Recent advances in antioxidant nanomedicines
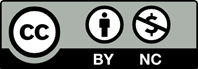
Oxidative stress is a common pathogenic factor for various human diseases. Therefore, mitigating or even eliminating oxidative stress by scavenging excessive reactive oxygen species (ROS) at pathological sites is of great importance in disease treatments. Although several molecular antioxidants have been used in clinics for treating oxidative stress-related diseases, their efficacies are still less satisfactory. Due to the advances in chemistry and nanotechnology, various antioxidant nanomedicines with diversified morphologies, structures, and compositions have been developed to trigger antioxidation reactions in lesion regions, mitigating oxidative stress in cells and regulating the inflammatory microenvironments, finally resulting in disease treatment. In this review, recent progresses achieved in this field are summarized and discussed in a comprehensive manner, with an emphasis on the underlying chemical mechanisms by which antioxidant nanomedicines scavenge ROS in response to the specific microenvironments of pathological regions. It is highly expected that a better understanding on the unique chemistry of antioxidant nanomedicines will facilitate the generation of more feasible antioxidant strategies for the treatments of oxidative stress-associated diseases.
- Finkel T, Holbrook NJ. Oxidants, oxidative stress and the biology of ageing. Nature. 2000;408(6809):239-247. doi: 10.1038/35041687
- Cai H, Harrison DG. Endothelial dysfunction in cardiovascular diseases: The role of oxidant stress. Circ Res. 2000;87(10):840-844. doi: 10.1161/01.res.87.10.840
- Phull AR, Nasir B, Haq IU, Kim SJ. Oxidative stress, consequences and ROS mediated cellular signaling in rheumatoid arthritis. Chem Biol Interact. 2018;281:121-136. doi: 10.1016/j.cbi.2017.12.024
- Barnham KJ, Masters CL, Bush AI. Neurodegenerative diseases and oxidative stress. Nat Rev Drug Discov. 2004;3(3):205-214. doi: 10.1038/nrd1330
- Ratliff BB, Abdulmahdi W, Pawar R, Wolin MS. Oxidant mechanisms in renal injury and disease. Antioxid Redox Signal. 2016;25(3):119-146. doi: 10.1089/ars.2016.6665
- Fiocchi C. Inflammatory bowel disease: Etiology and pathogenesis. Gastroenterology. 1998;115(1):182-205. doi: 10.1016/s0016-5085(98)70381-6
- Lambeth JD. NOX enzymes and the biology of reactive oxygen. Nat Rev Immunol. 2004;4(3):181-189. doi: 10.1038/nri1312
- Winterbourn CC. Reconciling the chemistry and biology of reactive oxygen species. Nat Chem Biol. 2008;4(5):278-286. doi: 10.1038/nchembio.85
- Wu G, Fang YZ, Yang S, Lupton JR, Turner ND. Glutathione metabolism and its implications for health. J Nutr. 2004;134(3):489-492. doi: 10.1093/jn/134.3.489
- Mates JM, Perez-Gomez C, Nunez de Castro I. Antioxidant enzymes and human diseases. Clin Biochem. 1999;32(8):595-603. doi: 10.1016/s0009-9120(99)00075-2
- Pisoschi AM, Pop A. The role of antioxidants in the chemistry of oxidative stress: A review. Eur J Med Chem. 2015;97:55-74. doi: 10.1016/j.ejmech.2015.04.040
- Sies H. Oxidative stress: Oxidants and antioxidants. Exp Physiol. 1997;82(2):291-295. doi: 10.1113/expphysiol.1997.sp004024
- Mittal M, Siddiqui MR, Tran K, Reddy SP, Malik AB. Reactive oxygen species in inflammation and tissue injury. Antioxid Redox Signal. 2014;20(7):1126-1167. doi: 10.1089/ars.2012.5149
- Leyane TS, Jere SW, Houreld NN. Oxidative stress in ageing and chronic degenerative pathologies: Molecular mechanisms involved in counteracting oxidative stress and chronic inflammation. Int J Mol Sci. 2022;23(13):7273. doi: 10.3390/ijms23137273
- Chouchani ET, Pell VR, Gaude E, et al. Ischaemic accumulation of succinate controls reperfusion injury through mitochondrial ROS. Nature. 2014;515(7527):431-435. doi: 10.1038/nature13909
- Giacco F, Brownlee M. Oxidative stress and diabetic complications. Circ Res. 2010;107(9):1058-1070. doi: 10.1161/CIRCRESAHA.110.223545
- Lee SF, Harris R, Stout-Delgado HW. Targeted antioxidants as therapeutics for treatment of pneumonia in the elderly. Transl Res. 2020;220:43-56. doi: 10.1016/j.trsl.2020.03.002
- Galley HF. Oxidative stress and mitochondrial dysfunction in sepsis. Br J Anaesth. 2011;107(1):57-64. doi: 10.1093/bja/aer093
- Cuzzocrea S, Riley DP, Caputi AP, Salvemini D. Antioxidant therapy: A new pharmacological approach in shock, inflammation, and ischemia/reperfusion injury. Pharmacol Rev. 2001;53(1):135-159.
