Advanced approaches with combination of 2D nanomaterials and 3D printing for exquisite neural tissue engineering
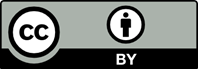
The regeneration of neural tissue presents significant challenges due to the complexity of the nervous system, which can be damaged by traumatic and non-traumatic injuries. Traditional approaches to repairing nerve damage, such as transplantation of auto-, allo-, or xeno-grafts, have limitations. Recently, researchers have been studying the use of two-dimensional (2D) nanomaterials as novel therapeutic approaches for treating neurological disorders. This review first presents the classification of 2D nanoparticles for neural regeneration and their biocompatibility. Thereafter, we discuss the recent approaches combining 2D nanomaterials and three-dimensional (3D) printing to produce hydrogel-based scaffolds for exquisite neural tissue engineering. Furthermore, recent highlighted studies focusing on the combination of 2D nanomaterials and 3D bioprinting for neural tissue engineering are specially introduced. Through this review, we aim to contribute to crafting a range of strategies for the biomedical applications of 3D printing with diverse nanomaterials and hope to encourage further research on understanding the mechanisms of toxicity as well as the synergistic effects of 2D nanomaterials and hydrogel scaffolds for neural tissue engineering and regeneration.
Dong-Wook Han serves as the Editorial Board Member of the journal, but did not in any way involve in the editorial and peer-review process conducted for this paper, directly or indirectly.
- López-Cebral R, Silva-Correia J, Reis R, et al., 2017, Peripheral nerve injury: Current challenges, conventional treatment approaches, and new trends in biomaterials-based regenerative strategies. ACS Biomater Sci Eng, 3: 3098–3122. https://doi.org/10.1021/acsbiomaterials.7b00655
- Nguyen R, Fiest KM, McChesney J, et al., 2016, The international incidence of traumatic brain injury: A systematic review and meta-analysis. Can J Neurol Sci, 43: 774–785. https://doi.org/10.1017/cjn.2016.290
- Liu R, Page M, Solheim K, et al., 2009, Quality of life in adults with brain tumors: Current knowledge and future directions. Neuro Oncol, 11: 330–339. https://doi.org/10.1215/15228517-2008-093
- Corps KN, Roth TL, McGavern DB, 2015, Inflammation and neuroprotection in traumatic brain injury. JAMA Neurol, 72: 355–362. https://doi.org/10.1001/jamaneurol.2014.3558
- Alam A, Thelin EP, Tajsic T, et al., 2020, Cellular infiltration in traumatic brain injury. J Neuroinflammation, 17: 328.
- Qin D, Wang J, Le A, et al., 2021, Traumatic brain injury: Ultrastructural features in neuronal ferroptosis, glial cell activation and polarization, and blood-brain barrier breakdown. Cells, 10: 1009. https://doi.org/10.3390/cells10051009
- Rosso G, Liashkovich I, Gess B, et al., 2014, Unravelling crucial biomechanical resilience of myelinated peripheral nerve fibres provided by the Schwann cell basal lamina and PMP22. Sci Rep, 4: 7286.
- Pindrik J, Belzberg AJ, 2014, Peripheral nerve surgery: Primer for the imagers. Neuroimaging Clin N Am, 24: 193–210. https://doi.org/10.1016/j.nic.2013.03.034
- Faisal SN, Iacopi F, 2022, Thin-film electrodes based on two-dimensional nanomaterials for neural interfaces. ACS Appl Nano Mater, 5: 10137–10150.
- Capasso A, Rodrigues J, Moschetta M, et al., 2021, Interactions between primary neurons and graphene films with different structure and electrical conductivity. Adv Funct Mater, 31: 2005300.
- Li Y, Hu Y, Wei H, et al., 2022, Two-dimensional Ti3C2Tx MXene promotes electrophysiological maturation of neural circuits. J Nanobiotechnology, 20: 398.
