3D-bioprinted RGD-Alg/GelMA/PCL scaffolds laden with Schwann-like cells for peripheral nerve reconstruction
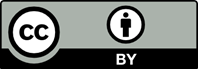
Autograft nerve transplantation is widely considered the gold standard for treating peripheral nerve defects, but it does have certain drawbacks. Alternatively, tissue engineering holds significant promise for nerve regeneration. In this study, three-dimensional (3D) bioprinting was utilized to fabricate a nerve regenerative conduit with arginine-glycine-aspartic acid-modified sodium alginate (RGD-Alg)/gelatin methacrylate (GelMA)/polycaprolactone (PCL) laden with induced Schwann-like stem cells from human-exfoliated deciduous teeth (scSHEDs). Stem cells from human-exfoliated deciduous teeth (SHEDs) could be stably induced into scSHEDs, as indicated by enhanced immunofluorescence staining for S-100β, GFAP, and P0 proteins. The physical properties of the 6% RGD-Alg/5% GelMA hydrogel were determined through rheological and maximum tensile testing, displaying decent mechanical and rheological characteristics. Incorporating PCL layers into the hydrogel significantly enhanced the scaffold’s physical properties. Live/dead cell staining and nerve growth factor (NGF) release test confirmed the growth of scSHEDs within the 6% RGD-Alg/5% GelMA hydrogel. S-100β immunofluorescence and cytoskeleton stainings verified the growth of scSHEDs within the scaffold. Eight weeks after anastomosis in rat models with sciatic nerve defects, electrophysiological examination, functional assessment, and immunohistochemical staining were conducted. The results indicated that the 3D-bioprinted scSHED-laden RGD-Alg/GelMA/PCL scaffold enhanced the efficacy of promoting nerve regeneration compared to the hydrogel and hydrogel/PCL scaffolds. Overall, this study highlighted the potential of scSHEDs and PCL enhancement as viable improvements to RGD-Alg/GelMA hydrogels for constructing nerve regenerative conduit. The 3D-bioprinted composite scaffold is expected to provide new insights into peripheral nerve regeneration and has a wide range of clinical prospects.
- Li S, Xie S, Li Z, et al. Facial reanimation after peripheral facial nerve paralysis: a single-institution surgical experience. J Craniofac Surg. 2023;34(6):1841-1844. doi: 10.1097/SCS.0000000000009513
- Tieman TE, Chaiet SR, Luijmes R, et al. A closer look at the paralyzed face; a narrative review of the neurobiological basis for functional and aesthetic appreciation between patients with a left and a right peripheral facial palsy. J Plast Reconstr Aesthet Surg. 2020;73(8):1434-1441. doi: 10.1016/j.bjps.2020.03.030
- Bruins TE, van Veen MM, Mooibroek-Leeuwerke T, Werker PMN, Broekstra DC, Dijkstra PU. Association of socioeconomic, personality, and mental health factors with health-related quality of life in patients with facial palsy. JAMA Otolaryngol Head Neck Surg. 2020;146(4): 331-337. doi: 10.1001/jamaoto.2019.4559
- Mohanty AJ, Perez JL, Hembd A, Thrikutam NP, Bartley J, Rozen SM. Orbicularis oculi muscle reinnervation confers corneal protective advantages over static interventions alone in the subacute facial palsy patient. Plast Reconstr Surg. 2020;145(3):791-801. doi: 10.1097/PRS.0000000000006608
- Yi CR, Oh TM, Jeong WS, Choi JW, Oh TS. Quantitative analysis of the impact of radiotherapy on facial nerve repair with sural nerve grafting after parotid gland surgery. J Craniomaxillofac Surg. 2020;48(8):724-732. doi: 10.1016/j.jcms.2020.05.012
- Karp E, Waselchuk E, Landis C, Fahnhorst J, Lindgren B, Lyford-Pike S. Facial rehabilitation as noninvasive treatment for chronic facial nerve paralysis. Otol Neurotol. 2019;40(2):241-245. doi: 10.1097/MAO.0000000000002107
