Development of a biocompatible radiotherapy spacer using 3D printing and microcellular foaming process for enhanced prostate cancer treatment
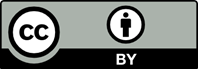
In this study, a new active spacer was developed using three-dimensional (3D) printing technology and microcellular foaming process to overcome the limitations of current spacers used in prostate cancer treatment. In prostate cancer treatment, a spacer is inserted between the prostate and rectum to increase the distance between the two organs so as to reduce the radiation dose to the rectum. Radiotherapy spacer is widely used in particle therapy, such as proton and carbon beams, and X-ray radiation therapies, including intensity-modulated radiotherapy and stereotactic body radiation therapy. However, existing spacers have been reported to cause significant side effects. Therefore, this study introduces a 3D printing technique using polycaprolactone to develop a spacer that provides customized treatment and minimizes side effects. This technique utilizes the volume expansion that occurs when a 3D spacer printed to fit a patient’s organs undergoes foaming through a supercritical carbon dioxide (scCO2)-assisted microcellular foaming process. Additionally, the use of scCO2 allows simultaneous gas absorption and sterilization, thereby reducing the number of process steps. This study confirms the potential of the newly developed spacer to provide effective and safe radiotherapy for prostate cancer, reduce patient discomfort, and minimize rectal side effects during radiation treatment.

- American Cancer Society. The cancer atlas: the burden: Europe [Internet]. Published Accessed at: December 9, 2020. https://canceratlas.cancer.org/the-burden/europe/
- Datta NR, Stutz E, Rogers S, Bodis S. Conventional versus hypofractionated Radiation therapy for localized or locally advanced prostate cancer: a systematic review and meta-analysis along with therapeutic implications. Int J Radiat Oncol Biol Phys. 2017;99(3):573-589. doi: 10.1016/j.ijrobp.2017.07.021
- Heemsbergen WD, Peeters STH, Koper PCM, Hoogeman MS, Lebesque JV. Acute and late gastrointestinal toxicity after radiotherapy in prostate cancer patients: Consequential late damage. Int J Radiat Oncol Biol Phys. 2006;66(1):3-10. doi: 10.1016/j.ijrobp.2006.03.055
- Noel Rolon. Letter to Noel Rolon. Food and Drug Administration; 2015. Accessed December 2, 2020. https://www. accessdata.fda.gov/cdrh_docs/pdf14/ DEN140030.pdf
- Payne HA, Pinkawa M, Peedell C, Bhattacharyya SK, Woodward E, Miller LE. SpaceOAR hydrogel spacer injection prior to stereotactic body radiation therapy for men with localized prostate cancer: a systematic review. Medicine (Baltimore). 2021;100(49):e28111. doi: 10.1097/MD.0000000000028111
- Whalley D, Hruby G, Alfieri F, Kneebone A, Eade T. SpaceOAR hydrogel in dose-escalated prostate cancer radiotherapy: rectal dosimetry and late toxicity. Clin Oncol. 2016;28(10):e148-e154. doi: 10.1016/j.clon.2016.05.005
- Babar M, Katz A, Ciatto M. Dosimetric and clinical outcomes of SpaceOAR in men undergoing external beam radiation therapy for localized prostate cancer: a systematic review. J Med Imaging Radiat Oncol. 2021;65(3):384-397. doi: 10.1111/1754-9485.13179
- Sanei M, Ghaffari H, Ardekani M, et al. Effectiveness of rectal displacement devices during prostate external-beam radiation therapy: a review. J Cancer Res Ther. 2021;17(2):303-310. doi: 10.4103/jcrt.JCRT_841_19
- Afkhami Ardekani M, Ghaffari H, Navaser M, Zoljalali Moghaddam SH, Refahi S. Effectiveness of rectal displacement devices in managing prostate motion: a systematic review. Strahlenther Onkol. 2021;197(2):97-115. doi: 10.1007/s00066-020-01633-9
- Van Lin ENJT, Hoffmann AL, Van Kollenburg P, Leer JW, Visser AG. Rectal wall sparing effect of three different endorectal balloons in 3D conformal and IMRT prostate radiotherapy. Int J Radiat Oncol Biol Phys. 2005;63(2):565-576. doi: 10.1016/j.ijrobp.2005.05.010
- Ghaffari H, Mehrabian A. Rectal retractor in prostate radiotherapy: pros and cons. Radiat Oncol. 2022;17(1):204. doi: 10.1186/s13014-022-02176-2
- Shi S, Vissapragada R, Abi Jaoude J, et al. Evolving role of biomaterials in diagnostic and therapeutic radiation oncology. Bioact Mater. 2020;5(2):233-240. doi: 10.1016/j.bioactmat.2020.01.011
- Armstrong N, Bahl A, Pinkawa M, et al. SpaceOAR hydrogel spacer for reducing radiation toxicity during radiotherapy for prostate cancer. A systematic review. Urology. 2021;156:e74-e85. doi: 10.1016/j.urology.2021.05.013
- Mahdavi SR, Ghaffari H, Mofid B, Rostami A, Reiazi R, Janani L. Rectal retractor application during image-guided dose-escalated prostate radiotherapy. Strahlenther Onkol. 2019;195(10):923-933. doi: 10.1007/s00066-019-01445-6
