Comprehensive insight into 3D bioprinting technology for brain tumor modelling
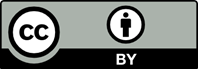
Glioblastoma is one of the most common primary malignant brain tumors with a poor prognosis and high mortality rate. Since only small lipophilic molecules can penetrate the blood–brain barrier, chemotherapy and immunotherapy are limited in their ability to effectively treat brain tumors. 3D bioprinting has the potential to directly model the 3D environment of human pathology and diseases. In many cancers, 3D bioprinting technology closely mimics the tumor microenvironment, making it a promising tool for drug screening and uncovering the mechanisms of cancer initiation and progression. Recent 3D bioprinting technologies have been developed to recreate the dynamic interactions between the tumor and endothelial cells. Brain tumor models using 3D bioprinting technology can reconstruct the biophysical heterogeneity and immune interactions in the brain. These advanced models regulate the organization of tumor structures for preclinical drug testing and reveal the immunological pathways involved in brain tumors. Here, we highlight 3D bioprinting technologies that can replace conventional in vitro models for brain tumor treatment.

- Tang M, Jiang S, Huang X, et al. Integration of 3D bioprinting and multi-algorithm machine learning identified glioma susceptibilities and microenvironment characteristics. Cell Discov. 2024;10(1):39. doi: 10.1038/s41421-024-00650-7
- Yi HG, Jeong YH, Kim Y, et al. A bioprinted human-glioblastoma-on-a-chip for the identification of patient-specific responses to chemoradiotherapy. Nat Biomed Eng. 2019;3(7):509-519. doi: 10.1038/s41551-019-0363-x
- Wang YI, Abaci HE, Shuler ML. Microfluidic blood-brain barrier model provides in vivo‐like barrier properties for drug permeability screening. Biotechnol Bioeng. 2017;114(1):184-194. doi: 10.1002/bit.26045
- Tang M, Xie Q, Gimple RC, et al. Three-dimensional bioprinted glioblastoma microenvironments model cellular dependencies and immune interactions. Cell Res. 2020;30(10):833-853. doi: 10.1038/s41422-020-0338-1
- Silvani G, Basirun C, Wu H, et al. A 3D‐bioprinted vascularized glioblastoma‐on‐a‐chip for studying the impact of simulated microgravity as a novel pre‐clinical approach in brain tumor therapy. Adv Ther. 2021;4(11):2100106. doi: 10.1002/adtp.202100106
- Colopi A, Fuda S, Santi S, et al. Impact of age and gender on glioblastoma onset, progression, and management. Mech Ageing Dev. 2023;211:111801. doi: 10.1016/j.mad.2023.111801
- Lauko A, Lo A, Ahluwalia MS, Lathia JD. Cancer cell heterogeneity & plasticity in glioblastoma and brain tumors. Semin Cancer Biol. 2022;82:162-175. doi: 10.1016/j.semcancer.2021.02.014
- Schaff LR, Mellinghoff IK. Glioblastoma and other primary brain malignancies in adults: a review. JAMA. 2023;329(7):574-587. doi: 10.1001/jama.2023.0023
- Xie Z, Chen M, Lian J, Wang H, Ma J. Glioblastoma-on-a-chip construction and therapeutic applications. Front Oncol. 2023;13:1183059. doi: 10.3389/fonc.2023.1183059
- Angom RS, Nakka NMR, Bhattacharya S. Advances in glioblastoma therapy: an update on current approaches. Brain Sci. 2023;13(11):1536. doi: 10.3390/brainsci13111536
- Sharma P, Aaroe A, Liang J, Puduvalli VK. Tumor microenvironment in glioblastoma: current and emerging concepts. Neurooncol Adv. 2023;5(1):vdad009. doi: 10.1093/noajnl/vdad009
- Pineda E, Domenech M, Hernandez A, Comas S, Balana C. Recurrent glioblastoma: ongoing clinical challenges and future prospects. Onco Targets Ther. 2023;16:71-86. doi: 10.2147/OTT.S366371
- Knowlton S, Onal S, Yu CH, Zhao JJ, Tasoglu S. Bioprinting for cancer research. Trends Biotechnol. 2015;33(9):504-513. doi: 10.1016/j.tibtech.2015.06.007
- Van Norman GA. Limitations of animal studies for predicting toxicity in clinical trials: is it time to rethink our current approach? JACC Basic Transl Sci. 2019;4(7):845-854. doi: 10.1016/j.jacbts.2020.03.010
- Kang Y, Datta P, Shanmughapriya S, Ozbolat IT. 3D bioprinting of tumor models for cancer research. ACS Appl Bio Mater. 2020;3(9):5552-5573. doi: 10.1021/acsabm.0c00791