- Raja IS, Kang MS, Hong SW, et al., 2023, State-of-the-art techniques for promoting tissue regeneration: Combination of three-dimensional bioprinting and carbon nanomaterials. Int J Bioprint, 9: 635. https://doi.org/10.18063/ijb.v9i1.635
- Naghieh S, Sarker M, Abelseth E, et al., 2019, Indirect 3D bioprinting and characterization of alginate scaffolds for potential nerve tissue engineering applications. J Mech Behav Biomed Mater, 93: 183–193.
- Murphy SV, Atala A, 2014, 3D bioprinting of tissues and organs. Nat Biotechnol, 32: 773–785. https://doi.org/10.1038/nbt.2958
- Mironov V, Kasyanov V, Markwald RR, 2011, Organ printing: From bioprinter to organ biofabrication line. Curr Opin Biotechnol, 22: 667–673. https://doi.org/10.1016/j.copbio.2011.02.006
- Novoselov KS, Geim AK, Morozov SV, et al., 2004, Electric field effect in atomically thin carbon films. Science, 306: 666–669. https://doi.org/10.1126/science.1102896
- Li X, Cai W, An J, et al., 2009, Large-area synthesis of high-quality and uniform graphene films on copper foils. Science, 324: 1312–1314. https://doi.org/10.1126/science.1171245
- Anurag K, Kumar S, 2021, Synthesis of graphene through electrochemical exfoliation technique in aqueous medium. Mater Today Proc, 44: 2695–2699.
- Saeed M, Alshammari Y, Majeed SA, et al., 2020, Chemical vapour deposition of graphene-Synthesis, characterisation, and applications: A review. Molecules, 25: 3856. https://doi.org/10.3390/molecules25173856
- Mao X, Zhu L, Liu H, et al., 2019, Synthesis of graphene via electrochemical exfoliation in different electrolytes for direct electrodeposition of a Cu/graphene composite coating. RSC Adv, 9: 35524–35531.
- Koehler FM, Stark WJ, 2013, Organic synthesis on graphene. Acc Chem Res, 46: 2297–2306. https://doi.org/10.1021/ar300125w
- Sun L, 2019, Structure and synthesis of graphene oxide. Chin J Chem Eng, 27: 2251–2260.
- Priyadarsini S, Mohanty S, Mukherjee S, et al., 2018, Graphene and graphene oxide as nanomaterials for medicine and biology application. J Nanostructure Chem, 8: 123–137.
- Filho JS, Venancio E, Silva S, et al., 2020, A thermal method for obtention of 2 to 3 reduced graphene oxide layers from graphene oxide. SN Appl Sci, 2: 1450.
- Zhou Y, Bao Q, Tang LAL, et al., 2009, Hydrothermal dehydration for the “green” reduction of exfoliated graphene oxide to graphene and demonstration of tunable optical limiting properties. Chem Mater, 21: 2950–2956.
- Ai L, Zhang C, Chen Z, 2011, Removal of methylene blue from aqueous solution by a solvothermal-synthesized graphene/ magnetite composite. J Hazard Mater, 192: 1515–1524.
- Cote LJ, Cruz-Silva R, Huang J, 2009, Flash reduction and patterning of graphite oxide and its polymer composite. J Am Chem Soc, 131: 11027–11032. https://doi.org/10.1021/ja902348k
- Akhavan O, 2011, Photocatalytic reduction of graphene oxides hybridized by ZnO nanoparticles in ethanol. Carbon, 49: 11–18.
- Kim YK, Kim MH, Min DH, 2011, Biocompatible reduced graphene oxide prepared by using dextran as a multifunctional reducing agent. Chem Commun, 47: 3195–3197.
- Mohan VB, Brown R, Jayaraman K, et al., 2015, Characterisation of reduced graphene oxide: Effects of reduction variables on electrical conductivity. Mater Sci Eng B, 193: 49–60.
- Karthika V, AlSalhi MS, Devanesan S, et al., 2020, Chitosan overlaid Fe3O4/rGO nanocomposite for targeted drug delivery, imaging, and biomedical applications. Sci Rep, 10: 18912.
