. Amyloid formation as a protein phase transition. Nat Rev Phys. 2023;5:379-397. doi: 10.1038/s42254-023-00598-9
- Chowdhury S, Sarkar N. Exploring the potential of amyloids in biomedical applications: A review. Biotechnol Bioeng. 2024;121(1):26-38. doi: 10.1002/bit.28569