- Forman HJ, Zhang H. Targeting oxidative stress in disease: Promise and limitations of antioxidant therapy. Nat Rev Drug Discov. 2021;20(9):689-709. doi: 10.1038/s41573-021-00233-1
- Firuzi O, Miri R, Tavakkoli M, Saso L. Antioxidant therapy: Current status and future prospects. Curr Med Chem. 2011;18(25):3871-3888. doi: 10.2174/092986711803414368
- Suzuki K. Anti-oxidants for therapeutic use: Why are only a few drugs in clinical use? Adv Drug Deliv Rev. 2009;61(4):287-289. doi: 10.1016/j.addr.2009.03.002
- Salvemini D, Riley DP, Cuzzocrea S. SOD mimetics are coming of age. Nat Rev Drug Discov. 2002;1(5):367-374. doi: 10.1038/nrd796
- Liu YL, Shi JJ. Antioxidative nanomaterials and biomedical applications. Nano Today. 2019;27:146-177. doi: 10.1016/j.nantod.2019.05.008
- Karakoti A, Singh S, Dowding JM, Seal S, Self WT. Redox-active radical scavenging nanomaterials. Chem Soc Rev. 2010;39(11):4422-4432. doi: 10.1039/b919677n
- Ferreira CA, Ni D, Rosenkrans ZT, Cai W. Scavenging of reactive oxygen and nitrogen species with nanomaterials. Nano Res. 2018;11(10):4955-4984. doi: 10.1007/s12274-018-2092-y
- Yang B, Chen Y, Shi J. Nanocatalytic medicine. Adv Mater. 2019;31(39):e1901778. doi: 10.1002/adma.201901778
- Xu C, Qu XG. Cerium oxide nanoparticle: A remarkably versatile rare earth nanomaterial for biological applications. NPG Asia Mater. 2014;6(3):e90. doi: 10.1038/am.2013.88
- Yao J, Cheng Y, Zhou M, et al. ROS scavenging Mn(3) O(4) nanozymes for in vivo anti-inflammation. Chem Sci. 2018;9(11):2927-2933. doi: 10.1039/c7sc05476a
- Wu C, Han X, Feng W, et al. Multi-enzymatic activities of ultrasmall ruthenium oxide for anti-inflammation and neuroprotection. Chem Eng J. 2021;411:128543. doi: 10.1016/j.cej.2021.128543
- Zhang W, Hu S, Yin JJ, et al. Prussian blue nanoparticles as multienzyme mimetics and reactive oxygen species scavengers. J Am Chem Soc. 2016;138(18):5860-5865. doi: 10.1021/jacs.5b12070
- Yang B, Chen Y, Shi J. Reactive oxygen species (ROS)-based nanomedicine. Chem Rev. 2019;119(8):4881-4985. doi: 10.1021/acs.chemrev.8b00626
- Pattni BS, Chupin VV, Torchilin VP. New developments in liposomal drug delivery. Chem Rev. 2015;115(19):10938-10966. doi: 10.1021/acs.chemrev.5b00046
- Yang B, Chen Y, Shi J. Mesoporous silica/organosilica nanoparticles: Synthesis, biological effect and biomedical application. Mater Sci Eng R Rep. 2019;137:66-105. doi: 10.1016/j.mser.2019.01.001
- Lu K, Aung T, Guo N, Weichselbaum R, Lin W. Nanoscale metal-organic frameworks for therapeutic, imaging, and sensing applications. Adv Mater. 2018;30(37):e1707634. doi: 10.1002/adma.201707634
- Mout R, Moyano DF, Rana S, Rotello VM. Surface functionalization of nanoparticles for nanomedicine. Chem Soc Rev. 2012;41(7):2539-2544. doi: 10.1039/c2cs15294k
- Bhattacharjee K, Prasad BLV. Surface functionalization of inorganic nanoparticles with ligands: A necessary step for their utility. Chem Soc Rev. 2023;52(8):2573-2595. doi: 10.1039/d1cs00876e
- Ulbrich K, Hola K, Subr V, Bakandritsos A, Tucek J, Zboril R. Targeted drug delivery with polymers and magnetic nanoparticles: Covalent and noncovalent approaches, release control, and clinical studies. Chem Rev. 2016;116(9):5338-5431. doi: 10.1021/acs.chemrev.5b00589
- King RE, Bomser JA, Min DB. Bioactivity of resveratrol. Compr Rev Food Sci Food Saf. 2006;5(3):65-70. doi: 10.1111/j.1541-4337.2006.00001.x
- Esatbeyoglu T, Huebbe P, Ernst IM, Chin D, Wagner AE, Rimbach G. Curcumin--from molecule to biological function. Angew Chem Int Ed Engl. 2012;51(22):5308-5332. doi: 10.1002/anie.201107724
- Hao Y, Song K, Tan X, et al. Reactive oxygen species-responsive polypeptide drug delivery system targeted activated hepatic stellate cells to ameliorate liver fibrosis. ACS Nano. 2022;16(12):20739-20757. doi: 10.1021/acsnano.2c07796
- Pu HL, Chiang WL, Maiti B, et al. Nanoparticles with dual responses to oxidative stress and reduced ph for drug release and anti-inflammatory applications. ACS Nano. 2014;8(2):1213-1221. doi: 10.1021/nn4058787
- Yang G, Phua SZF, Bindra AK, Zhao Y. Degradability and clearance of inorganic nanoparticles for biomedical applications. Adv Mater. 2019;31(10):e1805730. doi: 10.1002/adma.201805730
- Croissant JG, Fatieiev Y, Khashab NM. Degradability and clearance of silicon, organosilica, silsesquioxane, silica mixed oxide, and mesoporous silica nanoparticles. Adv Mater. 2017;29(9):1604634. doi: 10.1002/adma.201604634
- He F, Liu Z, Xu J, et al. Black phosphorus nanosheets suppress oxidative damage of stem cells for improved neurological recovery. Chem Eng J. 2023;451:138737. doi: 10.1016/j.cej.2022.138737
- Bao X, Zhao J, Sun J, Hu M, Yang X. Polydopamine nanoparticles as efficient scavengers for reactive oxygen species in periodontal disease. ACS Nano. 2018;12(9):8882-8892. doi: 10.1021/acsnano.8b04022
- Nelson BC, Johnson ME, Walker ML, Riley KR, Sims CM. Antioxidant cerium oxide nanoparticles in biology and medicine. Antioxidants (Basel). 2016;5(2):15. doi: 10.3390/antiox5020015
- Qin Z, Li Y, Gu N. Progress in applications of Prussian blue nanoparticles in biomedicine. Adv Healthc Mater. 2018;7(20):e1800347. doi: 10.1002/adhm.201800347
- Cheng Y, Cheng C, Yao J, et al. Mn3O4 nanozyme for inflammatory bowel disease therapy. Adv Ther. 2021;4(9):2100081. doi: 10.1002/adtp.202100081
