Recent advances in liver bioengineering and disease modeling using bioprinting
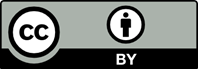
The increase in prevalence of liver diseases and lack of versatile treatment options for end-stage liver diseases stresses the need for novel approaches and advanced technologies for developing physiologically and pathologically relevant models of liver. Liver bioengineering through bioprinting has emerged as a pivotal area of research, aiming to address the critical shortage of donor organs and improve drug testing and disease modeling. Through bioprinting the complex structure, composition and geometry of liver microstructure can be replicated to give rise to liver models that can mimic the healthy or diseased tissue. These capabilities render bioprinted liver models suitable for advancing drug discovery and testing studies, as well as disease modeling. This review paper explores the application of bioprinting technologies in liver tissue engineering, highlighting the progress in the field through exploring materials, cell sources and new current techniques used in bioprinting of liver models. Additionally, the article explores spheroid printing and current preclinical models as well as key challenges and future perspectives. This comprehensive overview aims to provide insights into the current state of liver bioengineering through bioprinting and to identify future directions for research and clinical application.