- Azizzadeh B, Irvine LE, Diels J, et al. Modified selective neurectomy for the treatment of post-facial paralysis synkinesis. Plast Reconstr Surg. 2019;143(5):1483-1496. doi: 10.1097/PRS.0000000000005590
- Wang X, Yu Y, Yang C, Shang L, Zhao Y, Shen X. Dynamically responsive scaffolds from microfluidic 3D printing for skin flap regeneration. Adv Sci (Weinh). 2022;9(22):e2201155. doi: 10.1002/advs.202201155
- Li Z, Zhao Y, Wang Z, et al. Engineering multifunctional hydrogel-integrated 3D printed bioactive prosthetic interfaces for osteoporotic osseointegration. Adv Healthc Mater. 2022;11(11):e2102535. doi: 10.1002/adhm.202102535
- Mei Q, Rao J, Bei HP, Liu Y, Zhao X. 3D bioprinting photo-crosslinkable hydrogels for bone and cartilage repair. Int J Bioprint. 2021;7(3):367. doi: 10.18063/ijb.v7i3.367
- Sarker MD, Naghieh S, McInnes AD, Schreyer DJ, Chen X. Regeneration of peripheral nerves by nerve guidance conduits: influence of design, biopolymers, cells, growth factors, and physical stimuli. Prog Neurobiol. 2018;171:125-150. doi: 10.1016/j.pneurobio.2018.07.002
- Rodriguez-Sanchez DN, Pinto G, Cartarozzi LP, et al. 3D-printed nerve guidance conduits multi-functionalized with canine multipotent mesenchymal stromal cells promote neuroregeneration after sciatic nerve injury in rats. Stem Cell Res Ther. 2021;12(1):303. doi: 10.1186/s13287-021-02315-8
- Liu K, Yan L, Li R, et al. 3D printed personalized nerve guide conduits for precision repair of peripheral nerve defects. Adv Sci (Weinh). 2022;9(12):e2103875. doi: 10.1002/advs.202103875
- Oatari M, Uehara M, Shimizu F. Evaluation of the effects of a polyglycolic acid-collagen tube in the regeneration of facial nerve defects in rats. Int J Artif Organs. 2018;41(10):664-669. doi: 10.1177/0391398818783860
- Fang Y, Wang C, Liu Z, et al. 3D printed conductive multiscale nerve guidance conduit with hierarchical fibers for peripheral nerve regeneration. Adv Sci (Weinh). 2023;10(12):e2205744. doi: 10.1002/advs.202205744
- Chakraborty A, Roy A, Ravi SP, Paul A. Exploiting the role of nanoparticles for use in hydrogel-based bioprinting applications: concept, design, and recent advances. Biomater Sci. 2021;9(19):6337-6354. doi: 10.1039/d1bm00605c
- Liu XY, Chen C, Xu HH, et al. Integrated printed BDNF/ collagen/chitosan scaffolds with low temperature extrusion 3D printer accelerated neural regeneration after spinal cord injury. Regen Biomater. 2021;8(6):rbab047 doi: 10.1093/rb/rbab047
- Wu Z, Li Q, Xie S, Shan X, Cai Z. In vitro and in vivo biocompatibility evaluation of a 3D bioprinted gelatin-sodium alginate/rat Schwann-cell scaffold. Mater Sci Eng C Mater Biol Appl. 2020;109:110530. doi: 10.1016/j.msec.2019.110530
- Wu Z, Xie S, Kang Y, Shan X, Li Q, Cai Z. Biocompatibility evaluation of a 3D-bioprinted alginate-GelMA-bacteria nanocellulose (BNC) scaffold laden with oriented-growth RSC96 cells. Mater Sci Eng C Mater Biol Appl. 2021;129:112393. doi: 10.1016/j.msec.2021.112393
- Ravanbakhsh H, Karamzadeh V, Bao G, Mongeau L, Juncker D, Zhang YS. Emerging technologies in multi-material bioprinting. Adv Mater. 2021;33(49):e2104730. doi: 10.1002/adma.202104730
- Zhu L, Jia S, Liu T, et al. Aligned PCL fiber conduits immobilized with nerve growth factor gradients enhance and direct sciatic nerve regeneration. Adv Funct Mater. 2020;30(39). doi: 10.1002/adfm.202002610
- Dziadek M, Dziadek K, Checinska K, et al. PCL and PCL/ bioactive glass biomaterials as carriers for biologically active polyphenolic compounds: comprehensive physicochemical and biological evaluation. Bioact Mater. 2021;6(6):1811-1826. doi: 10.1016/j.bioactmat.2020.11.025