- Korsvik C, Patil S, Seal S, Self WT. Superoxide dismutase mimetic properties exhibited by vacancy engineered ceria nanoparticles. Chem Commun (Camb). 2007;(10):1056- 1058. doi: 10.1039/b615134e
- Pirmohamed T, Dowding JM, Singh S, et al. Nanoceria exhibit redox state-dependent catalase mimetic activity. Chem Commun (Camb). 2010;46(16):2736-2738. doi: 10.1039/b922024k
- Kim YG, Lee Y, Lee N, Soh M, Kim D, Hyeon T. Ceria-based therapeutic antioxidants for biomedical applications. Adv Mater. 2023;35(n/a):e2210819. doi: 10.1002/adma.202210819
- Murray CJ, Lopez AD. Global mortality, disability, and the contribution of risk factors: Global Burden of Disease Study. Lancet. 1997;349(9063):1436-1442. doi: 10.1016/S0140-6736(96)07495-8
- Izzo C, Vitillo P, Di Pietro P, et al. The role of oxidative stress in cardiovascular aging and cardiovascular diseases. Life (Basel). 2021;11(1):60. doi: 10.3390/life11010060
- Reed GW, Rossi JE, Cannon CP. Acute myocardial infarction. Lancet. 2017;389(10065):197-210. doi: 10.1016/S0140-6736(16)30677-8
- Chen YR, Zweier JL. Cardiac mitochondria and reactive oxygen species generation. Circ Res. 2014;114(3):524-537. doi: 10.1161/CIRCRESAHA.114.300559
- Nishikido T, Oyama J, Shiraki A, Komoda H, Node K. Deletion of apoptosis inhibitor of macrophage (AIM)/ CD5L attenuates the inflammatory response and infarct size in acute myocardial infarction. J Am Heart Assoc. 2016;5(4):e002863. doi: 10.1161/JAHA.115.002863
- Frangogiannis NG. The inflammatory response in myocardial injury, repair, and remodelling. Nat Rev Cardiol. 2014;11(5):255-265. doi: 10.1038/nrcardio.2014.28
- Rodrigo R, Prieto JC, Castillo R. Cardioprotection against ischaemia/reperfusion by vitamins C and E plus n-3 fatty acids: Molecular mechanisms and potential clinical applications. Clin Sci (Lond). 2013;124(1):1-15. doi: 10.1042/CS20110663
- Roubille F, Lacampagne A. New drug avenues for cardioprotection in patients with acute myocardial infarction. Am J Cardiovasc Drugs. 2014;14(1):73-77. doi: 10.1007/s40256-013-0049-9
- Hardy N, Viola HM, Johnstone VP, et al. Nanoparticle-mediated dual delivery of an antioxidant and a peptide against the L-Type Ca2+ channel enables simultaneous reduction of cardiac ischemia-reperfusion injury. ACS Nano. 2015;9(1):279-289. doi: 10.1021/nn5061404
- Guo J, Yang Z, Lu Y, et al. An antioxidant system through conjugating superoxide dismutase onto metal-organic framework for cardiac repair. Bioact Mater. 2022;10:56-67. doi: 10.1016/j.bioactmat.2021.08.019
- Cavallini C, Vitiello G, Adinolfi B, et al. Melanin and melanin-like hybrid materials in regenerative medicine. Nanomaterials (Basel). 2020;10(8):1518. doi: 10.3390/nano10081518
- Zhou J, Liu W, Zhao X, et al. Natural melanin/alginate hydrogels achieve cardiac repair through ROS scavenging and macrophage polarization. Adv Sci (Weinh). 2021;8(20):e2100505. doi: 10.1002/advs.202100505
- D’Ischia M, Napolitano A, Pezzella A, Meredith P, Sarna T. Chemical and structural diversity in eumelanins: Unexplored bio-optoelectronic materials. Angew Chem Int Ed Engl. 2009;48(22):3914-3921. doi: 10.1002/anie.200803786
- Mo XH, Xiang HJ, Wei L, et al. Nature-inspired allomelanin nanomedicine alleviates cardiac ischemia/reperfusion injury via scavenging free radicals and ameliorating myocardial microenvironment. Nano Today. 2022;46:101589. doi: 10.1016/j.nantod.2022.101589
- Hao T, Li J, Yao F, et al. Injectable fullerenol/alginate hydrogel for suppression of oxidative stress damage in brown adipose-derived stem cells and cardiac repair. ACS Nano. 2017;11(6):5474-5488. doi: 10.1021/acsnano.7b00221
- Im GB, Kim YG, Yoo TY, et al. Ceria nanoparticles as copper chaperones that activate SOD1 for synergistic antioxidant therapy to treat ischemic vascular diseases. Adv Mater. 2023;35(16):e2208989. doi: 10.1002/adma.202208989
- Pagliari F, Mandoli C, Forte G, et al. Cerium oxide nanoparticles protect cardiac progenitor cells from oxidative stress. ACS Nano. 2012;6(5):3767-3775. doi: 10.1021/nn2048069
- Blum RH, Carter SK. Adriamycin. A new anticancer drug with significant clinical activity. Ann Intern Med. 1974;80(2):249-259. doi: 10.7326/0003-4819-80-2-249
- Singal PK, Iliskovic N. Doxorubicin-induced cardiomyopathy. N Engl J Med. 1998;339(13):900-905. doi: 10.1056/NEJM199809243391307
- Minotti G, Menna P, Salvatorelli E, Cairo G, Gianni L. Anthracyclines: Molecular advances and pharmacologic developments in antitumor activity and cardiotoxicity. Pharmacol Rev. 2004;56(2):185-229. doi: 10.1124/pr.56.2.6
- Fang X, Wang H, Han D, et al. Ferroptosis as a target for protection against cardiomyopathy. Proc Natl Acad Sci U S A. 2019;116(7):2672-2680. doi: 10.1073/pnas.1821022116
- Peer D, Karp JM, Hong S, Farokhzad OC, Margalit R, Langer R. Nanocarriers as an emerging platform for cancer therapy. Nat Nanotechnol. 2007;2(12):751-760. doi: 10.1038/nnano.2007.387
- Tacar O, Sriamornsak P, Dass CR. Doxorubicin: An update on anticancer molecular action, toxicity and novel drug delivery systems. J Pharm Pharmacol. 2013;65(2):157-170. doi: 10.1111/j.2042-7158.2012.01567.x
- Park S, Yoon J, Bae S, et al. Therapeutic use of H2O2-responsive anti-oxidant polymer nanoparticles for doxorubicin-induced cardiomyopathy. Biomaterials. 2014;35(22):5944-5953. doi: 10.1016/j.biomaterials.2014.03.084