- Shin SR, Zihlmann C, Akbari M, et al., 2016, Reduced graphene oxide‐gelMA hybrid hydrogels as scaffolds for cardiac tissue engineering. Small, 12: 3677–3689.
- Reiner-Rozman C, Kotlowski C, Knoll W, 2016, Electronic biosensing with functionalized rGO FETs. Biosensors (Basel), 6: 17. https://doi.org/10.3390/bios6020017
- Favron A, Gaufrès E, Fossard F, et al., 2015, Photooxidation and quantum confinement effects in exfoliated black phosphorus. Nat Mater, 14: 826–832. https://doi.org/10.1038/nmat4299
- Castellanos-Gomez A, Vicarelli L, Prada E, et al., 2014, Isolation and characterization of few-layer black phosphorus. 2D Mater, 1: 025001.
- Gobre VV, Tkatchenko A, 2013, Scaling laws for van der Waals interactions in nanostructured materials. Nat Commun, 4: 2341. https://doi.org/10.1038/ncomms3341
- Ling X, Wang H, Huang S, et al., 2015, The renaissance of black phosphorus. Proc Natl Acad Sci U S A, 112: 4523–4530. https://doi.org/10.1073/pnas.1416581112
- Li L, Yu Y, Ye GJ, et al., 2014, Black phosphorus field-effect transistors. Nat Nanotechnol, 9: 372–377. https://doi.org/10.1038/nnano.2014.35
- Engel M, Steiner M, Avouris P, 2014, Black phosphorus photodetector for multispectral, high-resolution imaging. Nano Lett, 14: 6414–6417. https://doi.org/10.1021/nl502928y
- Batmunkh M, Bat‐Erdene M, Shapter JG, 2018, Black phosphorus: Synthesis and application for solar cells. Adv Energy Mater, 8: 1701832.
- Qiu M, Sun Z, Sang D, et al., 2017, Current progress in black phosphorus materials and their applications in electrochemical energy storage. Nanoscale, 9: 13384–13403.
- Cho SY, Lee Y, Koh HJ, et al., 2016, Superior chemical sensing performance of black phosphorus: comparison with MoS2 and graphene. Adv Mater, 28: 7020–7028. https://doi.org/10.1002/adma.201601167
- Chen W, Ouyang J, Liu H, et al., 2017, Black phosphorus nanosheet‐based drug delivery system for synergistic photodynamic/photothermal/chemotherapy of cancer. Adv Mater, 29: 1603864. https://doi.org/10.1002/adma.201603864
- Li Y, Liu Z, Hou Y, et al., 2017, Multifunctional nanoplatform based on black phosphorus quantum dots for bioimaging and photodynamic/photothermal synergistic cancer therapy. ACS Appl Mater Interfaces, 9: 25098–25106.
- Ghadiri M, Chrzanowski W, Rohanizadeh R, 2014, Antibiotic eluting clay mineral (Laponite®) for wound healing application: An in vitro study. J Mater Sci Mater Med, 25: 2513–2526. https://doi.org/10.1007/s10856-014-5272-7
- Wu Z, Huang X, Li YC, et al., 2018, Novel chitosan films with laponite immobilized Ag nanoparticles for active food packaging. Carbohydr Polym, 199: 210–218. https://doi.org/10.1016/j.carbpol.2018.07.030
- Fatnassi M, Es-Souni M, 2015, Nanoscale phase separation in laponite-polypyrrole nanocomposites. Application to electrodes for energy storage. RSC Adv, 5: 21550–21557.
- Kiaee G, Dimitrakakis N, Sharifzadeh S, et al., 2022, Laponite‐based nanomaterials for drug delivery. Adv Healthc Mater, 11: e2102054. https://doi.org/10.1002/adhm.202102054
- Mongondry P, Tassin JF, Nicolai T, 2005, Revised state diagram of Laponite dispersions. J Colloid Interface Sci, 283: 397–405.
