High inhibitory activity of flavonoids from Sophora japonica L. flower buds against α-amylase and α-glucosidase: A mechanistic insight
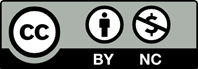
In this work, a method was developed to screen compounds with enzyme activity inhibition in vitro using chromatographic analysis combined with activity difference analysis (CAADA). The flower buds of Sophora japonica L. (FBSJ) were found to contain abundant flavonoids. These flavonoids were then screened for their high inhibitory activity against α-amylase and α-glucosidase using fingerprint and activity difference analysis. Consistent conclusions were drawn from multiple techniques, including the reported technique, IC50 data, and CAADA. The inhibitory mechanism was further analyzed through enzyme inhibition kinetics, circular dichroism, fluorescence spectrometry, molecular docking, and molecular dynamics (MD). Among the six flavonoid components studied, quercetin was found to act as a competitive inhibitor against α-amylase, while kaempferol showed a mixed manner of inhibition against α-glucosidase. Molecular docking and MD simulations demonstrated that quercetin and kaempferol have higher binding energies and bound more tightly to their targets. In general, flavonols exhibited higher inhibitory activity than their corresponding flavonol glycosides against both α-amylase and α-glucosidase. Quercetin and kaempferol in FBSJ showed potential as inhibitors of α-amylase and α-glucosidase. This study not only presented a novel method for screening compounds with high activity but also provided a theoretical basis for studying the application and mechanism of flavonoids against α-amylase and α-glucosidase in FBSJ.
- Zheng Y, Tian J, Yang W, et al. Inhibition mechanism of ferulic acid against α-amylase and α-glucosidase. Food Chem. 2020;317:126346. doi: 10.1016/j.foodchem.2020.126346
- Hussain T, Tan B, Murtaza G, et al. Flavonoids and type 2 diabetes: Evidence of efficacy in clinical and animal studies and delivery strategies to enhance their therapeutic efficacy. Pharmacol Res. 2020;152:104629. doi: 10.1016/j.phrs.2020.104629
- Xu X, Wang P, Wang B, et al. Glucose absorption regulation and mechanism of the compounds in Lilium lancifolium Thunb on Caco-2 cells. Food Chem Toxicol. 2021;149:112010. doi: 10.1016/j.fct.2021.112010
- Cui L, Wang J, Wang M, et al. Chemical composition and glucose uptake effect on 3T3-L1 adipocytes of Ligustrum lucidum Ait. Flowers. Food Sci Hum Well. 2020;9(2):124-129. doi: 10.1016/j.fshw.2020.02.002
- Sabiu S, O’Neill FH, Ashafa AOT. Kinetics of α-amylase and α-glucosidase inhibitory potential of Zea mays Linnaeus (Poaceae), Stigma maydis aqueous extract: An in vitro assessment. J Ethnopharmacol. 2016;183:1-8. doi: 10.1016/j.jep.2016.02.024
- Han S, Luo Y, Hu Z, D. et al. Targeting gut microbiota in type 2 diabetes mellitus: Potential roles of dietary flavonoids. Food Biosci. 2022;45:101500. doi: 10.1016/j.fbio.2021.101500
- Bui TT, Nguyen HT. Ethanolic extract of Sophora japonica flower buds alleviates cognitive deficits induced by scopolamine in mice. Oriental Pharm Exp M. 2017;17(4):337-344. doi: 10.1007/s13596-017-0286-6
- Li J, Gong Y, Li J, et al. In vitro xanthine oxidase inhibitory properties of Flos Sophorae Immaturus and potential mechanisms. Food Biosci. 2022;47:101711. doi: 10.1016/j.fbio.2022.101711
- Liu C, Ma C, Lu J, et al. A rapid method and mechanism to identify the active compounds in Malus micromalus Makino fruit with spectrum-effect relationship, components knock-out and molecular docking technology. Food Chem Toxicol. 2021;150:112086. doi: 10.1016/j.fct.2021.112086
- Li W, Zhang Y, Shi S, et al. Spectrum-effect relationship of antioxidant and tyrosinase activity with Malus pumila flowers by UPLC-MS/MS and component knock-out method. Food Chem Toxicol. 2019;133:110754. doi: 10.1016/j.fct.2019.110754
- Li F, Fu Y, Yang H, et al. The inhibition mechanism of luteolin on peroxidase based on multispectroscopic techniques. Int J Biol Macromol. 2021;166:1072-1081. doi: 10.1016/j.ijbiomac.2020.10.262
- Fu M, Shen W, Gao W, et al. Essential moieties of myricetins, quercetins and catechins for binding and inhibitory activity against alpha-Glucosidase. Bioorg Chem. 2021;115:105235. doi: 10.1016/j.bioorg.2021.105235
- Kazeem MI, Davies TC. Anti-diabetic functional foods as sources of insulin secreting, insulin sensitizing and insulin mimetic agents. J Funct Foods. 2016;20:122-138. doi: 10.1016/j.jff.2015.10.013
- Sansenya S, Payaka A, Mansalai P. Biological activity and inhibition potential against α-glucosidase and α-amylase of 2,4-di-tert-butylphenol from bamboo shoot extract by in vitro and in silico studies. Process Biochem. 2023;126:15-22.