- Huo M, Tang Z, Wang L, et al. Magnesium hexacyanoferrate nanocatalysts attenuate chemodrug-induced cardiotoxicity through an anti-apoptosis mechanism driven by modulation of ferrous iron. Nat Commun. 2022;13(1):7778. doi: 10.1038/s41467-022-35503-y
- Lusis AJ. Atherosclerosis. Nature. 2000;407(6801):233-241. doi: 10.1038/35025203
- Libby P, Ridker PM, Hansson GK. Progress and challenges in translating the biology of atherosclerosis. Nature. 2011;473(7347):317-325. doi: 10.1038/nature10146
- Weber C, Noels H. Atherosclerosis: Current pathogenesis and therapeutic options. Nat Med. 2011;17(11):1410-1422. doi: 10.1038/nm.2538
- Tyrrell DJ, Goldstein DR. Ageing and atherosclerosis: Vascular intrinsic and extrinsic factors and potential role of IL-6. Nat Rev Cardiol. 2021;18(1):58-68. doi: 10.1038/s41569-020-0431-7
- Koelwyn GJ, Corr EM, Erbay E, Moore KJ. Regulation of macrophage immunometabolism in atherosclerosis. Nat Immunol. 2018;19(6):526-537. doi: 10.1038/s41590-018-0113-3
- Thomas SR, Witting PK, Stocker R. A role for reduced coenzyme Q in atherosclerosis? Biofactors. 1999;9(2-4):207-224. doi: 10.1002/biof.5520090216
- Salonen RM, Nyyssonen K, Kaikkonen J, et al. Six-year effect of combined vitamin C and E supplementation on atherosclerotic progression: The antioxidant supplementation in atherosclerosis prevention (ASAP) study. Circulation. 2003;107(7):947-953. doi: 10.1161/01.cir.0000050626.25057.51
- Zimetbaum P, Eder H, Frishman W. Probucol: Pharmacology and clinical application. J Clin Pharmacol. 1990;30(1):3-9. doi: 10.1002/j.1552-4604.1990.tb03431.x
- Yamakoshi J, Kataoka S, Koga T, Ariga T. Proanthocyanidin-rich extract from grape seeds attenuates the development of aortic atherosclerosis in cholesterol-fed rabbits. Atherosclerosis. 1999;142(1):139-149. doi: 10.1016/s0021-9150(98)00230-5
- Kim CH, Mitchell JB, Bursill CA, et al. The nitroxide radical TEMPOL prevents obesity, hyperlipidaemia, elevation of inflammatory cytokines, and modulates atherosclerotic plaque composition in apoE-/- mice. Atherosclerosis. 2015;240(1):234-241. doi: 10.1016/j.atherosclerosis.2015.03.012
- Sugamura K, Keaney JF Jr. Reactive oxygen species in cardiovascular disease. Free Radic Biol Med. 2011;51(5):978-992. doi: 10.1016/j.freeradbiomed.2011.05.004
- Lobatto ME, Fuster V, Fayad ZA, Mulder WJ. Perspectives and opportunities for nanomedicine in the management of atherosclerosis. Nat Rev Drug Discov. 2011;10(11):835-852. doi: 10.1038/nrd3578
- Chen J, Zhang X, Millican R, et al. Recent advances in nanomaterials for therapy and diagnosis for atherosclerosis. Adv Drug Deliv Rev. 2021;170:142-199. doi: 10.1016/j.addr.2021.01.005
- Chen W, Schilperoort M, Cao Y, Shi J, Tabas I, Tao W. Macrophage-targeted nanomedicine for the diagnosis and treatment of atherosclerosis. Nat Rev Cardiol. 2022;19(4):228-249. doi: 10.1038/s41569-021-00629-x
- Maruf A, Wang Y, Yin T, et al. Atherosclerosis treatment with stimuli-responsive nanoagents: Recent advances and future perspectives. Adv Healthc Mater. 2019;8(11):e1900036. doi: 10.1002/adhm.201900036
- Lobatto ME, Calcagno C, Millon A, et al. Atherosclerotic plaque targeting mechanism of long-circulating nanoparticles established by multimodal imaging. ACS Nano. 2015;9(2):1837-1847. doi: 10.1021/nn506750r
- Smith BR. Nanotherapeutics for cardiovascular disease. Nat Rev Cardiol. 2021;18(9):617-618. doi: 10.1038/s41569-021-00594-5
- Wang Y, Li L, Zhao W, et al. Targeted therapy of atherosclerosis by a broad-spectrum reactive oxygen species scavenging nanoparticle with intrinsic anti-inflammatory activity. ACS Nano. 2018;12(9):8943-8960. doi: 10.1021/acsnano.8b02037
- Wang S, Zhang J, Li W, et al. Hyaluronic acid-guided assembly of ceria nanozymes as plaque-targeting ROS scavengers for anti-atherosclerotic therapy. Carbohydr Polym. 2022;296:119940. doi: 10.1016/j.carbpol.2022.119940
- Parayath NN, Parikh A, Amiji MM. Repolarization of tumor-associated macrophages in a genetically engineered nonsmall cell lung cancer model by intraperitoneal administration of hyaluronic acid-based nanoparticles encapsulating MicroRNA-125b. Nano Lett. 2018;18(6):3571-3579. doi: 10.1021/acs.nanolett.8b00689
- Mu D, Li J, Qi Y, et al. Hyaluronic acid-coated polymeric micelles with hydrogen peroxide scavenging to encapsulate statins for alleviating atherosclerosis. J Nanobiotechnology. 2020;18(1):179. doi: 10.1186/s12951-020-00744-w
- Chen X, Dai C, Hu R, Yu L, Chen Y, Zhang B. Engineering ROS-scavenging Prussian blue nanozymes for efficient atherosclerosis nanotherapy. J Mater Chem B. 2023;11(9):1881-1890. doi: 10.1039/d2tb02661a
- Yang Q, Jiang H, Wang Y, et al. Plaque macrophage-targeting nanosystems with cooperative co-regulation of ROS and TRAF6 for stabilization of atherosclerotic plaques. Adv Funct Mater. 2023;33:2301053. doi: 10.1002/adfm.202301053
- Mizushima N, Komatsu M. Autophagy: Renovation of cells and tissues. Cell. 2011;147(4):728-741. doi: 10.1016/j.cell.2011.10.026
- Rabinowitz JD, White E. Autophagy and metabolism. Science. 2010;330(6009):1344-1348. doi: 10.1126/science.1193497
- Klionsky DJ, Emr SD. Autophagy as a regulated pathway of cellular degradation. Science. 2000;290(5497):1717-1721. doi: 10.1126/science.290.5497.1717
