The effect of thermal rest time during in situ alloying of Ti41Nb through laser powder bed fusion
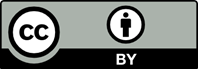
This study investigates the impact of thermal rest time variation on in situ alloying of Ti41Nb (wt.%) within a single part, building on previous research that utilized a short stripe width scanning strategy to promote interaction between individual melt pools. Employing a contour scan from the part center outwards, diverse thermal rest times were achieved at different locations within the component. Results revealed a significant influence of varying thermal rest times on the amount of unmelted Nb and mechanical properties of the part. The findings underscore the importance of considering thermal rest time in in situ alloying and laser powder bed fusion (LPBF) printing in general, as it can markedly affect print quality. This study contributes valuable insights for optimizing LPBF processing parameters and advancing the understanding of in situ alloying through LPBF.
- Chua CK, Leong KF. 3D Printing and Additive Manufacturing: Principles and Applications. Singapore: World Scientific; 2017. doi: 10.1142/10200
- Radhakrishnan J, Kumar P, Gan SS, Bryl A, McKinnell J, Ramamurty U. Fatigue resistance of the binder jet printed 17-4 precipitation hardened martensitic stainless steel. Mater Sci Eng A. 2023;865:144451. doi: 10.1016/j.msea.2022.144451
- Radhakrishnan J, Kumar P, Gan SS, Bryl A, McKinnell J, Ramamurty U. Microstructure and tensile properties of binder jet printed 17-4 precipitation hardened martensitic stainless steel. Mater Sci Eng A. 2022;860:144270. doi: 10.1016/j.msea.2022.144270
- Li SH, Kumar P, Chandra S, Ramamurty U. Directed energy deposition of metals: Processing, microstructures, and mechanical properties. Int Mater Rev. 2022;68:1-43. doi: 10.1080/09506608.2022.2097411
- Huang S, Kumar P, Lim CWJ, Radhakrishnan J, Ramamurty U. Fracture behavior of PH15-5 stainless steel manufactured via directed energy deposition. Mater Des. 2023;235:112421. doi: 10.1016/j.matdes.2023.112421
- Wei F, Wei S, Lau KB, et al. Compositionally graded AlxCoCrFeNi high-entropy alloy manufactured by laser powder bed fusion. Materialia. 2022;21:101308. doi: 10.1016/j.mtla.2021.101308
- Brodie EG, Medvedev AE, Frith JE, Dargusch MS, Fraser HL, Molotnikov A. Remelt processing and microstructure of selective laser melted Ti25Ta. J Alloys Compd. 2020;820:153082. doi: 10.1016/j.jallcom.2019.153082
- Brodie EG, Richter J, Wegener T, Niendorf T, Molotnikov A. Low-cycle fatigue performance of remelted laser powder bed fusion (L-PBF) biomedical Ti25Ta. Mater Sci Eng A. 2020;798:140228. doi: 10.1016/j.msea.2020.140228
- Wang C, Chandra S, Huang S, Tor SB, Tan X. Unraveling process-microstructure-property correlations in powder-bed fusion additive manufacturing through information-rich surface features with deep learning. J Mater Process Technol. 2023;311:117804. doi: 10.1016/j.jmatprotec.2022.117804
- Chandra S, Tan X, Narayan RL, Wang C, Tor SB, Seet G. A generalised hot cracking criterion for nickel-based superalloys additively manufactured by electron beam melting. Addit Manuf. 2021;37:101633. doi: 10.1016/j.addma.2020.101633
- Xiang DD, Wang P, Tan XP, et al. Anisotropic microstructure and mechanical properties of additively manufactured Co-Cr-Mo alloy using selective electron beam melting for orthopedic implants. Mater Sci Eng A. 2019;765:138270. doi: 10.1016/j.msea.2019.138270
- Tan XP, Chandra S, Kok Y, et al. Revealing competitive columnar grain growth behavior and periodic microstructural banding in additively manufactured Ti-6Al-4 V parts by selective electron beam melting. Materialia. 2019;7:100365. doi: 10.1016/j.mtla.2019.100365
- Kumar P, Huang S, Cook DH, et al. A strong fracture-resistant high-entropy alloy with nano-bridged honeycomb microstructure intrinsically toughened by 3D-printing. Nat Commun. 2024;15(1):841. doi: 10.1038/s41467-024-45178-2
- Loh LE, Chua CK, Zhang WY, et al. Numerical investigation and an effective modelling on the Selective Laser Melting (SLM) process with aluminium alloy 6061. Int J Heat Mass Transf. 2015;80:288-300. doi: 10.1016/j.ijheatmasstransfer.2014.09.014
- Wang D, Liu L, Deng G, et al. Recent progress on additive manufacturing of multi-material structures with laser powder bed fusion. Virtual Phys Prototyp. 2022;17:329-365. doi: 10.1080/17452759.2022.2028343
- Yao L, Huang S, Ramamurty U, Xiao Z. On the formation of “Fish-scale” morphology with curved grain interfacial microstructures during selective laser melting of dissimilar alloys. Acta Mater. 2021;220:117331. doi: 10.1016/j.actamat.2021.117331
- Yao L, Xiao Z, Huang S, Ramamurty U. The formation mechanism of metal-ceramic interlayer interface during laser powder bed fusion. Virtual Phys Prototyp. 2023;18(1):e2235324. doi: 10.1080/17452759.2023.2235324
- Huang S, Kumar P, Yeong WY, Narayan RL, Ramamurty U. Fracture behavior of laser powder bed fusion fabricated Ti41Nb via in-situ alloying. Acta Mater. 2022;225:117593. doi: 10.1016/j.actamat.2021.117593
- Dong Z, Han C, Zhao Y, et al. Role of heterogenous microstructure and deformation behavior in achieving superior strength-ductility synergy in zinc fabricated via laser powder bed fusion. Int J Extrem Manuf. 2024;6(4):045003. doi: 10.1088/2631-7990/ad3929
- Kumar P, Ramamurty U. High cycle fatigue in selective laser melted Ti-6Al-4V. Acta Mater. 2020;194:305-320. doi: 10.1016/j.actamat.2020.05.041
- Li Y, Yang C, Zhao H, Qu S, Li X, Li Y. New developments of Ti-based alloys for biomedical applications. Materials (Basel). 2014;7(3):1709-1800. doi: 10.3390/ma7031709
- Wolff J. Das Gesetz der Transformation der Knochen. Vol. 1. Berlin: Verlag von August Hirschwald; 1892. p. 1-152.