- Jatav S, Joshi YM, 2014, Chemical stability of Laponite in aqueous media. Appl Clay Sci, 97: 72–77.
- Mohanty RP, Joshi YM, 2016, Chemical stability phase diagram of aqueous Laponite dispersions. Appl Clay Sci, 119: 243–248.
- Thompson DW, Butterworth JT, 1992, The nature of laponite and its aqueous dispersions. J Colloid Interface Sci, 151: 236–243.
- Dávila JL, d’Ávila MA, 2017, Laponite as a rheology modifier of alginate solutions: Physical gelation and aging evolution. Carbohydr Polym, 157: 1–8.
- Kästner U, 2001, The impact of rheological modifiers on water-borne coatings. Colloids and Surfaces A Physicochem Eng Aspects, 183: 805–821.
- Bryant SJ, Calabrese V, Da Silva MA, et al., 2021, Rheological modification of partially oxidised cellulose nanofibril gels with inorganic clays. PLos One, 16: e0252660.
- Li X, Liu A, Ye R, et al., 2015, Fabrication of gelatin-laponite composite films: Effect of the concentration of laponite on physical properties and the freshness of meat during storage. Food Hydrocoll, 44: 390–398.
- Guo R, Wang J, Zhang S, et al., 2020, Multifunctional cross-linked polymer-Laponite nanocomposite binder for lithium-sulfur batteries. Chem Eng J, 388: 124316.
- Carvalho A, Mestre A, Pires J, et al., 2006, Granular activated carbons from powdered samples using clays as binders for the adsorption of organic vapours. Microporous Mesoporous Mater, 93: 226–231.
- Ahmad HM, Iqbal T, Al Harthi MA, et al., 2021, Synergistic effect of polymer and nanoparticles on shale hydration and swelling performance of drilling fluids. J Pet Sci Eng, 205: 108763.
- Huang X, Lv K, Sun J, et al., 2019, Enhancement of thermal stability of drilling fluid using laponite nanoparticles under extreme temperature conditions. Mater Lett, 248: 146–149.
- Wu Y, Guo R, Wen S, et al., 2014, Folic acid-modified laponite nanodisks for targeted anticancer drug delivery. J Mater Chem B, 2: 7410–7418.
- Wang G, Maciel D, Wu Y, et al., 2014, Amphiphilic polymer-mediated formation of laponite-based nanohybrids with robust stability and pH sensitivity for anticancer drug delivery. ACS Appl Mater Interfaces, 6: 16687–16695.
- Yu D, Ma M, Liu Z, et al., 2020, MOF-encapsulated nanozyme enhanced siRNA combo: Control neural stem cell differentiation and ameliorate cognitive impairments in Alzheimer’s disease model. Biomaterials, 255: 120160. https://doi.org/10.1016/j.biomaterials.2020.120160
- Khongkow M, Yata T, Boonrungsiman S, et al., 2019, Surface modification of gold nanoparticles with neuron-targeted exosome for enhanced blood–brain barrier penetration. Sci Rep, 9: 8278. https://doi.org/10.1038/s41598-019-44569-6
- Lavoie-Cardinal F, Salesse C, Bergeron É, et al., 2016. Gold nanoparticle-assisted all optical localized stimulation and monitoring of Ca2+ signaling in neurons. Sci Rep, 6: 20619. https://doi.org/10.1038/srep20619
- Wang S, Qiu J, Guo,W, et al., 2017. A nanostructured molybdenum disulfide film for promoting neural stem cell neuronal differentiation: Toward a nerve tissue‐engineered 3D scaffold. Adv Biosyst, 1: e1600042. https://doi.org/10.1002/adbi.201600042
- Goldman EB, Zak A, Tenne R, et al., 2015, Biocompatibility of tungsten disulfide inorganic nanotubes and fullerene-like nanoparticles with salivary gland cells. Tissue Eng Part A, 21: 1013–1023. https://doi.org/10.1089/ten.TEA.2014.0163
- Wang QH, Kalantar-Zadeh K, Kis A, et al., 2012, Electronics and optoelectronics of two-dimensional transition metal dichalcogenides. Nat Nanotechnol, 7: 699–712.