- Gungor-Ozkerim PS, Inci I, Zhang YS, Khademhosseini A, Dokmeci MR. Bioinks for 3D bioprinting: an overview. Biomater Sci. 2018;6(5):915-946. doi: 10.1039/c7bm00765e
- Prendergast ME, Burdick JA. Computational modeling and experimental characterization of extrusion printing into suspension baths. Adv Healthc Mater. 2022;11(7):e2101679. doi: 10.1002/adhm.202101679
- Ozbek II, Saybasili H, Ulgen KO. Applications of 3D bioprinting technology to brain cells and brain tumor models: special emphasis to glioblastoma. ACS Biomater Sci Eng. 2024;10(5):2616-2635. doi: 10.1021/acsbiomaterials.3c01569
- Qiu B, Bessler N, Figler K, et al. Bioprinting neural systems to model central nervous system diseases. Adv Funct Mater. 2020;30(44):1910250. doi: 10.1002/adfm.201910250
- DePalma TJ, Sivakumar H, Skardal A. Strategies for developing complex multi-component in vitro tumor models: highlights in glioblastoma. Adv Drug Deliv Rev. 2022;180:114067. doi: 10.1016/j.addr.2021.114067
- Jiao W, Shan J, Gong X, et al. GelMA hydrogel: a game-changer in 3D tumor modeling. Mater Today Chem. 2024;38:102111. doi: 10.1002/adfm.201910250
- Jung M, Ghamrawi S, Du EY, Gooding JJ, Kavallaris M. Advances in 3D bioprinting for cancer biology and precision medicine: from matrix design to application. Adv Healthc Mater. 2022;11(24):e2200690. doi: 10.1002/adhm.202200690
- Crivii C-B, Boșca AB, Melincovici CS, et al. Glioblastoma microenvironment and cellular interactions. Cancers. 2022;14(4):1092. doi: 10.1002/adfm.201910250
- Thenuwara G, Javed B, Singh B, Tian F. Biosensor-enhanced organ-on-a-chip models for investigating glioblastoma tumor microenvironment dynamics. Sensors. 2024;24(9):2865. doi: 10.3390/s24092865
- Parra-Cantu C, Li W, Quiñones-Hinojosa A, Zhang YS. 3D bioprinting of glioblastoma model. J 3D Print Med. 2020;4(2):113-125. doi: 10.2217/3dp-2019-0027
- Cadena M, Ning L, King A, et al. 3D bioprinting of neural tissues. Adv Healthc Mater. 2021;10(15):e2001600. doi: 10.1002/adhm.202001600
- Khoeini R, Nosrati H, Akbarzadeh A, et al. Natural and synthetic bioinks for 3D bioprinting. Adv NanoBiomed Res. 2021;1(8):2000097. doi: 10.1002/anbr.202000097
- Leong SW, Tan SC, Norhayati MN, Monif M, Lee S-Y. Effectiveness of bioinks and the clinical value of 3d bioprinted glioblastoma models: a systematic review. Cancers. 2022;14(9):2149. doi: 10.3390/cancers14092149
- Tang M, Rich JN, Chen S. Biomaterials and 3D bioprinting strategies to model glioblastoma and the blood-brain barrier. Adv Mater. 2021;33(5):2004776. doi: 10.1002/adma.202004776v
- Ma L, Li Y, Wu Y, et al. 3D bioprinted hyaluronic acid-based cell-laden scaffold for brain microenvironment simulation. Bio-Design Manuf. 2020;3:164-174. doi: 10.1007/s42242-020-00076-6
- de la Vega L, Lee C, Sharma R, Amereh M, Willerth SM. 3D bioprinting models of neural tissues: the current state of the field and future directions. Brain Res Bull. 2019;150:240-249. doi: 10.1016/j.brainresbull.2019.06.007
- Kumar SL, Sureka P, Sowmitha A, Sentisenla J, Swathy M. Recent advancements of hydroxyapatite and polyethylene glycol (PEG) composites for tissue engineering applications—a comprehensive review. Eur Polym J. 2024;215(9):113226. doi: 10.1016/j.eurpolymj.2024.113226
- Shi L, Zhang J, Zhao M, et al. Effects of polyethylene glycol on the surface of nanoparticles for targeted drug delivery. Nanoscale. 2021;13(24):10748-10764. doi: 10.1039/D1NR02065J
- Walus K, Beyer S, Willerth SM. Three-dimensional bioprinting healthy and diseased models of the brain tissue using stem cells. Curr Opin Biomed Eng. 2020;14:25-33. doi: 10.1016/j.cobme.2020.03.002
- Lee SJ, Esworthy T, Stake S, et al. Advances in 3D bioprinting for neural tissue engineering. Adv Biosyst. 2018;2(4): 1700213. doi: 10.1002/adbi.201700213
- Esworthy TJ, Miao S, Lee S-J, et al. Advanced 4D-bioprinting technologies for brain tissue modeling and study. Int J Smart Nano Mater. 2019;10(3):177-204. doi: 10.1080/19475411.2019.1631899