- Guo FX, Wu Q, Li P, et al. The role of the LncRNA-FA2H-2- MLKL pathway in atherosclerosis by regulation of autophagy flux and inflammation through mTOR-dependent signaling. Cell Death Differ. 2019;26(9):1670-1687. doi: 10.1038/s41418-018-0235-z
- Bravo-San Pedro JM, Kroemer G, Galluzzi L. Autophagy and mitophagy in cardiovascular disease. Circ Res. 2017;120(11):1812-1824. doi: 10.1161/CIRCRESAHA.117.311082
- Wu Z, Zhou M, Tang X, et al. Carrier-free trehalose-based nanomotors targeting macrophages in inflammatory plaque for treatment of atherosclerosis. ACS Nano. 2022;16(3):3808-3820. doi: 10.1021/acsnano.1c08391
- Segawa K, Nagata S. An apoptotic “eat me” signal: Phosphatidylserine exposure. Trends Cell Biol. 2015;25(11):639-650. doi: 10.1016/j.tcb.2015.08.003
- Wang PG, Xian M, Tang X, et al. Nitric oxide donors: Chemical activities and biological applications. Chem Rev. 2002;102(4):1091-1134. doi: 10.1021/cr000040l
- Carpenter AW, Schoenfisch MH. Nitric oxide release: Part II. Therapeutic applications. Chem Soc Rev. 2012;41(10):3742-3752. doi: 10.1039/c2cs15273h
- Sciarretta S, Yee D, Nagarajan N, et al. Trehalose-induced activation of autophagy improves cardiac remodeling after myocardial infarction. J Am Coll Cardiol. 2018;71(18):1999-2010. doi: 10.1016/j.jacc.2018.02.066
- Hu R, Dai C, Dong C, et al. Living macrophage-delivered tetrapod PdH nanoenzyme for targeted atherosclerosis management by ROS scavenging, hydrogen anti-inflammation, and autophagy activation. ACS Nano. 2022;16(10):15959-15976. doi: 10.1021/acsnano.2c03422
- Ouriel K. Peripheral arterial disease. Lancet. 2001;358(9289):1257-1264. doi: 10.1016/S0140-6736(01)06351-6
- Criqui MH, Aboyans V. Epidemiology of peripheral artery disease. Circ Res. 2015;116(9):1509-1526. doi: 10.1161/CIRCRESAHA.116.303849
- Koutakis P, Ismaeel A, Farmer P, et al. Oxidative stress and antioxidant treatment in patients with peripheral artery disease. Physiol Rep. 2018;6(7):e13650. doi: 10.14814/phy2.13650
- Brevetti G, Giugliano G, Brevetti L, Hiatt WR. Inflammation in peripheral artery disease. Circulation. 2010;122(18):1862-1875. doi: 10.1161/CIRCULATIONAHA.109.918417
- Violi F, Loffredo L, Mancini A, Marcoccia A. Antioxidants in peripheral arterial disease. Curr Drug Targets. 2003;4(8):651-655. doi: 10.2174/1389450033490786
- Giannopoulos G, Angelidis C, Vogiatzi G, Cleman MW, Deftereos S. Antioxidant treatment in peripheral artery disease: The rationale is there, but what about clinical results? Curr Opin Pharmacol. 2018;39:53-59. doi: 10.1016/j.coph.2018.01.008
- Jung E, Lee J, Jeong L, et al. Stimulus-activatable echogenic maltodextrin nanoparticles as nanotheranostic agents for peripheral arterial disease. Biomaterials. 2019;192:282-291. doi: 10.1016/j.biomaterials.2018.11.022
- Guo J, Ping Y, Ejima H, et al. Engineering multifunctional capsules through the assembly of metal-phenolic networks. Angew Chem Int Ed Engl. 2014;53(22):5546-5551. doi: 10.1002/anie.201311136
- Rahim MA, Kristufek SL, Pan S, Richardson JJ, Caruso F. Phenolic building blocks for the assembly of functional materials. Angew Chem Int Ed Engl. 2019;58(7):1904-1927. doi: 10.1002/anie.201807804
- Zhou J, Lin Z, Ju Y, Rahim MA, Richardson JJ, Caruso F. Polyphenol-mediated assembly for particle engineering. Acc Chem Res. 2020;53(7):1269-1278. doi: 10.1021/acs.accounts.0c00150
- Duan J, Chen Z, Liang X, et al. Construction and application of therapeutic metal-polyphenol capsule for peripheral artery disease. Biomaterials. 2020;255:120199. doi: 10.1016/j.biomaterials.2020.120199
- Mokra D, Joskova M, Mokry J. Therapeutic effects of green tea polyphenol (‒)-epigallocatechin-3-gallate (EGCG) in relation to molecular pathways controlling inflammation, oxidative stress, and apoptosis. Int J Mol Sci. 2022;24(1):340. doi: 10.3390/ijms24010340
- Mandinov L, Mandinova A, Kyurkchiev S, et al. Copper chelation represses the vascular response to injury. Proc Natl Acad Sci U S A. 2003;100(11):6700-6705. doi: 10.1073/pnas.1231994100
- Isselbacher EM. Thoracic and abdominal aortic aneurysms. Circulation. 2005;111(6):816-828. doi: 10.1161/01.CIR.0000154569.08857.7A
- Kent KC. Clinical practice. Abdominal aortic aneurysms. N Engl J Med. 2014;371(22):2101-2108. doi: 10.1056/NEJMcp1401430
- Schanzer A, Oderich GS. Management of abdominal aortic aneurysms. N Engl J Med. 2021;385(18):1690-1698. doi: 10.1056/NEJMcp2108504
- Chaikof EL, Dalman RL, Eskandari MK, et al. The society for vascular surgery practice guidelines on the care of patients with an abdominal aortic aneurysm. J Vasc Surg. 2018;67(1):2-77.e2. doi: 10.1016/j.jvs.2017.10.044
- McCormick ML, Gavrila D, Weintraub NL. Role of oxidative stress in the pathogenesis of abdominal aortic aneurysms. Arterioscler Thromb Vasc Biol. 2007;27(3):461-469. doi: 10.1161/01.ATV.0000257552.94483.14
- Miller FJ Jr., Sharp WJ, Fang X, Oberley LW, Oberley TD, Weintraub NL. Oxidative stress in human abdominal aortic aneurysms: A potential mediator of aneurysmal remodeling. Arterioscler Thromb Vasc Biol. 2002;22(4):560-565. doi: 10.1161/01.atv.0000013778.72404.30
- Shimizu K, Mitchell RN, Libby P. Inflammation and cellular immune responses in abdominal aortic aneurysms. Arterioscler Thromb Vasc Biol. 2006;26(5):987-994. doi: 10.1161/01.ATV.0000214999.12921.4f