- Kim MJ, Park JH, Seok JM, et al. BMP-2-immobilized PCL 3D printing scaffold with a leaf-stacked structure as a physically and biologically activated bone graft. Biofabrication. 2024;16(2):025014. doi: 10.1088/1758-5090/ad2537
- Li Y, Lv S, Yuan H, et al. Peripheral nerve regeneration with 3D printed bionic scaffolds loading neural crest stem cell derived schwann cell progenitors. Adv Funct Mater. 2021;31(16). doi: 10.1002/adfm.202010215
- Zhang Q, Nguyen PD, Shi S, et al. Neural crest stem-like cells non-genetically induced from human gingiva-derived mesenchymal stem cells promote facial nerve regeneration in rats. Mol Neurobiol. 2018;55(8): 6965-6983. doi: 10.1007/s12035-018-0913-3
- Lee HS, Jeon EY, Nam JJ, et al. Development of a regenerative porous PLCL nerve guidance conduit with swellable hydrogel-based microgrooved surface pattern via 3D printing. Acta Biomater. 2022;141:219-232. doi: 10.1016/j.actbio.2022.01.042
- Hua L, Qian H, Lei T, Zhang Y, Lei P, Hu Y. 3D-printed porous tantalum coated with antitubercular drugs achieving antibacterial properties and good biocompatibility. Macromol Biosci. 2022;22(1):e2100338. doi: 10.1002/mabi.202100338
- Tang N, Wang X, Zhu J, Sun K, Li S, Tao K. Labelling stem cells with a nanoprobe for evaluating the homing behaviour in facial nerve injury repair. Biomater Sci. 2022;10(3): 808-818. doi: 10.1039/d1bm01823j
- Zhou G, Tian A, Yi X, et al. Study on a 3D-bioprinted tissue model of self-assembled nanopeptide hydrogels combined with adipose-derived mesenchymal stem cells. Front Bioeng Biotechnol. 2021;9:663120. doi: 10.3389/fbioe.2021.663120
- Lyu J, Hashimoto Y, Honda Y, Matsumoto N. Comparison of osteogenic potentials of dental pulp and bone marrow mesenchymal stem cells using the new cell transplantation platform, CellSaic, in a rat congenital cleft-jaw model. Int J Mol Sci. 2021;22(17). doi: 10.3390/ijms22179478
- Luo L, He Y, Jin L, et al. Application of bioactive hydrogels combined with dental pulp stem cells for the repair of large gap peripheral nerve injuries. Bioact Mater. 2021;6(3): 638-654. doi: 10.1016/j.bioactmat.2020.08.028
- Jiang L, Jones S, Jia X. Stem cell transplantation for peripheral nerve regeneration: current options and opportunities. Int J Mol Sci. 2017;18(1). doi: 10.3390/ijms18010094
- Huang CC, Narayanan R, Alapati S, Ravindran S. Exosomes as biomimetic tools for stem cell differentiation: applications in dental pulp tissue regeneration. Biomaterials. 2016;111:103-115. doi: 10.1016/j.biomaterials.2016.09.029
- Xiong H, Chen K. Multipotent stem cells from apical pulp of human deciduous teeth with immature apex. Tissue Cell. 2021;71:101556. doi: 10.1016/j.tice.2021.101556
- Zhu SY, Yuan CY, Lin YF, et al. Stem cells from human exfoliated deciduous teeth (SHEDs) and dental pulp stem cells (DPSCs) display a similar profile with pericytes. Stem Cells Int. 2021;2021:8859902. doi: 10.1155/2021/8859902
- Wei J, Song Y, Du Z, et al. Exosomes derived from human exfoliated deciduous teeth ameliorate adult bone loss in mice through promoting osteogenesis. J Mol Histol. 2020;51(4):455-466. doi: 10.1007/s10735-020-09896-3
- Pereira LV, Bento RF, Cruz DB, et al. Stem cells from human exfoliated deciduous teeth (SHED) differentiate in vivo and promote facial nerve regeneration. Cell Transplant. 2019;28(1):55-64. doi: 10.1177/0963689718809090
- Sotthibundhu A, Muangchan P, Phonchai R, et al. Autophagy promoted neural differentiation of human placenta-derived mesenchymal stem cells. In Vivo. 2021;35(5):2609-2620. doi: 10.21873/invivo.12543