- Wang X, Dai X, Zhang X, et al. 3D bioprinted glioma cell‐laden scaffolds enriching glioma stem cells via epithelial–mesenchymal transition. J Biomed Mater Res A. 2019;107(2):383-391. doi: 10.1002/jbm.a.36549
- Sánchez-Salazar MG, Álvarez MM, Trujillo-de Santiago G. Advances in 3D bioprinting for the biofabrication of tumor models. Bioprinting. 2021;21:e00120. doi: 10.1016/j.bprint.2020.e00120
- Wang X, Li X, Ding J, et al. 3D bioprinted glioma microenvironment for glioma vascularization. J Biomed Mater Res A. 2021;109(6):915-925. doi: 10.1002/jbm.a.37082
- Graber P, Dolman MEM, Jung M, Kavallaris M. Ex vivo modeling of the tumor microenvironment to develop therapeutic strategies for gliomas. Adv Ther. 2024:2300442. doi: 10.1002/adtp.202300442
- Wang X, Li X, Dai X, et al. Coaxial extrusion bioprinted shell-core hydrogel microfibers mimic glioma microenvironment and enhance the drug resistance of cancer cells. Colloids Surf B Biointerfaces. 2018;171:291-299. doi: 10.1016/j.colsurfb.2018.07.042
- Suresh P. Saponins-uptake and targeting issues for brain-specific delivery for enhanced cell death induction in glioblastoma. Lett Drug Des Discov. 2022;19(6): 473-480. doi: 10.2174/1570180819666220121145332
- Dai X, Ma C, Lan Q, Xu T. 3D bioprinted glioma stem cells for brain tumor model and applications of drug susceptibility. Biofabrication. 2016;8(4):045005. doi: 10.1088/1758-5090/8/4/045005
- Ahmed T. Biomaterial-based in vitro 3D modeling of glioblastoma multiforme. Cancer Pathog Ther. 2023;1(3):177-194. doi: 10.1016/j.cpt.2023.01.002
- Han S, Kim S, Chen Z, et al. 3D bioprinted vascularized tumour for drug testing. Int J Mol Sci. 2020;21(8):2993. doi: 10.3390/ijms21082993
- Kim EH, Song HS, Yoo SH, Yoon M. Tumor treating fields inhibit glioblastoma cell migration, invasion and angiogenesis. Oncotarget. 2016;7(40):65125-65136. doi: 10.18632/oncotarget.11372
- Tricinci O, De Pasquale D, Marino A, Battaglini M, Pucci C, Ciofani G. A 3D biohybrid real‐scale model of the brain cancer microenvironment for advanced in vitro testing. Adv Mater Technol. 2020;5(10):2000540. doi: 10.1002/admt.202000540
- Bakirci E, Schaefer N, Dahri O, et al. Melt electrowritten in vitro radial device to study cell growth and migration. Adv Biosyst. 2020;4(10):2000077. doi: 10.1002/adbi.202000077
- Huang W, Ding X, Ye H, Wang J, Shao J, Huang T. Hypoxia enhances the migration and invasion of human glioblastoma U87 cells through PI3K/Akt/mTOR/HIF-1α pathway. Neuroreport. 2018;29(18):1578-1585. doi: 10.1097/WNR.0000000000001156
- Smits IP, Blaschuk OW, Willerth SM. Novel N-cadherin antagonist causes glioblastoma cell death in a 3D bioprinted co-culture model. Biochem Biophys Res Commun. 2020;529(2):162-168. doi: 10.1016/j.bbrc.2020.06.001
- Wang X, Li X, Dai X, et al. Bioprinting of glioma stem cells improves their endotheliogenic potential. Colloids Surf B Biointerfaces. 2018;171:629-637. doi: 10.1016/j.colsurfb.2018.08.006
- Böttcher B, Pflieger A, Schumacher J, Jungnickel B, Feller K-H. 3d bioprinting of prevascularized full-thickness gelatin-alginate structures with embedded co-cultures. Bioengineering. 2022;9(6):242. doi: 10.3390/bioengineering9060242
- Dai X, Liu L, Ouyang J, et al. Coaxial 3D bioprinting of self-assembled multicellular heterogeneous tumor fibers. Sci Rep. 2017;7(1):1457. doi: 10.1038/s41598-017-01581-y
- Zhang Z, Chen X, Gao S, Fang X, Ren S. 3D bioprinted tumor model: a prompt and convenient platform for overcoming immunotherapy resistance by recapitulating the tumor microenvironment. Cell Oncol (Dordr). 2024;47:1113-1126. doi: 10.1007/s13402-024-00935-9
- Hermida MA, Kumar JD, Schwarz D, et al. Three dimensional in vitro models of cancer: Bioprinting multilineage glioblastoma models. Adv Biol Regul. 2020;75:100658. doi: 10.1016/j.jbior.2019.100658
- Jamee R, Araf Y, Naser IB, Promon SK. The promising rise of bioprinting in revolutionalizing medical science: advances and possibilities. Regen Ther. 2021;18:133-145. doi: 10.1016/j.reth.2021.05.006
- Schiffer D, Annovazzi L, Casalone C, Corona C, Mellai M. Glioblastoma: microenvironment and niche concept. Cancers. 2018;11(1):5. doi: 10.3390/cancers11010005