- Stavrou M, Stathis A, Papadakis I, et al., 2022, Silicon nanosheets: An emerging 2D photonic material with a large transient nonlinear optical response beyond graphene. Nanomaterials, 12: 90.
- Ma L, Song X, Yu Y, et al., 2021, Two‐dimensional silicene/silicon nanosheets: An emerging silicon‐composed nanostructure in biomedicine. Adv Mater, 33: 2008226.
- Song SJ, Raja IS, Lee YB, et al., 2019, Comparison of cytotoxicity of black phosphorus nanosheets in different types of fibroblasts. Biomater Res, 23: 23. https://doi.org/10.1186/s40824-019-0174-x
- Kumar S, Chatterjee K, 2016, Comprehensive review on the use of graphene-based substrates for regenerative medicine and biomedical devices. ACS Appl Mater Interfaces, 8: 26431–26457. https://doi.org/10.1021/acsami.6b09801
- Zhang Y, Ali SF, Dervishi E, et al., 2010, Cytotoxicity effects of graphene and single-wall carbon nanotubes in neural phaeochromocytoma-derived PC12 cells. ACS Nano, 4: 3181–3186. https://doi.org/10.1021/nn1007176
- Lv M, Zhang Y, Liang L, et al., 2012, Effect of graphene oxide on undifferentiated and retinoic acid-differentiated SH-SY5Y cells line. Nanoscale, 4: 3861–3866.
- Kang Y, Liu J, Wu J, et al., 2017, Graphene oxide and reduced graphene oxide induced neural pheochromocytoma-derived PC12 cell lines apoptosis and cell cycle alterations via the ERK signaling pathways. Int J Nanomed, 12: 5501–5510. https://doi.org/10.2147/IJN.S141032
- Chen W, Ouyang J, Yi X, et al., 2018, Black phosphorus nanosheets as a neuroprotective nanomedicine for neurodegenerative disorder therapy. Adv Mater, 30: 1703458. https://doi.org/10.1002/adma.201703458
- Yang K, Wan J, Zhang S, et al., 2011, In vivo pharmacokinetics, long-term biodistribution, and toxicology of PEGylated graphene in mice. ACS Nano, 5: 516–522. https://doi.org/10.1021/nn1024303
- Liu X, Miller AL, Park S, et al., 2017, Functionalized carbon nanotube and graphene oxide embedded electrically conductive hydrogel synergistically stimulates nerve cell differentiation. ACS Appl Mater Interfaces, 9: 14677–14690. https://doi.org/10.1021/acsami.7b02072
- Kang MS, Song SJ, Cha JH, et al., 2020, Increased neuritogenesis on ternary nanofiber matrices of PLCL and laminin decorated with black phosphorus. J Ind Eng Chem, 92: 226–235.
- Portioli C, Bussy C, Mazza M, et al., 2020, Intracerebral injection of graphene oxide nanosheets mitigates microglial activation without inducing acute neurotoxicity: A pilot comparison to other nanomaterials. Small, 16: 2004029.
- Basina G, Diamantopoulos G, Devlin E, et al., 2022, LAPONITE® nanodisk-“decorated” Fe3O4 nanoparticles: A biocompatible nano-hybrid with ultrafast magnetic hyperthermia and MRI contrast agent ability. J Mater Chem B, 10: 4935–4943.
- Zhu W, Harris BT, Zhang LG, 2016, Gelatin Methacrylamide Hydrogel with Graphene Nanoplatelets for Neural Cell-laden 3D Bioprinting. In: 38th Annual International Conference of the IEEE Engineering in Medicine and Biology Society (EMBC), August 16-20, 2016, Institute of Electrical and Electronics Engineers, Piscataway NJ. p.4185–4188.
