Microstructure evolution and ductility improvement of additively manufactured biodegradable zinc–magnesium alloys via annealing
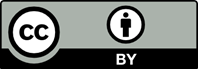
Zinc–magnesium (Zn–Mg) alloys, fabricated by laser powder bed fusion (LPBF) additive manufacturing techniques, have emerged as promising candidates for biomedical implants due to their biodegradation capability, superior mechanical strength, and excellent biocompatibility. However, LPBF-fabricated Zn–Mg alloys still face challenges related to extremely low ductility and limited exploration of degradation characteristics. In this study, the impact of Mg incorporation on the printability, degradation properties, microstructure, and mechanical properties of LPBF-fabricated Zn–Mg alloys was primarily investigated. Furthermore, we proposed a viable annealing post-processing route for the first time to tailor the microstructural characteristics of the fabricated Zn–Mg alloy and enhanced its limited ductility. The results demonstrated that by applying a laser power of 80 W and a scanning speed of 600 mm/s, the relative density of LPBF-fabricated Zn–Mg alloy reached 98.62%. Increasing the Mg amount from 1 to 5 wt% refined the grain size while promoting an increase in Mg2Zn11 and MgZn2 phases. Among these compositions, the Zn–1Mg alloy exhibited the greatest degradation rate at 0.126 mm/year. The annealing treatment facilitated the microstructure evolution of the Zn–1Mg alloy, resulting in equiaxed grains, increased average grain size, high-angle grain boundaries, and enrichment of Mg at grain boundaries. After annealing at 300°C for 0.5 h, the tensile strength of Zn– 1Mg alloy decreased from 254.92 to 170.93 MPa, while the elongation significantly increased by a factor of 14.3 from 0.55% to 8.43%. These findings provide valuable insights into an effective post-processing approach for tailoring the microstructure and resultant mechanical properties of LPBF-fabricated Zn and its alloys.
- Zhao D, Yu K, Sun T, et al. Material–structure–function integrated additive manufacturing of degradable metallic bone implants for load‐bearing applications. Adv Funct Mater. 2023;33(16):2213128. doi: 10.1002/adfm.202213128
- Li H, Wang P, Lin G, Huang J. The role of rare earth elements in biodegradable metals: a review. Acta Biomater. 2021;129: 33-42. doi: 10.1016/j.actbio.2021.05.014
- Shuai C, Zhong S, Shuai Y, Yang W, Peng S, He C. Accelerated anode and cathode reaction due to direct electron uptake and consumption by manganese dioxide and titanium dioxide composite cathode in degradation of iron composite. J Colloid Interface Sci. 2023;632(Pt A):95-107. doi: 10.1016/j.jcis.2022.11.055
- Ling C, Li Q, Zhang Z, et al. Influence of heat treatment on microstructure, mechanical and corrosion behavior of WE43 alloy fabricated by laser-beam powder bed fusion. Int J Extreme Manuf. 2023;6(1):015001. doi: 10.1088/2631-7990/acfad5
- Yang Y, Ling C, Li Y, et al. Microstructure development and biodegradation behavior of additively manufactured Mg-Zn-Gd alloy with LPSO structure. J Mater Sci Technol. 2023;144:1-14. doi: 10.1016/j.jmst.2022.09.059
- Zhou Y, Wang J, Yang Y, et al. Laser additive manufacturing of zinc targeting for biomedical application. Int J Bioprint. 2022;8(1):501. doi: 10.18063/ijb.v8i1.501
- Kabir H, Munir K, Wen C, Li Y. Recent research and progress of biodegradable zinc alloys and composites for biomedical applications: biomechanical and biocorrosion perspectives. Bioact Mater. 2021;6(3):836-879. doi: 10.1016/j.bioactmat.2020.09.013
- Xiao C, Wang L, Ren Y, et al. Indirectly extruded biodegradable Zn-0.05 wt% Mg alloy with improved strength and ductility: in vitro and in vivo studies. J Mater Sci Technol. 2018;34(9):1618-1627. doi: 10.1016/j.jmst.2018.01.006
- Zhang Y, Yan Y, Xu X, et al. Investigation on the microstructure, mechanical properties, in vitro degradation behavior and biocompatibility of newly developed Zn-0.8% Li-(Mg, Ag) alloys for guided bone regeneration. Mater Sci Eng C. 2019;99:1021-1034. doi: 10.1016/j.msec.2019.01.120