- Nieland L, Morsett LM, Broekman MLD, Breakefield XO, Abels ER. Extracellular vesicle-mediated bilateral communication between glioblastoma and astrocytes. Trends Neurosci. 2021;44(3):215-226. doi: 10.1016/j.tins.2020.10.014
- Figueroa J, Phillips LM, Shahar T, et al. Exosomes from glioma-associated mesenchymal stem cells increase the tumorigenicity of glioma stem-like cells via transfer of miR- 1587. Cancer Res. 2017;77(21):5808-5819. doi: 10.1158/0008-5472.can-16-2524
- Parak A, Pradeep P, du Toit LC, Kumar P, Choonara YE, Pillay V. Functionalizing bioinks for 3D bioprinting applications. Drug Discov Today. 2019;24(1):198-205. doi: 10.1016/j.drudis.2018.09.012
- Heinrich MA, Bansal R, Lammers T, Zhang YS, Michel Schiffelers R, Prakash J. 3D‐bioprinted mini‐brain: a glioblastoma model to study cellular interactions and therapeutics. Adv Mater. 2019;31(14):1806590. doi: 10.1002/adma.201806590
- Wang L, Wang C, Wu S, Fan Y, Li X. Influence of the mechanical properties of biomaterials on degradability, cell behaviors and signaling pathways: current progress and challenges. Biomater Sci. 2020;8(10):2714-2733. doi: 10.1039/D0BM00269K
- Tang M, Tiwari SK, Agrawal K, et al. Rapid 3D bioprinting of glioblastoma model mimicking native biophysical heterogeneity. Small. 2021;17(15):2006050. doi: 10.1002/smll.202006050
- Yue K, Trujillo-de Santiago G, Alvarez MM, Tamayol A, Annabi N, Khademhosseini A. Synthesis, properties, and biomedical applications of gelatin methacryloyl (GelMA) hydrogels. Biomaterials. 2015;73:254-271. doi: 10.1016/j.biomaterials.2015.08.045
- Tavsanli B, Okay O. Mechanically strong hyaluronic acid hydrogels with an interpenetrating network structure. Eur Polym J. 2017;94:185-195. doi: 10.1016/j.eurpolymj.2017.07.009
- Stanković T, Ranđelović T, Dragoj M, et al. In vitro biomimetic models for glioblastoma-a promising tool for drug response studies. Drug Resiste Updates. 2021;55: 100753. doi: 10.1016/j.drup.2021.100753
- Ivanov DP, Parker TL, Walker DA, et al. In vitro co-culture model of medulloblastoma and human neural stem cells for drug delivery assessment. J Biotechnol. 2015;205:3-13. doi: 10.1016/j.jbiotec.2015.01.002
- Northcott PA, Robinson GW, Kratz CP, et al. Medulloblastoma. Nat Rev Dis Primers. 2019;5(1):11. doi: 10.1038/s41572-019-0063-6
- Reyhanoglu G, Tadi P. Etoposide; 2020.
- Iwadate Y. Epithelial-mesenchymal transition in glioblastoma progression. Oncol Lett. 2016;11(3): 1615-1620. doi: 10.3892/ol.2016.4113
- Nowicki MO, Hayes JL, Chiocca EA, Lawler SE. Proteomic analysis implicates vimentin in glioblastoma cell migration. Cancers. 2019;11(4):466. doi: 10.3390/cancers11040466
- Huleihel L, Dziki JL, Bartolacci JG, et al. Macrophage phenotype in response to ECM bioscaffolds. Semin Immunol. 2017;29:2-13. doi: 10.1016/j.smim.2017.04.004
- Im JH, Buzzelli JN, Jones K, et al. FGF2 alters macrophage polarization, tumour immunity and growth and can be targeted during radiotherapy. Nat Commun. 2020;11(1):4064. doi: 10.1038/s41467-020-17914-x
- Chen Z, Feng X, Herting CJ, et al. Cellular and molecular identity of tumor-associated macrophages in glioblastoma. Cancer Res. 2017;77(9):2266-2278. doi: 10.1158/0008-5472.CAN-16-2310
- Sweeney MD, Zhao Z, Montagne A, Nelson AR, Zlokovic BV. Blood-brain barrier: from physiology to disease and back. Physiol Rev. 2018;99(1):21-78. doi: 10.1152/physrev.00050.2017
- Alahmari A. Blood-brain barrier overview: structural and functional correlation. Neural Plast. 2021;2021:1-10. doi: 10.1155/2021/6564585
- Aday S, Cecchelli R, Hallier-Vanuxeem D, Dehouck MP, Ferreira L. Stem cell-based human blood-brain barrier models for drug discovery and delivery. Trends Biotechnol. 2016;34(5):382-393. doi: 10.1016/j.tibtech.2016.01.001
- Gastfriend BD, Palecek SP, Shusta EV. Modeling the blood-brain barrier: Beyond the endothelial cells. Curr Opin Biomed Eng. Mar 2018;5:6-12. doi: 10.1016/j.cobme.2017.11.002
- Brown LS, Foster CG, Courtney JM, King NE, Howells DW, Sutherland BA. Pericytes and neurovascular function in the healthy and diseased brain. Front Cell Neurosci. 2019; 13:282. doi: 10.3389/fncel.2019.00282