- Xiao Y, Shan X, Wang H, et al. Spectrum-effect relationship between HPLC fingerprint and antioxidant of “San-Bai Decoction” extracts. J Chromatogr B Analyt Technol Biomed Life Sci. 2022;1208:123380. doi: 10.1016/j.jchromb.2022.123380
- Ma TC, Sun Y, Guo LN, et al. Screening potential α-glucosidase inhibitors from Anemarrhena asphodeloides using response surface methodology coupled with grey relational analysis. Chin Herb Med. 2019;11(1):60-69.doi: 10.1016/j.chmed.2018.08.010
- Wu X, Hu M, Hu X, et al. Inhibitory mechanism of epicatechin gallate on α-amylase and α-glucosidase and its combinational effect with acarbose or epigallocatechin gallate. J Mol Liq. 2019;290:111202. doi: 10.1016/j.molliq.2019.111202
- Yang J, Wang X, Zhang C, et al. Comparative study of inhibition mechanisms of structurally different flavonoid compounds on alpha-glucosidase and synergistic effect with acarbose. Food Chem. 2021;347:129056. doi: 10.1016/j.foodchem.2021.129056
- Guo S, Meng J, Wang S, et al. Preparation of magnetic microcapsules of α-amylase and α-glucosidase for dual-target affinity screening of active components from Toona sinensis. Process Biochem. 2023;124:92-99. doi: 10.1016/j.procbio.2022.11.018
- Wang R, Fan R, Meng T, et al. Exploration of the inhibitory mechanisms of trans-polydatin/resveratrol on α-glucosidase by multi-spectroscopic analysis, in silico docking and molecular dynamics simulation. Spectrochim Acta A Mol Biomol Spectrosc. 2023;299:122866. doi: 10.1016/j.saa.2023.122866
- Wang D, Gu X, Fang K, et al. Study on quality control of Zuojin pill by HPLC fingerprint with quantitative analysis of multi-components by single marker method and antioxidant activity analysis. J Pharm Biomed Anal. 2023;225:115075. doi: 10.1016/j.jpba.2022.115075
- Li C, Cui Y, Lu J, et al. Spectrum-effect relationship of immunologic activity of Ganoderma lucidum by UPLC-MS/ MS and component knock-out method. Food Sci Hum Wellness. 2021;10(3):278-288. doi: 10.1016/j.fshw.2021.02.019
- Chen Y, Pan G, Xu W, et al. Spectrum-effect relationship study between HPLC fingerprints and antioxidant activity of Sabia parviflora. J Chromatogr B Analyt Technol Biomed Life Sci. 2020;1140:121970. doi: 10.1016/j.jchromb.2020.121970
- Zhao Y, Wang M, Huang G. Structure-activity relationship and interaction mechanism of nine structurally similar flavonoids and α-amylase. J Funct Foods. 2021;86:104739. doi: 10.1016/j.jff.2021.104739
- Wu H, Lv Y, Wei F, Li C, Ge W, Du W. Comparative analysis of anti-osteoporosis efficacy in Radix Dipsaci before and after processing with salt based on spectrum-effect relationship. J Pharm Biomed. 2022;221:115078. doi: 10.1016/j.jpba.2022.115078
- Yang F, Zou Y, Li C, et al. Discovery of potential hypoglycemic metabolites in Cassiae Semen by coupling UHPLC-QTOF-MS/MS combined plant metabolomics and spectrum-effect relationship analyses. Food Funct. 2022;13(19):10291-10304. doi: 10.1039/d2fo00562j
- Zhang J, Chen T, Li K, et al. Screening active ingredients of rosemary based on spectrum-effect relationships between UPLC fingerprint and vasorelaxant activity using three chemometrics. J Chromatogr B Analyt Technol Biomed Life Sci. 2019;1134-1135:121854. doi: 10.1016/j.jchromb.2019.121854