- Gao J, Cao H, Hu G, et al. The mechanism and therapy of aortic aneurysms. Signal Transduct Target Ther. 2023;8(1):55. doi: 10.1038/s41392-023-01325-7
- Lin W, Hu K, Li C, et al. A multi-bioactive nanomicelle-based “one stone for multiple birds” strategy for precision therapy of abdominal aortic aneurysms. Adv Mater. 2022;34(44):e2204455. doi: 10.1002/adma.202204455
- Wilcox CS, Pearlman A. Chemistry and antihypertensive effects of tempol and other nitroxides. Pharmacol Rev. 2008;60(4):418-469. doi: 10.1124/pr.108.000240
- LeMaire SA, Russell L. Epidemiology of thoracic aortic dissection. Nat Rev Cardiol. 2011;8(2):103-113. doi: 10.1038/nrcardio.2010.187
- Nienaber CA, Clough RE. Management of acute aortic dissection. Lancet. 2015;385(9970):800-811. doi: 10.1016/S0140-6736(14)61005-9
- Fan LM, Douglas G, Bendall JK, et al. Endothelial cell-specific reactive oxygen species production increases susceptibility to aortic dissection. Circulation. 2014;129(25):2661-2672. doi: 10.1161/CIRCULATIONAHA.113.005062
- Furie B, Furie BC. Mechanisms of thrombus formation. N Engl J Med. 2008;359(9):938-949. doi: 10.1056/NEJMra0801082
- Gailani D, Renne T. The intrinsic pathway of coagulation: A target for treating thromboembolic disease? J Thromb Haemost. 2007;5(6):1106-1112. doi: 10.1111/j.1538-7836.2007.02446.x
- Wendelboe AM, Raskob GE. Global burden of thrombosis: Epidemiologic aspects. Circ Res. 2016;118(9):1340-1347. doi: 10.1161/CIRCRESAHA.115.306841
- Absar S, Gupta N, Nahar K, Ahsan F. Engineering of plasminogen activators for targeting to thrombus and heightening thrombolytic efficacy. J Thromb Haemost. 2015;13(9):1545-1556. doi: 10.1111/jth.13033
- Gaffney PJ. Tissue plasminogen activator for thrombolytic therapy: Expectation versus reality. J R Soc Med. 1992;85(11):692-698. doi: 10.1177/014107689208501112
- Shi K, Zou M, Jia DM, et al. tPA mobilizes immune cells that exacerbate hemorrhagic transformation in stroke. Circ Res. 2021;128(1):62-75. doi: 10.1161/CIRCRESAHA.120.317596
- Greer IA. Clinical practice. Pregnancy complicated by venous thrombosis. N Engl J Med. 2015;373(6):540-547. doi: 10.1056/NEJMcp1407434
- Su M, Dai Q, Chen C, Zeng Y, Chu C, Liu G. Nano-medicine for thrombosis: A precise diagnosis and treatment strategy. Nanomicro Lett. 2020;12(1):96. doi: 10.1007/s40820-020-00434-0
- Zenych A, Fournier L, Chauvierre C. Nanomedicine progress in thrombolytic therapy. Biomaterials. 2020;258:120297. doi: 10.1016/j.biomaterials.2020.120297
- Cheng J, Zhang S, Li C, et al. Functionally integrating nanoparticles alleviate deep vein thrombosis in pregnancy and rescue intrauterine growth restriction. Nat Commun. 2022;13(1):7166. doi: 10.1038/s41467-022-34878-2
- Xu L, Luo Y, Du Q, et al. Magnetic response combined with bioactive ion therapy: A RONS-scavenging theranostic nanoplatform for thrombolysis and renal ischemia-reperfusion injury. ACS Nano. 2023;17(6):5695-5712. doi: 10.1021/acsnano.2c12091
- Chandler AB, Chapman I, Erhardt LR, et al. Coronary thrombosis in myocardial infarction. Report of a workshop on the role of coronary thrombosis in the pathogenesis of acute myocardial infarction. Am J Cardiol. 1974;34(7):823-833. doi: 10.1016/0002-9149(74)90703-6
- Guo X, Hong T, Zang J, et al. Thrombus-specific/ responsive biomimetic nanomedicine for spatiotemporal thrombolysis and alleviation of myocardial ischemia/ reperfusion injury. J Nanobiotechnology. 2022;20(1):531. doi: 10.1186/s12951-022-01686-1
- Firestein GS. Evolving concepts of rheumatoid arthritis. Nature. 2003;423(6937):356-361. doi: 10.1038/nature01661
- Li RQ, Ma Y, Hong J, Ding Y. Nanoengineered therapy aiming at the etiology of rheumatoid arthritis. Nano Today. 2022;42:101367. doi: 10.1016/j.nantod.2021.101367
- Tardito S, Martinelli G, Soldano S, et al. Macrophage M1/ M2 polarization and rheumatoid arthritis: A systematic review. Autoimmun Rev. 2019;18(11):102397. doi: 10.1016/j.autrev.2019.102397
- Zhu Y, Zhao TJ, Liu M, et al. Rheumatoid arthritis microenvironment insights into treatment effect of nanomaterials. Nano Today. 2022;42:101358. doi: 10.1016/j.nantod.2021.101358
- Aletaha D, Smolen JS. Diagnosis and management of rheumatoid arthritis: A review. JAMA. 2018;320(13):1360-1372. doi: 10.1001/jama.2018.13103
- Yuan F, Quan LD, Cui L, Goldring SR, Wang D. Development of macromolecular prodrug for rheumatoid arthritis. Adv Drug Deliv Rev. 2012;64(12):1205-1219. doi: 10.1016/j.addr.2012.03.006
- Wang S, Lv J, Meng S, Tang J, Nie L. Recent advances in nanotheranostics for treat-to-target of rheumatoid arthritis. Adv Healthc Mater. 2020;9(6):e1901541. doi: 10.1002/adhm.201901541
- Ma Y, Lu Z, Jia B, et al. DNA origami as a nanomedicine for targeted rheumatoid arthritis therapy through reactive oxygen species and nitric oxide scavenging. ACS Nano. 2022;16(8):12520-12531. doi: 10.1021/acsnano.2c03991
- Xu C, Wang S, Wang H, et al. Magnesium-based micromotors as hydrogen generators for precise rheumatoid arthritis therapy. Nano Lett. 2021;21(5):1982-1991. doi: 10.1021/acs.nanolett.0c04438
- Hirst SM, Karakoti AS, Tyler RD, Sriranganathan N, Seal S, Reilly CM. Anti-inflammatory properties of cerium oxide nanoparticles. Small. 2009;5(24):2848-2856. doi: 10.1002/smll.200901048