- Heithoff BP, George KK, Phares AN, Zuidhoek IA, Munoz‐ Ballester C, Robel S. Astrocytes are necessary for blood– brain barrier maintenance in the adult mouse brain. Glia. 2021;69(2):436-472. doi: 10.1002/glia.23908
- Luo H, Shusta EV. Blood-brain barrier modulation to improve glioma drug delivery. Pharmaceutics. 2020;12(11):1085. doi: 10.3390/pharmaceutics12111085
- Chaulagain B, Gothwal A, Lamptey RNL, et al. Experimental models of in vitro blood–brain barrier for CNS drug delivery: an evolutionary perspective. Int J Mol Sci. 2023; 24(3):2710. doi: 10.3390/ijms24032710
- Sivandzade F, Cucullo L. In-vitro blood-brain barrier modeling: A review of modern and fast-advancing technologies. J Cereb Blood Flow Metab. 2018;38(10):1667-1681. doi: 10.1177/0271678X18788769
- Risau W, Wolburg H. Development of the blood-brain barrier. Trends Neurosci. 1990;13(5):174-178. doi: 10.1016/0166-2236(90)90043-a
- Ventura RD. An overview of laser-assisted bioprinting (LAB) in tissue engineering applications. Med Lasers 2021;10(2):76-81. doi: 10.25289/ML.2021.10.2.76
- Marino A, Tricinci O, Battaglini M, et al. A 3D real‐scale, biomimetic, and biohybrid model of the blood‐brain barrier fabricated through two‐photon lithography. Small. 2018;14(6):1702959. doi: 10.1002/smll.201702959
- Liu Z-L, Chen H-H, Zheng L-L, Sun L-P, Shi L. Angiogenic signaling pathways and anti-angiogenic therapy for cancer. Signal Trans Targeted Ther. 2023;8(1):198. doi: 10.1038/s41392-023-01460-1
- Akbarian M, Bertassoni LE, Tayebi L. Biological aspects in controlling angiogenesis: current progress. Cell Mol Life Sci. 2022;79(7):349. doi: 10.1007/s00018-022-04348-5
- Ansari MJ, Bokov D, Markov A, et al. Cancer combination therapies by angiogenesis inhibitors; a comprehensive review. Cell Commun Signal. 2022;20(1):49. doi: 10.1186/s12964-022-00838-y
- Cao Y, Langer R, Ferrara N. Targeting angiogenesis in oncology, ophthalmology and beyond. Nat Rev Drug Discov. 2023;22(6):476-495. doi: 10.1038/s41573-023-00671-z
- Yang Y, Cao Y. The impact of VEGF on cancer metastasis and systemic disease. Semin Cancer Biol. 2022;86(Pt 3):251-261. doi: 10.1016/j.semcancer.2022.03.011
- Ahmad A, Nawaz MI. Molecular mechanism of VEGF and its role in pathological angiogenesis. J Cell Biochem. 2022;123(12):1938-1965. doi: 10.1002/jcb.30344
- Xu D, Luo Y, Wang P, et al. Clinical progress of anti-angiogenic targeted therapy and combination therapy for gastric cancer. Front Oncol. 2023;13:1148131. doi: 10.3389/fonc.2023.1148131
- Zou G, Zhang X, Wang L, et al. Herb-sourced emodin inhibits angiogenesis of breast cancer by targeting VEGFA transcription. Theranostics. 2020;10(15):6839-6853. doi: 10.7150/thno.43622
- Majewska A, Wilkus K, Brodaczewska K, Kieda C. Endothelial cells as tools to model tissue microenvironment in hypoxia-dependent pathologies. Int J Mol Sci. 2021;22(2):520. doi: 10.3390/ijms22020520
- Lizarraga-Verdugo E, Avendano-Felix M, Bermudez M, Ramos-Payan R, Perez-Plasencia C, Aguilar-Medina M. Cancer stem cells and its role in angiogenesis and vasculogenic mimicry in gastrointestinal cancers. Front Oncol. 2020;10:413. doi: 10.3389/fonc.2020.00413
- Schulte JD, Aghi MK, Taylor JW. Anti-angiogenic therapies in the management of glioblastoma. Chin Clin Oncol. 2021;10(4):37. doi: 10.21037/cco.2020.03.06
- Claesson-Welsh L, Welsh M. VEGFA and tumour angiogenesis. J Intern Med. 2013;273(2):114-27. doi: 10.1111/joim.12019
- Mandrycky CJ, Howard CC, Rayner SG, Shin YJ, Zheng Y. Organ-on-a-chip systems for vascular biology. J Mol Cell Cardiol. 2021;159:1-13. doi: 10.1016/j.yjmcc.2021.06.002
- Pollet A, den Toonder JMJ. Recapitulating the vasculature using organ-on-chip technology. Bioengineering (Basel). 2020;7(1):17. doi: 10.3390/bioengineering7010017
- Farhang Doost N, Srivastava SK. A comprehensive review of organ-on-a-chip technology and its applications. Biosensors. 2024;14(5):225. doi: 10.3390/bios14050225
- Kumar V, Varghese S. Ex vivo tumor‐on‐a‐chip platforms to study intercellular interactions within the tumor microenvironment. Adv Healthc Mater. 2019;8(4): 1801198. doi: 10.1002/adhm.201801198