- Niinomi M, Liu Y, Nakai M, Liu H, Li H. Biomedical titanium alloys with young’s moduli close to that of cortical bone. Regen Biomater. 2016;3(3):173-185. doi: 10.1093/rb/rbw016
- Niinomi M. Recent metallic materials for biomedical applications. Metall Mater Trans A. 2002;33(3):477-486. doi: 10.1007/s11661-002-0109-2
- Kuroda D, Niinomi M, Morinaga M, Kato Y, Yashiro T. Design and mechanical properties of new β type titanium alloys for implant materials. Mater Sci Eng A. 1998;243(1):244-249. doi: 10.1016/S0921-5093(97)00808-3
- Wang Q, Han C, Choma T, et al. Effect of Nb content on microstructure, property and in vitro apatite-forming capability of Ti-Nb alloys fabricated via selective laser melting. Mater Des. 2017;126:268-277. doi: 10.1016/j.matdes.2017.04.026
- Huang S, Narayan RL, Tan JHK, Sing SL, Yeong WY. Resolving the porosity-unmelted inclusion dilemma during In-situ alloying of Ti34Nb via laser powder bed fusion. Acta Mater. 2021;204:116522. doi: 10.1016/j.actamat.2020.116522
- Huang S, Sing SL, Delooze G, Wilson R, Yeong WY. Laser powder bed fusion of titanium-tantalum alloys: Compositions and designs for biomedical applications. J Mech Behav Biomed Mater. 2020;108:103775. doi: 10.1016/j.jmbbm.2020.103775
- Huang S, Sing SL, Yeong WY. Selective laser melting of Ti42Nb composite powder and the effect of laser re-melting. Key Eng Mater. 2019;801:270-275. doi: 10.4028/www.scientific.net/KEM.801.270
- Sing SL, Wiria FE, Yeong WY. Selective laser melting of titanium alloy with 50 wt% tantalum: Effect of laser process parameters on part quality. Int J Refract Met Hard Mater. 2018;77:120-127. doi: 10.1016/j.ijrmhm.2018.08.006
- Sing SL, Wiria FE, Yeong WY. Selective laser melting of lattice structures: A statistical approach to manufacturability and mechanical behavior. Robot Comput Integr Manuf. 2018;49:170-180. doi: 10.1016/j.rcim.2017.06.006
- Huang S, Yeong WY. Laser Re-scanning Strategy in Selective Laser Melting for Part Quality Enhancement: A Review. In: Proceedings of the 3rd International Conference on Progress in Additive Manufacturing (ProAM 2018); 2018. doi: 10.25341/D4GP4J
- Gao M, He D, Cui L, et al. Investigation on the microstructure and mechanical properties of the ti-ta alloy with unmelted ta particles by laser powder bed fusion. Materials (Basel). 2023;16(6):2208. doi: 10.3390/ma16062208
- Long M, Rack HJ. Titanium alloys in total joint replacement--a materials science perspective. Biomaterials. 1998;19(18):1621-1639. doi: 10.1016/s0142-9612(97)00146-4
- Ozaki T, Matsumoto H, Watanabe S, Hanada S. Beta ti alloys with low young’s modulus. Mater Trans. 2004;45(8):2776-2779. doi: 10.2320/matertrans.45.2776
- Fischer M, Joguet D, Robin G, Peltier L, Laheurte P. In situ elaboration of a binary Ti-26Nb alloy by selective laser melting of elemental titanium and niobium mixed powders. Mater Sci Eng C Mater Biol Appl. 2016;62:852-859. doi: 10.1016/j.msec.2016.02.033
- Tang C, Le KQ, Wong CH. Physics of humping formation in laser powder bed fusion. Int J Heat Mass Transf. 2020;149:119172. doi: 10.1016/j.ijheatmasstransfer.2019.119172
- Bönisch M, Panigrahi A, Calin M, et al. Thermal stability and latent heat of Nb-rich martensitic Ti-Nb alloys. J Alloys Compd. 2017;697:300-309. doi: 10.1016/j.jallcom.2016.12.108
- Wang W, Zhang X, Mei W, Sun J. Role of omega phase evolution in plastic deformation of twinning-induced plasticity β Ti-12V-2Fe-1Al alloy. Mater Des. 2020;186:108282. doi: 10.1016/j.matdes.2019.108282
- Lai MJ, Tasan CC, Zhang J, Grabowski B, Huang LF, Raabe D. Origin of shear induced β to ω transition in Ti-Nb-based alloys. Acta Mater. 2015;92:55-63. doi: 10.1016/j.actamat.2015.03.040