- Yang H, Jia B, Zhang Z, et al. Alloying design of biodegradable zinc as promising bone implants for load-bearing applications. Nat Commun. 2020;11(1):401. doi: 10.1038/s41467-019-14153-7
- Su Y, Cockerill I, Wang Y, et al. Zinc-based biomaterials for regeneration and therapy. Trends Biotechnol. 2019;37(4): 428-441. doi: 10.1016/j.tibtech.2018.10.009
- Ran Z, Wang Y, Li J, et al. 3D-printed biodegradable magnesium alloy scaffolds with zoledronic acid-loaded ceramic composite coating promote osteoporotic bone defect repair. Int J Bioprint. 2023;9(5):769. doi: 10.18063/ijb.769
- Venezuela J, Dargusch MS. The influence of alloying and fabrication techniques on the mechanical properties, biodegradability and biocompatibility of zinc: a comprehensive review. Acta Biomater. 2019;87:1-40. doi: 10.1016/j.actbio.2019.01.035
- Kubásek J, Vojtěch D, Jablonská E, Pospíšilová I, Lipov J, Ruml T. Structure, mechanical characteristics and in vitro degradation, cytotoxicity, genotoxicity and mutagenicity of novel biodegradable Zn–Mg alloys. Mat Sci Eng C-Mater. 2016;58:24-35. doi: 10.1016/j.msec.2015.08.015
- Kubásek J, Vojtěch D, Pospíšilová I, Michalcová A, Maixner J. Microstructure and mechanical properties of the micrograined hypoeutectic Zn–Mg alloy. Int J Min Met Mater. 2016;23:1167-1176. doi: 10.1007/s12613-016-1336-7
- Dong Z, Han C, Zhao Y, et al. Role of heterogenous microstructure and deformation behavior in achieving superior strength-ductility synergy in zinc fabricated via laser powder bed fusion. Int J Extreme Manuf. 2024;6(4):045003 do: 10.1088/2631-7990/ad3929
- Shuai C, Li D, Yao X, Li X, Gao C. Additive manufacturing of promising heterostructure for biomedical applications. Int J Extreme Manuf. 2023;5:032012. doi: 10.1088/2631-7990/acded2
- Zhao D, Han C, Peng B, et al. Corrosion fatigue behavior and anti-fatigue mechanisms of an additively manufactured biodegradable zinc-magnesium gyroid scaffold. Acta Biomater. 2022;153:614-629. doi: 10.1016/j.actbio.2022.09.047
- Zhou K, Han C. Metal Powder-Based Additive Manufacturing. John Wiley & Sons; 2023. doi: 10.1002/9783527822249.ch1
- Bandyopadhyay A, Ciliveri S, Guariento S, Zuckschwerdt N, Hogg WW. Fatigue behavior of additively manufactured Ti3Al2V alloy. Mater Sci Addit Manuf. 2023;2(3): 1705. doi: 10.36922/msam.1705
- Qin Y, Wen P, Guo H, et al. Additive manufacturing of biodegradable metals: current research status and future perspectives. Acta Biomater. 2019;98:3-22. doi: 10.1016/j.actbio.2019.04.046
- Gao C, Li C, Peng S, Shuai C. Spiral-eutectic-reinforced biodegradable Zn–Mg–Ag alloy prepared via selective laser melting. Chin J Mech Eng Addit Manuf Fron. 2022;1(2):100022. doi: 10.1016/j.cjmeam.2022.100022
- Wang C, Hu Y, Zhong C, Lan C, Li W, Wang X. Microstructural evolution and mechanical properties of pure Zn fabricated by selective laser melting. Mat Sci Eng: A. 2022;846:143276. doi: 10.1016/j.msea.2022.143276
- Liu J, Wen P. Metal vaporization and its influence during laser powder bed fusion process. Mater Des. 2022;215: 110505. doi: 10.1016/j.matdes.2022.110505
- Ning J, Ma Z, Zhang L, Wang D, Na S. Effects of magnesium on microstructure, properties and degradation behaviors of zinc-based alloys prepared by selective laser melting. Mater Res Express. 2022;9(8):086511. doi: 10.1088/2053-1591/ac88b7
- Yang Y, Yuan F, Gao C, et al. A combined strategy to enhance the properties of Zn by laser rapid solidification and laser alloying. J Mech Behav Biomed Mater. 2018;82:51-60. doi: 10.1016/j.jmbbm.2018.03.018
- Qin Y, Liu A, Guo H, et al. Additive manufacturing of Zn- Mg alloy porous scaffolds with enhanced osseointegration: in vitro and in vivo studies. Acta Biomater. 2022;145: 403-415. doi: 10.1016/j.actbio.2022.03.055
- Bai J, Xu Y, Fan Q, et al. Mechanical properties and degradation behaviors of Zn-xMg alloy fine wires for biomedical applications. Scanning. 2021;2021:4831387. doi: 10.1155/2021/4831387
- Dambatta MS, Izman S, Kurniawan D, Farahany S, Yahaya B, Hermawan H. Influence of thermal treatment on microstructure, mechanical and degradation properties of Zn–3Mg alloy as potential biodegradable implant material. Mater Des. 2015;85:431-437. doi: 10.1016/j.matdes.2015.06.181