- Fetah K, Tebon P, Goudie MJ, et al. The emergence of 3D bioprinting in organ-on-chip systems. Prog Biomed Eng. 2019;1(1):012001. doi: 10.1088/2516-1091/ab23df
- Gao Q, Liu Z, Lin Z, et al. 3D bioprinting of vessel-like structures with multilevel fluidic channels. ACS Biomater Sci Eng. 2017;3(3):399-408. doi: 10.1021/acsbiomaterials.6b00643
- Samadian H, Jafari S, Sepand M, et al. 3D bioprinting technology to mimic the tumor microenvironment: tumor-on-a-chip concept. Mater Today Adv. 2021;12:100160. doi: 10.1016/j.mtadv.2021.100160
- Alves AH, Nucci MP, Mamani JB, et al. The advances in glioblastoma on-a-chip for therapy approaches. Cancers. 2022;14(4):869. doi: 10.3390/cancers14040869
- Song Q, Sun J, Mu Y, Xu Y, Zhu Q, Jin Q. A new method for polydimethylsiloxane (PDMS) microfluidic chips to maintain vacuum-driven power using Parylene C. Sens Actuators B: Chem. 2018;256:1122-1130. doi: 10.1016/j.snb.2017.10.006
- Tabatabaei Rezaei N, Kumar H, Liu H, Lee SS, Park SS, Kim K. Recent advances in organ-on-chips integrated with bioprinting technologies for drug screening. Adv Healthc Mater. 2023;12(20):e2203172. doi: 10.1002/adhm.202203172
- Nie J, Gao Q, Fu J, He Y. Grafting of 3D bioprinting to in vitro drug screening: a review. Adv Healthc Mater. 2020;9(7):e1901773. doi: 10.1002/adhm.201901773
- Radhakrishnan J, Varadaraj S, Dash SK, Sharma A, Verma RS. Organotypic cancer tissue models for drug screening: 3D constructs, bioprinting and microfluidic chips. Drug Discov Today. 2020;25(5):879-890. doi: 10.1016/j.drudis.2020.03.002
- Yang Z, Wu S, Fontana F, et al. The tight junction protein Claudin-5 limits endothelial cell motility. J Cell Sci. 2021;134(1):jcs248237. doi: 10.1242/jcs.248237
- Greene C, Campbell M. Tight junction modulation of the blood brain barrier: CNS delivery of small molecules. Tissue Barriers. 2016;4(1):e1138017. doi: 10.1080/21688370.2015.1138017
- Yang JY, Shin D-S, Jeong M, et al. Evaluation of drug blood-brain-barrier permeability using a microfluidic chip. Pharmaceutics. 2024;16(5):574. doi: 10.3390/pharmaceutics16050574
- Chittiboyina S, Rahimi R, Atrian F, Ochoa M, Ziaie B, Lelievre SA. Gradient-on-a-chip with reactive oxygen species reveals thresholds in the nucleus response of cancer cells depending on the matrix environment. ACS Biomater Sci Eng. 2018;4(2):432-445. doi: 10.1021/acsbiomaterials.7b00087
- Sontheimer-Phelps A, Hassell BA, Ingber DE. Modelling cancer in microfluidic human organs-on-chips. Nat Rev Cancer. 2019;19(2):65-81. doi: 10.1038/s41568-018-0104-6
- Malik A, Pal R, Gupta SK. EGF-mediated reduced miR-92a- 1-5p controls HTR-8/SVneo cell invasion through activation of MAPK8 and FAS which in turn increase MMP-2/-9 expression. Sci Rep. 2020;10(1):12274. doi: 10.1038/s41598-020-68966-4
- Liu D, Yang T, Ma W, Wang Y. Clinical strategies to manage adult glioblastoma patients without MGMT hypermethylation. J Cancer. 2022;13(1):354. doi: 10.7150/jca.63595
- Qu S, Qi S, Zhang H, et al. Albumin-bound paclitaxel augment temozolomide treatment sensitivity of glioblastoma cells by disrupting DNA damage repair and promoting ferroptosis. J Exp Clin Cancer Res. 2023;42(1):285. doi: 10.1186/s13046-023-02843-6
- Tsai C-Y, Ko H-J, Huang C-YF, et al. Ionizing radiation induces resistant glioblastoma stem-like cells by promoting autophagy via the Wnt/β-catenin pathway. Life. 2021;11(5):451. doi: 10.3390/life11050451
- Yu W, Zhang L, Wei Q, Shao A. O6-methylguanine- DNA methyltransferase (MGMT): challenges and new opportunities in glioma chemotherapy. Front Oncol. 2020;9:1547. doi: 10.3389/fonc.2019.01547
- Kohutova A, Munzova D, Pesl M, Rotrekl V. alpha(1)- Adrenoceptor agonist methoxamine inhibits base excision repair via inhibition of apurinic/apyrimidinic endonuclease 1 (APE1). Acta Pharm. 2023;73(2):281-291. doi: 10.2478/acph-2023-0012 doi: 10.1007/s11154-020-09551-y
- Burman P, Lamb L, McCormack A. Temozolomide therapy for aggressive pituitary tumours–current understanding and future perspectives. Rev Endocr Metab Disord. 2020;21:263-276.
