Treating myocardial infarction with 3D-printed conductive myocardial patch fabricated from granular composite hydrogel
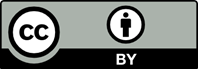
In recent years, engineered conductive myocardial patches have gained increasing attention for their potential in repairing myocardial infarction (MI). However, the traditional fabrication process of these patches often includes the use of toxic conductive monomers and crosslinking agents, along with harsh physical treatments such as low-temperature drying. These elements not only hinder the effective in situ loading of myocardial cells but also limit the efficiency and retention of cell seeding post-fabrication. To address these challenges, we developed an innovative approach using a granular composite hydrogel, comprising myocardial cell-laden microgels integrated with an interstitial conductive matrix, capable of being printed into 3D complex electroactive cardiac patches. We used microfluidic technology to encapsulate cardiomyocytes in microgels. Furthermore, we integrated the conductive polymer poly(3,4-ethylenedioxythiophene):poly(styre ne sulfonate) into a GelMA prepolymer to fabricate a conductive matrix. This matrix was subsequently combined with microgels to form a conductive bioink, which was then utilized for printing a conductive myocardial patch. The results demonstrated that the myocardial cell-laden microgels within the patches maintained robust viability and functionality. Moreover, the patches exhibited electrical conductivity aligned with physiological levels and possessed the requisite mechanical properties for structural support to the infarcted heart. We found that these conductive cell-laden patches significantly improved left ventricular remodeling and heart function post-infarction in a rat model of MI. We believe that the design and engineering of conductive cellular myocardium patches represent a significant advancement in the treatment of MI.

- Bolognese L, Neskovic AN, Parodi G, et al. Left ventricular remodeling after primary coronary angioplasty: patterns of left ventricular dilation and long-term prognostic implications. Circulation. 2002;106(18):2351-2357. doi: 10.1161/01.cir.0000036014.90197.fa
- Ghofrani A, Taghavi L, Khalilivavdareh B, et al. Additive manufacturing and advanced functionalities of cardiac patches: a review. Eur Polym J. 2022;174:111332. doi: 10.1016/j.eurpolymj.2022.111332
- Wang L, Liu Y, Ye G, et al. Injectable and conductive cardiac patches repair infarcted myocardium in rats and minipigs. Nat Biomed Eng. 2021;5(10):1157-1173. doi: 10.1038/s41551-021-00796-9
- Silvestri A, Boffito M, Sartori S, et al. Biomimetic materials and scaffolds for myocardial tissue regeneration. Macromol Biosci. 2013;13(8):984-1019. doi: 10.1002/mabi.201200483
- Kapnisi M, Mansfield C, Marijon C, et al. Auxetic cardiac patches with tunable mechanical and conductive properties toward treating myocardial infarction. Adv Funct Mater. 2018;28(21):1800618. doi: 10.1002/adfm.201800618
- Hu X, Zhang P, Liu J, et al. A self-association cross-linked conductive zwitterionic hydrogel as a myocardial patch for restoring cardiac function. Chem Eng J. 2022; 446:136988. doi: 10.1016/j.cej.2022.136988
- Spencer AR, Primbetova A, Koppes AN, et al. Electroconductive gelatin methacryloyl-PEDOT:PSS composite hydrogels: design, synthesis, and properties. ACS Biomater Sci Eng. 2018;4(5):1558-1567. doi: 10.1021/acsbiomaterials.8b00135
- Yin Q, Zhu P, Liu W, et al. A conductive bioengineered cardiac patch for myocardial infarction treatment by improving tissue electrical integrity. Adv Healthc Mater. 2023;12(1):e2201856. doi: 10.1002/adhm.202201856
- Sun L, Zhu X, Zhang X, et al. Induced cardiomyocytes-integrated conductive microneedle patch for treating myocardial infarction. Chem Eng J. 2021;414:128723. doi: 10.1016/j.cej.2021.128723
- Mehrotra S, Singh RD, Bandyopadhyay A, et al. Engineering microsphere-loaded non-mulberry silk-based 3D bioprinted vascularized cardiac patches with oxygen-releasing and immunomodulatory potential. ACS Appl Mater Interfaces. 2021;13(43):50744-50759. doi: 10.1021/acsami.1c14118