- Zhang D, Liu X, Yang Z, et al. Interactions between phenols and alkylamides of Sichuan Pepper (Zanthoxylum genus) in alpha-glucosidase inhibition: A structural mechanism analysis. J Agric Food Chem. 2021;69(20):5583-5598. doi: 10.1021/acs.jafc.1c00741
- Aleixandre A, Gil JV, Sineiro J, et al. Understanding phenolic acids inhibition of α-amylase and α-glucosidase and influence of reaction conditions. Food Chem. 2022;372:131231. doi: 10.1016/j.foodchem.2021.131231
- Lim J, Ferruzzi MG, Hamaker BR. Structural requirements of flavonoids for the selective inhibition of alpha-amylase versus alpha-glucosidase. Food Chem. 2022;370:130981. doi: 10.1016/j.foodchem.2021.130981
- Wang S, Li Y, Huang D, et al. The inhibitory mechanism of chlorogenic acid and its acylated derivatives on alpha-amylase and α-glucosidase. Food Chem. 2022;372:131334. doi: 10.1016/j.foodchem.2021.131334
- Ghani U, Nur-e-Alam M, Yousaf M, Ul-Haq Z, Noman OM, Al-Rehaily AJ. Natural flavonoid α-glucosidase inhibitors from Retama raetam: Enzyme inhibition and molecular docking reveal important interactions with the enzyme active site. Bioorg Chem. 2019;87:736-742. doi: 10.1016/j.bioorg.2019.03.079
- Wang Y, Zhang G, Pan J, et al. Novel insights into the inhibitory mechanism of kaempferol on xanthine oxidase. J Agric Food Chem. 2015;63(2):526-534. doi: 10.1021/jf505584m
- Hua F, Zhou P, Wu HY, et al. Inhibition of α-glucosidase and α-amylase by flavonoid glycosides from Lu’an GuaPian tea: Molecular docking and interaction mechanism. Food Funct. 2018;9(8):4173-4183. doi: 10.1039/c8fo00562a
- Wu Y, Han Z, Wen M, et al. Screening of α-glucosidase inhibitors in large-leaf yellow tea by offline bioassay coupled with liquid chromatography tandem mass spectrometry. Food Sci Hum Well. 2022;11(3):627-634. doi: 10.1016/j.fshw.2021.12.019
- Sun L, Chen W, Meng Y, et al. Interactions between polyphenols in thinned young apples and porcine pancreatic α-amylase: Inhibition, detailed kinetics and fluorescence quenching. Food Chem. 2016;208:51-60. doi: 10.1016/j.foodchem.2016.03.093
- Wang S, Xie X, Zhang L, et al. Inhibition mechanism of α-glucosidase inhibitors screened from Artemisia selengensis Turcz root. Ind Crop Prod. 2020;143:111941. doi: 10.1016/j.indcrop.2019.111941
- Wang L, Wang L, Wang T, et al. Comparison of quercetin and rutin inhibitory influence on Tartary buckwheat starch digestion in vitro and their differences in binding sites with the digestive enzyme. Food Chem. 2022;367:130762. doi: 10.1016/j.foodchem.2021.130762
- Yan J, Zhang G, Pan J, Wang Y. α-Glucosidase inhibition by luteolin: Kinetics, interaction and molecular docking. Int J Biol Macromol. 2014;64:213-223. doi: 10.1016/j.ijbiomac.2013.12.007
- Wang Q, Li R, Li N, et al. The antioxidant activities, inhibitory effects, kinetics, and mechanisms of artocarpin and alpha-mangostin on alpha-glucosidase and alpha-amylase. Int J Biol Macromol. 2022;213:880-889. doi: 10.1016/j.ijbiomac.2022.06.017