- Jessen KR, Mirsky R, 1999, Schwann cells and their precursors emerge as major regulators of nerve development. Trends Neurosci, 22: 402–410.
- Stierli S, Imperatore V, Lloyd AC, 2019, Schwann cell plasticity‐roles in tissue homeostasis, regeneration, and disease. Glia, 67: 2203–2215. https://doi.org/10.1002/glia.23643
- Gu X, Ding F, Yang Y, et al., 2011, Construction of tissue engineered nerve grafts and their application in peripheral nerve regeneration. Prog Neurobiol, 93: 204–230. https://doi.org/10.1016/j.pneurobio.2010.11.002
- Uz M, Donta M, Mededovic M, et al., 2019, Development of gelatin and graphene-based nerve regeneration conduits using three-dimensional (3D) printing strategies for electrical transdifferentiation of mesenchymal stem cells. Ind Eng Chem Res, 58: 7421–7427.
- Reina G, González-Domínguez JM, Criado A, et al., 2017, Promises, facts and challenges for graphene in biomedical applications. Chem Soc Rev, 46: 4400–4416.
- Huang Q, Cai Y, Zhang X, et al., 2021, Aligned graphene mesh-supported double network natural hydrogel conduit loaded with netrin-1 for peripheral nerve regeneration. ACS Appl Mater Interfaces, 13: 112–122.
- Martino G, Pluchino S, 2006, The therapeutic potential of neural stem cells. Nat Rev Neurosci, 7: 395–406. https://doi.org/10.1038/nrn1908
- Leventhal A, Chen G, Negro A, et al., 2012, The benefits and risks of stem cell technology. Oral Dis, 18: 217–222. 91. Gonzalez R, Lee JP, Snyder EY, 2009, Neural stem cells for central nervous system repair. Essentials of Stem Cell Biology. United States: Academic Press. p.485–496.
- Guo BF, Dong MM, 2009, Application of neural stem cells in tissue-engineered artificial nerve. Otolaryngol Head Neck Surg, 140: 159–164. https://doi.org/10.1016/j.otohns.2008.10.039
- Gasparotto M, Bellet P, Scapin G, et al., 2022, 3D printed graphene-PLA scaffolds promote cell alignment and differentiation. Int J Mol Sci, 23: 1736.
- Jakus AE, Secor EB, Rutz AL, et al., 2015, Three-dimensional printing of high-content graphene scaffolds for electronic and biomedical applications. ACS Nano, 9: 4636–4648. https://doi.org/10.1021/acsnano.5b01179
- Guo F, Creighton M, Chen Y, et al., 2014, Porous structures in stacked, crumpled and pillared graphene-based 3D materials. Carbon N Y, 66: 476–484. https://doi.org/10.1016/j.carbon.2013.09.024
- Mundinamani S, 2020, Large area, multilayer graphene films as a flexible electronic material. ACS Omega, 5: 17479–17485.
- Kang ES, Kim H, Han Y, et al., 2021, Enhancing osteogenesis of adipose-derived mesenchymal stem cells using gold nanostructure/peptide-nanopatterned graphene oxide. Colloids Surf B Biointerfaces, 204: 111807.
- Long X, Duan L, Weng W, et al., 2021, Light-induced osteogenic differentiation of BMSCs with graphene/ TiO2 composite coating on Ti implant. Colloids Surf B Biointerfaces, 207: 111996.
- Lee SM, Kim JH, Ahn JH, 2015, Graphene as a flexible electronic material: Mechanical limitations by defect formation and efforts to overcome. Mater Today, 18: 336–344.
- Li P, Cao K, Jiang C, et al., 2019, In situ tensile fracturing of multilayer graphene nanosheets for their in-plane mechanical properties. Nanotechnology, 30: 475708.