- Shabankhah M, Moghaddaszadeh A, Najmoddin N. 3D printed conductive PCL/GO scaffold immobilized with gelatin/CuO accelerates H9C2 cells attachment and proliferation. Prog Organ Coat. 2024;186:108013. doi: 10.1016/j.porgcoat.2023.108013
- Liang Y, Qiao L, Qiao B, et al. Conductive hydrogels for tissue repair. Chem Sci. 2023;14(12):3091-3116. doi: 10.1039/d3sc00145h
- Tropp J, Collins CP, Xie X, et al. Conducting polymer nanoparticles with intrinsic aqueous dispersibility for conductive hydrogels. Adv Mater. 2024;36(1):e2306691. doi: 10.1002/adma.202306691
- Taghizadeh A, Taghizadeh M, Jouyandeh M, et al. Conductive polymers in water treatment: a review. J Mol Liquids. 2020;312:113447. doi: 10.1016/j.molliq.2020.113447
- Mushtaq AB, Reyaz AR, Aabid HS, et al. PEDOT and PEDOT:PSS conducting polymeric hydrogels: a report on their emerging applications. Synth Metals. 2021; 273:116709. doi: 10.1016/j.synthmet.2021.116709
- Mu L, Dong R, Guo B. Biomaterials‐based cell therapy for myocardial tissue regeneration. Adv Healthc Mater. 2023;12(10):e2202699. doi: 10.1002/adhm.202202699
- Liu W, Zhao N, Yin Q, et al. Injectable hydrogels encapsulating dual-functional Au@Pt core-shell nanoparticles regulate infarcted microenvironments and enhance the therapeutic efficacy of stem cells through antioxidant and electrical integration. ACS Nano. 2023;17(3):2053-2066. doi: 10.1021/acsnano.2c07436
- Ghanta RK, Aghlara-Fotovat S, Pugazenthi A, et al. Immune-modulatory alginate protects mesenchymal stem cells for sustained delivery of reparative factors to ischemic myocardium. Biomater Sci. 2020;8(18):5061-5070. doi: 10.1039/d0bm00855a
- Rosellini E, Cascone MG, Guidi L, et al. Mending a broken heart by biomimetic 3D printed natural biomaterial-based cardiac patches: a review. Front Bioeng Biotechnol. 2023;11:1254739. doi: 10.3389/fbioe.2023.1254739
- Noor N, Shapira A, Edri R, et al. 3D printing of personalized thick and perfusable cardiac patches and hearts. Adv Sci. 2019;6(11):1900344. doi: 10.1002/advs.201970066
- Yin J, Yan M, Wang Y, et al. 3D bioprinting of low-concentration cell-laden gelatin methacrylate (GelMA) bioinks with a two-step cross-linking strategy. ACS Appl Mater Interfaces. 2018;10(8):6849-6857. doi: 10.1021/acsami.7b16059
- Fang Y, Guo Y, Ji M, et al. 3D printing of cell‐laden microgel‐based biphasic bioink with heterogeneous microenvironment for biomedical applications. Adv Funct Mater. 2022;32(13):2109810. doi: 10.1002/adfm.202109810
- Fang Y, Wang C, Liu Z, et al. 3D printed conductive multiscale nerve guidance conduit with hierarchical fibers for peripheral nerve regeneration. Adv Sci (Weinh). 2023;10(12):e2205744. doi: 10.1002/advs.202205744
- Liu W, Heinrich MA, Zhou Y, et al. Extrusion bioprinting of shear‐thinning gelatin methacryloyl bioinks. Adv Healthc Mater. 2017;6(12):1601451. doi: 10.1002/adhm.201601451
- Fang Y, Sun W, Zhang T, et al. Recent advances on bioengineering approaches for fabrication of functional engineered cardiac pumps: a review. Biomaterials. 2022;280:121298. doi: 10.1016/j.biomaterials.2021.12129
- Ostrakhovitch EA, Byers JC, O’Neil KD, et al. Directed differentiation of embryonic P19 cells and neural stem cells into neural lineage on conducting PEDOT–PEG and ITO glass substrates. Arch Biochem Biophys. 2012; 528(1):21-31. doi: 10.1016/j.abb.2012.08.006
- He Y, Li Q, Chen P, et al. A smart adhesive Janus hydrogel for non-invasive cardiac repair and tissue adhesion prevention. Nat Commun. 2022;13(1):7666. doi: 10.1038/s41467-022-35437-5
- Song X, Wang X, Zhang J, et al. A tunable self-healing ionic hydrogel with microscopic homogeneous conductivity as a cardiac patch for myocardial infarction repair. Biomaterials. 2021;273:120811. doi: 10.1016/j.biomaterials.2021.120811
- Bertassoni LE, Cardoso JC, Manoharan V, et al. Direct-write bioprinting of cell-laden methacrylated gelatin hydrogels. Biofabrication. 2014;6(2):24105. doi: 10.1088/1758-5082/6/2/024105
- Theus AS, Ning L, Hwang B, et al. Bioprintability: physiomechanical and biological requirements of materials for 3D bioprinting processes. Polymers (Basel). 2020;12(10):2262. doi: 10.3390/polym12102262
- Li M, Wu H, Yuan Y, et al. Recent fabrications and applications of cardiac patch in myocardial infarction treatment. VIEW. 2022;3(2):20200153. doi: 10.1002/VIW.20200153