- Jeong HG, Cha BG, Kang DW, et al. Ceria nanoparticles fabricated with 6-aminohexanoic acid that overcome systemic inflammatory response syndrome. Adv Healthc Mater. 2019;8(9):e1801548. doi: 10.1002/adhm.201801548
- Kim J, Kim HY, Song SY, et al. Synergistic oxygen generation and reactive oxygen species scavenging by manganese ferrite/ceria co-decorated nanoparticles for rheumatoid arthritis treatment. ACS Nano. 2019;13(3):3206-3217. doi: 10.1021/acsnano.8b08785
- Roubenoff R, Freeman LM, Smith DE, Abad LW, Dinarello CA, Kehayias JJ. Adjuvant arthritis as a model of inflammatory cachexia. Arthritis Rheum. 1997;40(3):534-539. doi: 10.1002/art.1780400320
- Xu C, Jiang Y, Wang H, et al. Arthritic microenvironment actuated nanomotors for active rheumatoid arthritis therapy. Adv Sci (Weinh). 2023;10(4):e2204881. doi: 10.1002/advs.202204881
- Guo L, Zhong S, Liu P, Guo M, Ding J, Zhou W. Radicals scavenging MOFs enabling targeting delivery of siRNA for rheumatoid arthritis therapy. Small. 2022;18(27):e2202604. doi: 10.1002/smll.202202604
- Yang B, Yao H, Yang J, Chen C, Shi J. Construction of a two-dimensional artificial antioxidase for nanocatalytic rheumatoid arthritis treatment. Nat Commun. 2022;13(1):1988. doi: 10.1038/s41467-022-29735-1
- Batinic-Haberle I, Tovmasyan A, Spasojevic I. An educational overview of the chemistry, biochemistry and therapeutic aspects of Mn porphyrins--from superoxide dismutation to H2O2-driven pathways. Redox Biol. 2015;5:43-65. doi: 10.1016/j.redox.2015.01.017
- Tovmasyan A, Sheng H, Weitner T, et al. Design, mechanism of action, bioavailability and therapeutic effects of mn porphyrin-based redox modulators. Med Princ Pract. 2013;22(2):103-130. doi: 10.1159/000341715
- Batinic-Haberle I, Tovmasyan A, Spasojevic I. Mn porphyrin-based redox-active drugs: Differential effects as cancer therapeutics and protectors of normal tissue against oxidative injury. Antioxid Redox Signal. 2018;29(16):1691-1724. doi: 10.1089/ars.2017.7453
- Yang B, Shi J. Defect engineering of mesoporous silica nanoparticles for biomedical applications. Accounts Mater Res. 2021;2(8):581-593. doi: 10.1021/accountsmr.1c00055
- Garstang SV, Stitik TP. Osteoarthritis: Epidemiology, risk factors, and pathophysiology. Am J Phys Med Rehabil. 2006;85(11 Suppl):S2-11; quiz S12-S14. doi: 10.1097/01.phm.0000245568.69434.1a
- Sharma L, Kapoor D, Issa S. Epidemiology of osteoarthritis: An update. Curr Opin Rheumatol. 2006;18(2):147-156. doi: 10.1097/01.bor.0000209426.84775.f8
- Altman RD. Osteoarthritis. Differentiation from rheumatoid arthritis, causes of pain, treatment. Postgrad Med. 1990;87(3):66-72, 77-78. doi: 10.1080/00325481.1990.11704582
- Lepetsos P, Papavassiliou AG. ROS/oxidative stress signaling in osteoarthritis. Biochim Biophys Acta. 2016;1862(4):576-591. doi: 10.1016/j.bbadis.2016.01.003
- McAlindon TE, Bannuru RR, Sullivan MC, et al. OARSI guidelines for the non-surgical management of knee osteoarthritis. Osteoarthritis Cartilage. 2014;22(3):363-88. doi: 10.1016/j.joca.2014.01.003
- Cheuk YC, Fu SC, Mok SW, Ho KK, Hung LK, Chan KM. Intra-articular injection of an antioxidant formulation did not improve structural degeneration in a rat model of post-traumatic osteoarthritis. J Orthop Translat. 2017;8:25-31. doi: 10.1016/j.jot.2016.08.001
- Elmali N, Esenkaya I, Harma A, Ertem K, Turkoz Y, Mizrak B. Effect of resveratrol in experimental osteoarthritis in rabbits. Inflamm Res. 2005;54(4):158-162. doi: 10.1007/s00011-004-1341-6
- Natarajan V, Madhan B, Tiku ML. Intra-articular injections of polyphenols protect articular cartilage from inflammation-induced degradation: Suggesting a potential role in cartilage therapeutics. PLoS One. 2015;10(6):e0127165. doi: 10.1371/journal.pone.0127165
- Howard MD, Hood ED, Zern B, Shuvaev VV, Grosser T, Muzykantov VR. Nanocarriers for vascular delivery of anti-inflammatory agents. Annu Rev Pharmacol Toxicol. 2014;54:205-226. doi: 10.1146/annurev-pharmtox-011613-140002
- Gui T, Luo L, Chhay B, et al. Superoxide dismutase-loaded porous polymersomes as highly efficient antioxidant nanoparticles targeting synovium for osteoarthritis therapy. Biomaterials. 2022;283:121437. doi: 10.1016/j.biomaterials.2022.121437
- Zhou F, Mei J, Yang S, et al. Modified ZIF-8 nanoparticles attenuate osteoarthritis by reprogramming the metabolic pathway of synovial macrophages. ACS Appl Mater Interfaces. 2020;12(2):2009-2022. doi: 10.1021/acsami.9b16327
- Zhao C, Chen J, Ye J, et al. Structural transformative antioxidants for dual-responsive anti-inflammatory delivery and photoacoustic inflammation imaging. Angew Chem Int Ed Engl. 2021;60(26):14458-14466. doi: 10.1002/anie.202100873
- Yang B, Yin J, Chen Y, et al. 2D-black-phosphorus-reinforced 3D-printed scaffolds: A stepwise countermeasure for osteosarcoma. Adv Mater. 2018;30(10):1705611. doi: 10.1002/adma.201705611
- Lu H, Wei J, Liu K, et al. Radical-scavenging and subchondral bone-regenerating nanomedicine for osteoarthritis treatment. ACS Nano. 2023;17(6):6131-6146. doi: 10.1021/acsnano.3c01789
- Kumar S, Adjei IM, Brown SB, Liseth O, Sharma B. Manganese dioxide nanoparticles protect cartilage from inflammation-induced oxidative stress. Biomaterials. 2019;224:119467. doi: 10.1016/j.biomaterials.2019.119467
- Klibanski A, Adams-Campbell L, Bassford T, et al. Osteoporosis prevention, diagnosis, and therapy. JAMA. 2001;285(6):785-795.