- Zhang Q, Nguyen P, Burrell JC, et al. Harnessing 3D collagen hydrogel-directed conversion of human GMSCs into SCP-like cells to generate functionalized nerve conduits. NPJ Regen Med. 2021;6(1):59. doi: 10.1038/s41536-021-00170-y
- Kim HS, Lee J, Lee DY, et al. Schwann cell precursors from human pluripotent stem cells as a potential therapeutic target for myelin repair. Stem Cell Reports. 2017;8(6):1714-1726. doi: 10.1016/j.stemcr.2017.04.011
- Rowley JA, Mooney DJ. Alginate type and RGD density control myoblast phenotype. J Biomed Mater Res. 2002;60(2):217-223. doi: 10.1002/jbm.1287
- Bain JR, Mackinnon SE, Hunter DA. Functional evaluation of complete sciatic, peroneal, and posterior tibial nerve lesions in the rat. Plast Reconstr Surg. 1989;83(1): 129-138. doi: 10.1097/00006534-198901000-00024
- Shahidi S, Janmaleki M, Riaz S, Sanati NA, Syed N. A tuned gelatin methacryloyl (GelMA) hydrogel facilitates myelination of dorsal root ganglia neurons in vitro. Mater Sci Eng C Mater Biol Appl. 2021;126:112131. doi: 10.1016/j.msec.2021.112131
- Liu W, Heinrich MA, Zhou Y, et al. Extrusion bioprinting of shear-thinning gelatin methacryloyl bioinks. Adv Healthc Mater. 2017;6(12). doi: 10.1002/adhm.201601451
- Huang Z, Powell R, Phillips JB, Haastert-Talini K. Perspective on Schwann cells derived from induced pluripotent stem cells in peripheral nerve tissue engineering. Cells. 2020;9(11). doi: 10.3390/cells9112497
- Nakamura S, Yamada Y, Katagiri W, Sugito T, Ito K, Ueda M. Stem cell proliferation pathways comparison between human exfoliated deciduous teeth and dental pulp stem cells by gene expression profile from promising dental pulp. J Endod. 2009;35(11):1536-1542. doi: 10.1016/j.joen.2009.07.024
- Ko CS, Chen JH, Su WT. Stem cells from human exfoliated deciduous teeth: a concise review. Curr Stem Cell Res Ther. 2020;15(1):61-76. doi: 10.2174/1574888X14666191018122109
- Nishii T, Osuka K, Nishimura Y, et al. Protective mechanism of stem cells from human exfoliated deciduous teeth in treating spinal cord injury. J Neurotrauma. Published online January 23, 2024. doi: 10.1089/neu.2023.0251
- Martens W, Sanen K, Georgiou M, et al. Human dental pulp stem cells can differentiate into Schwann cells and promote and guide neurite outgrowth in an aligned tissue-engineered collagen construct in vitro. FASEB J. 2014;28(4):1634-1643. doi: 10.1096/fj.13-243980
- Jarmalaviciute A, Tunaitis V, Strainiene E, et al. A new experimental model for neuronal and glial differentiation using stem cells derived from human exfoliated deciduous teeth. J Mol Neurosci. Published online June 26, 2013. doi: 10.1007/s12031-013-0046-0
- Pepelanova I, Kruppa K, Scheper T, Lavrentieva A. Gelatin-methacryloyl (GelMA) hydrogels with defined degree of functionalization as a versatile toolkit for 3D cell culture and extrusion bioprinting. Bioengineering (Basel). 2018;5(3). doi: 10.3390/bioengineering5030055
- Yang GH, Kim W, Kim J, Kim G. A skeleton muscle model using GelMA-based cell-aligned bioink processed with an electric-field assisted 3D/4D bioprinting. Theranostics. 2021;11(1):48-63. doi: 10.7150/thno.50794
- Dong Q, Zhang M, Zhou X, et al. 3D-printed Mg-incorporated PCL-based scaffolds: a promising approach for bone healing. Mater Sci Eng C Mater Biol Appl. 2021;129:112372. doi: 10.1016/j.msec.2021.112372
- Liu C, Wang Z, Wei X, Chen B, Luo Y. 3D printed hydrogel/ PCL core/shell fiber scaffolds with NIR-triggered drug release for cancer therapy and wound healing. Acta Biomater. 2021;131:314-325. doi: 10.1016/j.actbio.2021.07.011
- Du M, Liu S, Lan N, et al. Electrospun PCL/gelatin/arbutin nanofiber membranes as potent reactive oxygen species scavengers to accelerate cutaneous wound healing. Regen Biomater. 2024;11:rbad114. doi: 10.1093/rb/rbad114