- Erasimus H, Gobin M, Niclou S, Van Dyck E. DNA repair mechanisms and their clinical impact in glioblastoma. Mutat Res Rev Mutat Res. 2016;769:19-35. doi: 10.1016/j.mrrev.2016.05.005
- Shu J, Wang X, Yang X, Zhao G. Author correction: ATM inhibitor KU60019 synergistically sensitizes lung cancer cells to topoisomerase II poisons by multiple mechanisms. Sci Rep. 2024;14(1):8785. doi: 10.1038/s41598-024-59332-9
- Wang C, Li J, Sinha S, Peterson A, Grant GA, Yang F. Mimicking brain tumor-vasculature microanatomical architecture via co-culture of brain tumor and endothelial cells in 3D hydrogels. Biomaterials. 2019;202:35-44. doi: 10.1016/j.biomaterials.2019.02.024
- Nguyen T, Sarkar T, Tran T, Moinuddin SM, Saha D, Ahsan F. Multilayer soft photolithography fabrication of microfluidic devices using a custom-built wafer-scale PDMS slab aligner and cost-efficient equipment. Micromachines (Basel). 2022;13(8):1357. doi: 10.3390/mi13081357
- Neufeld L, Yeini E, Reisman N, et al. Microengineered perfusable 3D-bioprinted glioblastoma model for in vivo mimicry of tumor microenvironment. Sci Adv. 2021;7(34):eabi9119. doi: 10.1126/sciadv.abi9119
- Schober AL, Wicki-Stordeur LE, Murai KK, Swayne LA. Foundations and implications of astrocyte heterogeneity during brain development and disease. Trends Neurosci. 2022;45(9):692-703. doi: 10.1016/j.tins.2022.06.009
- Slika H, Karimov Z, Alimonti P, et al. Preclinical models and technologies in glioblastoma research: evolution, current state, and future avenues. Int J Mol Sci. 2023;24(22):16316. doi: 10.3390/ijms242216316
- Jung S, Jo H, Hyung S, Jeon NL. Advances in 3D vascularized tumor-on-a-Chip technology. Adv Exp Med Biol.2022;1379:231-256. doi: 10.1007/978-3-031-04039-9_9
- Li W, Zhou Z, Zhou X, et al. 3D biomimetic models to reconstitute tumor microenvironment in vitro: spheroids, organoids, and tumor‐on‐a‐chip. Adv Healthc Mater. 2023;12(18):2202609. doi: 10.1002/adhm.202202609
- Langhans SA. Three-dimensional in vitro cell culture models in drug discovery and drug repositioning. Front Pharmacol. 2018;9:6. doi: 10.3389/fphar.2018.00006
- Joseph JS, Malindisa ST, Ntwasa M. Two-dimensional (2D) and three-dimensional (3D) cell culturing in drug discovery. Cell Cult. 2018;2:1-22. doi: 10.5772/intechopen.81552
- Hagenbuchner J, Nothdurfter D, Ausserlechner MJ. 3D bioprinting: novel approaches for engineering complex human tissue equivalents and drug testing. Essays Biochem. 2021;65(3):417-427. doi: 10.1042/EBC20200153
- Sunildutt N, Parihar P, Chethikkattuveli Salih AR, Lee SH, Choi KH. Revolutionizing drug development: harnessing the potential of organ-on-chip technology for disease modeling and drug discovery. Front Pharmacol. 2023;14:1139229. doi: 10.3389/fphar.2023.1139229
- Peng W, Datta P, Ayan B, Ozbolat V, Sosnoski D, Ozbolat IT. 3D bioprinting for drug discovery and development in pharmaceutics. Acta Biomater. 2017;57:26-46. doi: 10.1016/j.actbio.2017.05.025
- Satpathy A, Datta P, Wu Y, Ayan B, Bayram E, Ozbolat IT. Developments with 3D bioprinting for novel drug discovery. Expert Opin Drug Discov. 2018;13(12):1115-1129. doi: 10.1080/17460441.2018.1542427
- Yun EJ, Kim S, Hsieh JT, Baek ST. Wnt/beta-catenin signaling pathway induces autophagy-mediated temozolomide-resistance in human glioblastoma. Cell Death Dis. 2020;11(9):771. doi: 10.1038/s41419-020-02988-8
- Song Q, Peng S, Sun Z, Heng X, Zhu X. Temozolomide drives ferroptosis via a DMT1-dependent pathway in glioblastoma cells. Yonsei Med J. 2021;62(9):843-849. doi: 10.3349/ymj.2021.62.9.843
- Karve AS, Desai JM, Gadgil SN, et al. A review of approaches to potentiate the activity of temozolomide against glioblastoma to overcome resistance. Int J Mol Sci. 2024;25(6):3217. doi: 10.3390/ijms25063217
- Ibarra LE, Vilchez ML, Caverzan MD, Milla Sanabria LN. Understanding the glioblastoma tumor biology to optimize photodynamic therapy: From molecular to cellular events. J Neurosci Res. 2021;99(4):1024-1047. doi: 10.1002/jnr.24776
- Reed MR, Maddukuri L, Ketkar A, et al. Inhibition of tryptophan 2,3-dioxygenase impairs DNA damage tolerance and repair in glioma cells. NAR Cancer. 2021;3(2):zcab014. doi: 10.1093/narcan/zcab014
- Cirri D, Chiaverini L, Pratesi A, Marzo T. Is the next cisplatin already in our laboratory? Comm Inorganic Chem. 2023;43(6):465-478. doi: 10.1080/02603594.2022.2152016
- Luo H, Yi T, Huang D, et al. circ_PTN contributes to-cisplatin resistance in glioblastoma via PI3K/AKT signaling through the miR-542-3p/PIK3R3 pathway. Mol Ther Nucleic Acids. 2021;26:1255-1269. doi: 10.1016/j.omtn.2021.08.034