- Yang Y, Zhang P, Huang Z, et al. Phenolics from Sterculia nobilis Smith pericarp by-products delay carbohydrate digestion by uncompetitively inhibiting α-glucosidase and α-amylase. LWT. 2023;173:114339. doi: 10.1016/j.lwt.2022.114339
- Yu Q, Fan L, Duan Z. Five individual polyphenols as tyrosinase inhibitors: Inhibitory activity, synergistic effect, action mechanism, and molecular docking. Food Chem. 2019;297:124910. doi: 10.1016/j.foodchem.2019.05.184
- Song X, Ni M, Zhang Y, Zhang G, Pan J, Gong D. Comparing the inhibitory abilities of epigallocatechin-3-gallate and gallocatechin gallate against tyrosinase and their combined effects with kojic acid. Food Chem. 2021;349:129172. doi: 10.1016/j.foodchem.2021.129172
- Ding H, Wu X, Pan J, et al. New insights into the inhibition mechanism of betulinic acid on α-glucosidase. J Agric Food Chem. 2018;66(27):7065-7075. doi: 10.1021/acs.jafc.8b02992
- Peng X, Zhang G, Liao Y, et al. Inhibitory kinetics and mechanism of kaempferol on alpha-glucosidase. Food Chem. 2016;190:207-215. doi: 10.1016/j.foodchem.2015.05.088
- Han L, Song J, Yan C, et al. Inhibitory activity and mechanism of calycosin and calycosin-7-O-β-D-glucoside on α-glucosidase: Spectroscopic and molecular docking analyses. Process Biochem. 2022;118:227-235. doi: 10.1016/j.procbio.2022.04.035
- Shen H, Wang J, Ao J, et al. Structure-activity relationships and the underlying mechanism of alpha-amylase inhibition by hyperoside and quercetin: Multi-spectroscopy and molecular docking analyses. Spectrochim Acta A Mol Spectrosc. 2023;285:121797. doi: 10.1016/j.saa.2022.121797
- Li M, Bao X, Zhang X, et al. Exploring the phytochemicals and inhibitory effects against α-glucosidase and dipeptidyl peptidase-IV in Chinese pickled chili pepper: Insights into mechanisms by molecular docking analysis. LWT. 2022;162:113467. doi: 10.1016/j.lwt.2022.113467
- Han L, Fang C, Zhu R, et al. Inhibitory effect of phloretin on alpha-glucosidase: Kinetics, interaction mechanism and molecular docking. Int J Biol Macromol. 2017;95:520-527. doi: 10.1016/j.ijbiomac.2016.11.089
- Zhang J, Ding W, Tang Z, et al. Identification of the effective alpha-amylase inhibitors from Dalbergia odorifera: Virtual screening, spectroscopy, molecular docking, and molecular dynamic simulation. Spectrochim Acta A Mol Biomol Spectrosc. 2022;280:121448. doi: 10.1016/j.saa.2022.121448
- Phong NV, Yang SY, Min BS, et al. Insights into the inhibitory activity and mechanism of action of flavonoids from the stems and branches of Acer mono Maxim. Against α-glucosidase via kinetic analysis, molecular docking, and molecular dynamics simulations. J Mol Struct. 2023;1282:135188. doi: 10.1016/j.molstruc.2023.135188
- Yadav R, Hasan S, Mahato S, et al. Molecular docking, DFT analysis, and dynamics simulation of natural bioactive compounds targeting ACE2 and TMPRSS2 dual binding sites of spike protein of SARS CoV-2. J Mol Liq. 2021;342:116942. doi: 10.1016/j.molliq.2021.116942
- Sharifpour S, Fakhraee S, Behjatmanesh-Ardakani R. Insights into the mechanism of inhibition of phospholipase A2 by resveratrol: An extensive molecular dynamics simulation and binding free energy calculation. J Mol Graph Model. 2020;100:107649. doi: 10.1016/j.jmgm.2020.107649