- Rachner TD, Khosla S, Hofbauer LC. Osteoporosis: Now and the future. Lancet. 2011;377(9773):1276-1287. doi: 10.1016/S0140-6736(10)62349-5
- Manolagas SC, Jilka RL. Bone marrow, cytokines, and bone remodeling. Emerging insights into the pathophysiology of osteoporosis. N Engl J Med. 1995;332(5):305-311. doi: 10.1056/NEJM199502023320506
- Bax BE, Alam AS, Banerji B, et al. Stimulation of osteoclastic bone resorption by hydrogen peroxide. Biochem Biophys Res Commun. 1992;183(3):1153-1158. doi: 10.1016/s0006-291x(05)80311-0
- Agidigbi TS, Kim C. Reactive oxygen species in osteoclast differentiation and possible pharmaceutical targets of ROS-mediated osteoclast diseases. Int J Mol Sci. 2019;20(14):3576. doi: 10.3390/ijms20143576
- Teitelbaum SL. Bone resorption by osteoclasts. Science. 2000;289(5484):1504-1508. doi: 10.1126/science.289.5484.1504
- Rodan GA, Martin TJ. Therapeutic approaches to bone diseases. Science. 2000;289(5484):1508-1514. doi: 10.1126/science.289.5484.1508
- Reid IR, Billington EO. Drug therapy for osteoporosis in older adults. Lancet. 2022;399(10329):1080-1092. doi: 10.1016/S0140-6736(21)02646-5
- Ye C, Zhang W, Zhao Y, et al. Prussian blue nanozyme normalizes microenvironment to delay osteoporosis. Adv Healthc Mater. 2022;11(19):e2200787. doi: 10.1002/adhm.202200787
- Tian Q, Wang W, Cao L, et al. Multifaceted catalytic ROS-scavenging via electronic modulated metal oxides for regulating stem cell fate. Adv Mater. 2022;34(43):e2207275. doi: 10.1002/adma.202207275
- Wu Z, Sun Y, Mu S, et al. Manganese-based antioxidase-inspired biocatalysts with axial Mn-N5 Sites and 2D d-pi-conjugated networks for rescuing stem cell fate. Angew Chem Int Ed Engl. 2023;62:e202302329. doi: 10.1002/anie.202302329
- Terstappen GC, Meyer AH, Bell RD, Zhang W. Strategies for delivering therapeutics across the blood-brain barrier. Nat Rev Drug Discov. 2021;20(5):362-383. doi: 10.1038/s41573-021-00139-y
- Furtado D, Bjornmalm M, Ayton S, Bush AI, Kempe K, Caruso F. Overcoming the blood-brain barrier: The role of nanomaterials in treating neurological diseases. Adv Mater. 2018;30(46):e1801362. doi: 10.1002/adma.201801362
- Tang W, Fan W, Lau J, Deng L, Shen Z, Chen X. Emerging blood-brain-barrier-crossing nanotechnology for brain cancer theranostics. Chem Soc Rev. 2019;48(11):2967-3014. doi: 10.1039/c8cs00805a
- Krol S, Macrez R, Docagne F, et al. Therapeutic benefits from nanoparticles: the potential significance of nanoscience in diseases with compromise to the blood brain barrier. Chem Rev. 2013;113(3):1877-1903. doi: 10.1021/cr200472g
- Butterfield DA, Halliwell B. Oxidative stress, dysfunctional glucose metabolism and Alzheimer disease. Nat Rev Neurosci. 2019;20(3):148-160. doi: 10.1038/s41583-019-0132-6
- Blennow K, de Leon MJ, Zetterberg H. Alzheimer’s disease. Lancet. 2006;368(9533):387-403. doi: 10.1016/S0140-6736(06)69113-7
- Tonnies E, Trushina E. Oxidative stress, synaptic dysfunction, and Alzheimer’s disease. J Alzheimers Dis. 2017;57(4):1105-1121. doi: 10.3233/JAD-161088
- Liu Y, Nguyen M, Robert A, Meunier B. Metal ions in Alzheimer’s disease: A key role or not? Acc Chem Res. 2019;52(7):2026-2035. doi: 10.1021/acs.accounts.9b00248
- Gaggelli E, Kozlowski H, Valensin D, Valensin G. Copper homeostasis and neurodegenerative disorders (Alzheimer’s, prion, and Parkinson’s diseases and amyotrophic lateral sclerosis). Chem Rev. 2006;106(6):1995-2044. doi: 10.1021/cr040410w
- Hardy J, Allsop D. Amyloid deposition as the central event in the aetiology of Alzheimer’s disease. Trends Pharmacol Sci. 1991;12(10):383-388. doi: 10.1016/0165-6147(91)90609-v
- Kepp KP. Bioinorganic chemistry of Alzheimer’s disease. Chem Rev. 2012;112(10):5193-5239. doi: 10.1021/cr300009x
- Huang X, Atwood CS, Hartshorn MA, et al. The A beta peptide of Alzheimer’s disease directly produces hydrogen peroxide through metal ion reduction. Biochemistry. 1999;38(24):7609-7616. doi: 10.1021/bi990438f
- Huang X, Cuajungco MP, Atwood CS, et al. Cu(II) potentiation of Alzheimer abeta neurotoxicity. Correlation with cell-free hydrogen peroxide production and metal reduction. J Biol Chem. 1999;274(52):37111-3716. doi: 10.1074/jbc.274.52.37111
- Mondragon-Rodriguez S, Perry G, Zhu X, Moreira PI, Acevedo-Aquino MC, Williams S. Phosphorylation of tau protein as the link between oxidative stress, mitochondrial dysfunction, and connectivity failure: Implications for Alzheimer’s disease. Oxid Med Cell Longev. 2013;2013:940603. doi: 10.1155/2013/940603
- Reisberg B, Doody R, Stoffler A, et al. Memantine in moderate-to-severe Alzheimer’s disease. N Engl J Med. 2003;348(14):1333-1341. doi: 10.1056/NEJMoa013128
- Zhang L, Zhao P, Yue C, et al. Sustained release of bioactive hydrogen by Pd hydride nanoparticles overcomes Alzheimer’s disease. Biomaterials. 2019;197:393-404. doi: 10.1016/j.biomaterials.2019.01.037
- Yao J, Irwin RW, Zhao L, Nilsen J, Hamilton RT, Brinton RD. Mitochondrial bioenergetic deficit precedes Alzheimer’s pathology in female mouse model of Alzheimer’s disease. Proc Natl Acad Sci U S A. 2009;106(34):14670-14675. doi: 10.1073/pnas.0903563106
- Qian K, Bao X, Li Y, et al. Cholinergic neuron targeting nanosystem delivering hybrid peptide for combinatorial mitochondrial therapy in Alzheimer’s disease. ACS Nano. 2022;16(7):11455-11472. doi: 10.1021/acsnano.2c05795
- Kwon HJ, Cha MY, Kim D, et al. Mitochondria-targeting ceria nanoparticles as antioxidants for Alzheimer’s disease. ACS Nano. 2016;10(2):2860-2870. doi: 10.1021/acsnano.5b08045
- Zeng F, Wu Y, Li X, et al. Custom-made ceria nanoparticles show a neuroprotective effect by modulating phenotypic polarization of the microglia. Angew Chem Int Ed Engl. 2018;57(20):5808-5812. doi: 10.1002/anie.201802309
- Chen Q, Du Y, Zhang K, et al. Tau-targeted multifunctional nanocomposite for combinational therapy of Alzheimer’s disease. ACS Nano. 2018;12(2):1321-1338. doi: 10.1021/acsnano.7b07625