- Lee SH, Kang MS, Jeon S, et al. 3D bioprinting of human mesenchymal stem cells-laden hydrogels incorporating MXene for spontaneous osteodifferentiation. Heliyon. 2023;9(3):e14490. doi: 10.1016/j.heliyon.2023.e14490
- Vignesh A, Binoy A, Thurakkal L, Bhuvanesh NS, Sadhukhan S, Porel M. Pyrene aroylhydrazone-based Pd (II) complexes for DNA/protein binding, cellular imaging and in vitro anticancer activity via ROS production. J Mol Struct. 2024;1295:136693. doi: 10.1016/j.molstruc.2023.136693
- Ma Q, Sun J, Wang H, et al. Far upstream element-binding protein 1 confers lobaplatin resistance by transcriptionally activating PTGES and facilitating the arachidonic acid metabolic pathway in osteosarcoma. Med Comm (2020). 2023;4(3):e257. doi: 10.1002/mco2.257
- Du L, Fei Z, Song S, Wei N. Antitumor activity of Lobaplatin against esophageal squamous cell carcinoma through caspase-dependent apoptosis and increasing the Bax/Bcl-2 ratio. Biomed Pharmacother. 2017;95:447-452. doi: 10.1016/j.biopha.2017.08.119
- Vaz-Salgado MA, Villamayor M, Albarran V, et al. Recurrent glioblastoma: a review of the treatment options. Cancers (Basel). 2023;15(17):4279. doi: 10.3390/cancers15174279
- Yamamuro S, Takahashi M, Satomi K, et al. Lomustine and nimustine exert efficient antitumor effects against glioblastoma models with acquired temozolomide resistance. Cancer Sci. 2021;112(11):4736-4747. doi: 10.1111/cas.15141
- Schneider M, Potthoff AL, Evert BO, et al. Inhibition of Intercellular Cytosolic Traffic via Gap Junctions Reinforces Lomustine-Induced Toxicity in Glioblastoma Independent of MGMT Promoter Methylation Status. Pharmaceuticals (Basel). 2021;14(3):195. doi: 10.3390/ph14030195
- Amrein PC. Low-dose dasatinib: when less can be more. Lancet Haematol. 2021;8(12):e867-e868. doi: 10.1016/S2352-3026(21)00342-2
- Owusu A, Bonnaire K, Hailemeskel B. Lovastatin use in transplant: a review and survey. Int J Pharm Res Allied Sci. 2024;13(1):83-90. doi: 10.51847/LJ2kXhjbtG
- Alhalabi OT, Fletcher MNC, Hielscher T, et al. A novel patient stratification strategy to enhance the therapeutic efficacy of dasatinib in glioblastoma. Neuro Oncol. 2022;24(1): 39-51. doi: 10.1093/neuonc/noab158
- Ryu KY, Lee HJ, Woo H, et al. Dasatinib regulates LPS-induced microglial and astrocytic neuroinflammatory responses by inhibiting AKT/STAT3 signaling. J Neuroinflammation. 2019;16(1):190. doi: 10.1186/s12974-019-1561-x
- Liu PC, Lu G, Deng Y, et al. Inhibition of NF-kappaB pathway and modulation of MAPK signaling pathways in glioblastoma and implications for lovastatin and tumor necrosis factor-related apoptosis inducing ligand (TRAIL) combination therapy. PLoS One. 2017;12(1):e0171157. doi: 10.1371/journal.pone.0171157
- Zhu Z, Zhang P, Li N, et al. Lovastatin enhances cytotoxicity of temozolomide via impairing autophagic flux in glioblastoma cells. Biomed Res Int. 2019;2019:2710693. doi: 10.1155/2019/2710693
- Wu Q, Zhu C, Zhang S, Zhou Y, Zhong Y. Hematological toxicities of concurrent chemoradiotherapies in head and neck cancers: comparison among cisplatin, nedaplatin, lobaplatin, and nimotuzumab. Front Oncol. 2021;11:762366. doi: 10.3389/fonc.2021.762366
- Hua S, Kong X, Chen B, et al. Anticancer mechanism of lobaplatin as monotherapy and in combination with paclitaxel in human gastric cancer. Curr Mol Pharmacol. 2018;11(4):316-325. doi: 10.2174/1874467211666180813095050
- Weller M, Le Rhun E. How did lomustine become standard of care in recurrent glioblastoma? Cancer Treat Rev. 2020;87:102029. doi: 10.1016/j.ctrv.2020.102029
- Mekala JR, Adusumilli K, Chamarthy S, Angirekula HSR. Novel sights on therapeutic, prognostic, and diagnostics aspects of non-coding RNAs in glioblastoma multiforme. Metabolic Brain Dis. 2023;38(6):1801-1829. doi: 10.1007/s11011-023-01234-2
- Wang X, Luo Y, Ma Y, Wang P, Yao R. Converging bioprinting and organoids to better recapitulate the tumor microenvironment. Trends Biotechnol. 2024;42(5):648-663. doi: 10.1016/j.tibtech.2023.11.006
- Li Y, Liu J, Xu S, Wang J. 3D bioprinting: an important tool for tumor microenvironment research. Int J Nanomed. 2023:18;8039-8057. doi: 10.2147/IJN.S435845
- Shah B, Dong X. Current status of in vitro models of the blood-brain barrier. Curr Drug Deliv. 2022; doi: 10.2174/1567201819666220303102614
- Yigci D, Sarabi MR, Ustun M, et al. 3D bioprinted glioma models. Prog Biomed Eng. 2022;4(4):042001. doi: 10.1088/2516-1091/ac7833