- Chua C, Sing SL, Chua CK. Characterisation of in-situ alloyed titanium-tantalum lattice structures by laser powder bed fusion using finite element analysis. Virtual Phys Prototyp. 2023;18(1):e2138463. doi: 10.1080/17452759.2022.2138463
- Yao C, Wang Z, Tay SL, Zhu T, Gao W. Effects of Mg on microstructure and corrosion properties of Zn–Mg alloy. J Alloys Compd. 2014;602:101-107. doi: 10.1016/j.jallcom.2014.03.025
- Dambatta MS, Izman S, Hermawan H, Kurniawan D. Influence of heat treatment cooling mediums on the degradation property of biodegradable Zn-3Mg alloy. Adv Mat Res. 2014;845:7-11. doi: 10.4028/www.scientific.net/AMR.845.7
- Dambatta MS, Izman S, Hermawan H, Kurniawan D. Effect of heat treatment on microstructure homogeneity of Zn-3Mg alloy. Appl Mech Mater. 2014;493: 777-782. doi: 10.4028/www.scientific.net/AMM.493.777
- Cui L, Zhang Z, Chen X. Microstructure and mechanical properties of novel Al–Cu–Mg–Zn lightweight entropy alloys for elevated-temperature applications. Mater Charact. 2023;200:112927. doi: 10.1016/j.matchar.2023.112927
- Yang Y, Lu C, Shen L, Zhao Z, Peng S, Shuai C. In-situ deposition of apatite layer to protect Mg-based composite fabricated via laser additive manufacturing. J Magnes Alloy. 2023;11(2):629-640. doi: 10.1016/j.jma.2021.04.009
- Parsons EM, Shaik SZ. Additive manufacturing of aluminum metal matrix composites: mechanical alloying of composite powders and single track consolidation with laser powder bed fusion. Addit Manuf. 2022;50:102450. doi: 10.1016/j.addma.2021.102450
- Bouabbou A, Vaudreuil S. Understanding laser-metal interaction in selective laser melting additive manufacturing through numerical modelling and simulation: a review. Virtual Phys Prototyp. 2022;17(3):543-562. doi: 10.1080/17452759.2022.2052488
- Zheng YF, Gu XN, Witte F. Biodegradable metals. Mat Sci Eng R. 2014;77:1-34. doi: 10.1016/j.mser.2014.01.001
- Ye L, Huang H, Sun C, et al. Effect of grain size and volume fraction of eutectic structure on mechanical properties and corrosion behavior of as-cast Zn–Mg binary alloys. J Mater Res Technol. 2022;16:1673-1685. doi: 10.1016/j.jmrt.2021.12.101
- Gao C, Li S, Liu L, et al. Dual alloying improves the corrosion resistance of biodegradable Mg alloys prepared by selective laser melting. J Magnes Alloy. 2021;9(1):305-316. doi: 10.1016/j.jma.2020.03.016
- Hosking NC, Ström MA, Shipway PH, Rudd CD. Corrosion resistance of zinc–magnesium coated steel. Corros Sci. 2007;49(9):3669-3695. doi: 10.1016/j.corsci.2007.03.032
- Prosek T, Thierry D, Taxén C, Maixner J. Effect of cations on corrosion of zinc and carbon steel covered with chloride deposits under atmospheric conditions. Corros Sci. 2007;49(6):2676-2693. doi: 10.1016/j.corsci.2006.11.004
- Hausbrand R, Stratmann M, Rohwerder M. Corrosion of zinc–magnesium coatings: mechanism of paint delamination. Corros Sci. 2009;51(9):2107-2114. doi: 10.1016/j.corsci.2009.05.042
- Falk T, Svensson JE, Johansson LG. The influence of CO2 and NaCl on the atmospheric corrosion of zinc: a laboratory study. J Electrochem Soc. 1998;145(9):2993. doi: 10.1149/1.1838753
- Ralston KD, Birbilis N, Davies C. Revealing the relationship between grain size and corrosion rate of metals. Scr Mater. 2010;63(12):1201-1204. doi: 10.1016/j.scriptamat.2010.08.035
- Pachla W, Przybysz S, Jarzębska A, et al. Structural and mechanical aspects of hypoeutectic Zn–Mg binary alloys for biodegradable vascular stent applications. Bioact Mater. 2021;6(1):26-44. doi: 10.1016/j.bioactmat.2020.07.004
- Li HF, Xie XH, Zheng YF, et al. Development of biodegradable Zn-1X binary alloys with nutrient alloying elements Mg, Ca and Sr. Sci Rep. 2015;5(1):10719. doi: 10.1038/srep10719
- García-Mintegui C, Córdoba LC, Buxadera-Palomero J, et al. Zn-Mg and Zn-Cu alloys for stenting applications: from nanoscale mechanical characterization to in vitro degradation and biocompatibility. Bioact Mater. 2021;6(12):4430-4446. doi: 10.1016/j.bioactmat.2021.04.015