- Qian Y, Zhao X, Han Q, et al., 2018, An integrated multi-layer 3D-fabrication of PDA/RGD coated graphene loaded PCL nanoscaffold for peripheral nerve restoration. Nat Commun, 9: 323. https://doi.org/10.1038/s41467-017-02598-7
- Huang CT, Shrestha LK, Ariga K, et al., 2017, A graphene– polyurethane composite hydrogel as a potential bioink for 3D bioprinting and differentiation of neural stem cells. J Mater Chem B, 5: 8854–8864.
- Siqueira PR, Souza JP, Estevão BM, et al., 2022, Concentration-and time-dependence toxicity of graphene oxide (GO) and reduced graphene oxide (rGO) nanosheets upon zebrafish liver cell line. Aquat Toxicol, 248: 106199. https://doi.org/10.1016/j.aquatox.2022.106199
- Vijayavenkataraman S, Thaharah S, Zhang S, et al., 2019, 3D‐printed PCL/rGO conductive scaffolds for peripheral nerve injury repair. Artif Organs, 43: 515–523. https://doi.org/10.1111/aor.13360
- Qing H, Ji Y, Li W, et al., 2019, Microfluidic printing of three-dimensional graphene electroactive microfibrous scaffolds. ACS Appl Mater Interfaces, 12: 2049–2058. https://doi.org/10.1021/acsami.9b17948
- Zhang X, Zhang H, Zhang Y, et al., 2023, 3D printed reduced graphene oxide-GelMA hybrid hydrogel scaffolds for potential neuralized bone regeneration. J Mater Chem B, 11: 1288–1301. https://doi.org/10.1039/d2tb01979e
- Xu C, Xu Y, Yang M, et al., 2020, Black‐phosphorus‐incorporated hydrogel as a conductive and biodegradable platform for the enhancement of the neural differentiation of mesenchymal stem cells. Adv Funct Mater, 30: 2000177.
- Simon JD, Peles DN, 2010, The red and the black. Acc Chem Res, 43: 1452–1460. https://doi.org/10.1021/ar100079y
- Tondera C, Akbar TF, Thomas AK, et al., 2019, Highly conductive, stretchable, and cell‐adhesive hydrogel by nanoclay doping. Small, 15: e1901406. https://doi.org/10.1002/smll.201901406
- Ou L, Song B, Liang H, et al., 2016, Toxicity of graphene-family nanoparticles: A general review of the origins and mechanisms. Part Fibre Toxicol, 13: 57.
- Fadeel B, Bussy C, Merino S, et al., 2018, Safety assessment of graphene-based materials: Focus on human health and the environment. ACS Nano, 12: 10582–10620.
- Rhazouani A, Gamrani H, El Achaby M, et al., 2021, Synthesis and toxicity of graphene oxide nanoparticles: A literature review of in vitro and in vivo studies. Biomed Res Int, 2021: 5518999. https://doi.org/10.1155/2021/5518999
- Fatima J, Shah AN, Tahir MB, et al., 2022, Tunable 2D nanomaterials; their key roles and mechanisms in water purification and monitoring. Front Environ Sci, 10: 766743.
- Murphy SV, De Coppi P, Atala A, 2020, Opportunities and challenges of translational 3D bioprinting. Nat Biomed Eng, 4: 370–380. https://doi.org/10.1038/s41551-019-0471-7
- Sawosz E, Jaworski S, Kutwin M, et al., 2014, Toxicity of pristine graphene in experiments in a chicken embryo model. Int J Nanomed, 9: 3913. https://doi.org/10.2147/IJN.S65633
- Jaworski S, Sawosz E, Kutwin M, et al., 2015, In vitro and in vivo effects of graphene oxide and reduced graphene oxide on glioblastoma. Int J Nanomed, 10: 1585–1596. https://doi.org/10.2147/IJN.S77591
- Jin L, Hu P, Wang Y, et al., 2020, Fast‐acting black‐phosphorus‐assisted depression therapy with low toxicity. Adv Mater, 32: e1906050. https://doi.org/10.1002/adma.201906050