- Aminsharifi A, Kotamarti S, Silver D, Schulman A. Major complications and adverse events related to the injection of the spaceoar hydrogel system before radiotherapy for prostate cancer: review of the manufacturer and user facility device experience database. J Endourol. 2019;33(10): 868-871. doi: 10.1089/end.2019.0431
- Mohamed RM, Yusoh K. A review on the recent research of polycaprolactone (PCL). Adv Mat Res. 2015;1134:249-255. doi: 10.4028/www.scientific.net/amr.1134.249
- Zhang Q, Jiang Y, Zhang Y, Ye Z, Tan W, Lang M. Effect of porosity on long-term degradation of poly (ε-caprolactone) scaffolds and their cellular response. Polym Degrad Stab. 2013;98(1):209-218. doi: 10.1016/j.polymdegradstab.2012.10.008
- Yeong WY, Sudarmadji N, Yu HY, et al. Porous polycaprolactone scaffold for cardiac tissue engineering fabricated by selective laser sintering. Acta Biomater. 2010;6(6):2028-2034. doi: 10.1016/j.actbio.2009.12.033
- Woodruff MA, Hutmacher DW. The return of a forgotten polymer - polycaprolactone in the 21st century. Prog Polymer Sci (Oxf). 2010;35(10):1217-1256. doi: 10.1016/j.progpolymsci.2010.04.002
- Malikmammadov E, Tanir TE, Kiziltay A, Hasirci V, Hasirci N. PCL and PCL-based materials in biomedical applications. J Biomater Sci Polym Ed. 2018;29(7-9):863-893. doi: 10.1080/09205063.2017.1394711
- Dugad R, Radhakrishna G, Gandhi A. Recent advancements in manufacturing technologies of microcellular polymers: a review. J Polymer Res. 2020;27(7):182. doi: 10.1007/s10965-020-02157-7
- Cha SW. A Microcellular Foaming/Forming Process Performed at Ambient Temperature and a Super-Microcellular Foaming Process. Mechanical Engineering Department; 1994. Ph D Thesis. Accessed June 9, 2023. https://cir.nii.ac.jp/crid/1572824498934882176.bib?lang=ja
- Hou J, Jiang J, Guo H, et al. Fabrication of fibrillated and interconnected porous poly(ϵ-caprolactone) vascular tissue engineering scaffolds by microcellular foaming and polymer leaching. RSC Adv. 2020;10(17):10055-10066. doi: 10.1039/d0ra00956c
- Wang H, Li W, Kumar V. Solid-State Foaming Of Polycaprolactone (PCL); 2007. http://www.asme.org
- Hatami T, Johner JCF, de Castro KC, Mei LHI, Vieira MGA, Angela M. New insight into a step-by-step modeling of supercritical CO2 foaming to fabricate poly(ε-caprolactone) scaffold. Ind Eng Chem Res. 2020;59(45):20033-20044. doi: 10.1021/acs.iecr.0c04372
- Kosowska K, Krzysztoforski J, Henczka M. Foaming of PCL-based composites using scCO2: structure and physical properties. Materials (Basel). 2022;15(3):1169. doi: 10.3390/ma15031169
- Ribeiro N, Soares GC, Santos-Rosales V, et al. A new era for sterilization based on supercritical CO2 technology. J Biomed Mater Res B Appl Biomater. 2020;108(2):399-428. doi: 10.1002/jbm.b.34398
- Soares GC, Learmonth DA, Vallejo MC, et al. Supercritical CO2 technology: the next standard sterilization technique? Mater Sci Eng C. 2019;99:520-540. doi: 10.1016/j.msec.2019.01.121
- Matthews IP, Gibson C, Samuel AH. Sterilisation of implantable devices. Clin Mater. 1994;15(3):191-215. doi: 10.1016/0267-6605(94)90082-5
- Tipnis NP, Burgess DJ. Sterilization of implantable polymer-based medical devices: a review. Int J Pharm. 2018;544(2):455-460. doi: 10.1016/j.ijpharm.2017.12.003
- Rogers WJ. 2 - Steam and dry heat sterilization of biomaterials and medical devices. In: Lerouge S, Simmons A, eds. Sterilisation of Biomaterials and Medical Devices. Woodhead Publishing; 2012:20-55. doi: 10.1533/9780857096265.20
- Li G, Li Y, Wang J, et al. Guidelines for radiotherapy of prostate cancer (2020 edition). Precis Radiat Oncol. 2021;5(3):160-182. doi: 10.1002/pro6.1129
- Kuboki T. Foaming behavior of cellulose fiber-reinforced polypropylene composites in extrusion. J Cell Plastics. 2014;50(2):113-128. doi: 10.1177/0021955X13504775
- Gong P, Taniguchi T, Ohshima M. Nanoporous structure of the cell walls of polycarbonate foams. J Mater Sci. 2014;49(6):2605-2617. doi: 10.1007/s10853-013-7959-4
- Baker SC, Rohman G, Southgate J, Cameron NR. The relationship between the mechanical properties and cell behaviour on PLGA and PCL scaffolds for bladder tissue engineering. Biomaterials. 2009;30(7):1321-1328. doi: 10.1016/j.biomaterials.2008.11.033
- Onizuka R, Araki F, Ohno T, et al. Accuracy of dose calculation algorithms for virtual heterogeneous phantoms and intensity-modulated radiation therapy in the head and neck. Radiol Phys Technol. 2016;9(1):77-87. doi: 10.1007/s12194-015-0336-z
- Wang K, Mavroidis P, Royce TJ, et al. Prostate stereotactic body radiation therapy: an overview of toxicity and dose response. Int J Radiat Oncol Biol Phys. 2021;110(1): 237-248. doi: 10.1016/j.ijrobp.2020.09.054