Bioprinted autologous human skin equivalents for in vitro testing of therapeutic antibodies
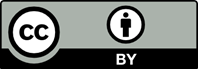
In recent years, advances in tissue engineering have brought forward the accessibility of human skin equivalents for in vitro applications; however, the availability of human-based engineered tissue models suitable for high-throughput screening of biologics remains limited. Here, we report a method of manufacturing fully autologous (with both fibroblasts and keratinocytes from the same donor) human skin equivalents for determining preclinical therapeutic antibody adverse immune reactions in vitro. Using a combination of precise solenoid microvalve-based bioprinting and 96-well scale Alvetex inserts, autologous skin cells were bioprinted and cultured to develop a scalable approach to manufacturing skin equivalents. We demonstrated that fibroblasts and keratinocytes can be bioprinted with a high degree of precision while maintaining viability post printing. Histological staining showed that the bioprinted 96-well based skin equivalents were comparable to human skin. The fully autologous human skin equivalents were co-cultured in vitro with autologous peripheral blood monocytes with and without muromonab-CD3 (OKT3) and natalizumab (Tysabri), biologics which are known to cause and inhibit adverse immune reactions (type IV hypersensitivity), respectively. Analysis of supernatants from skin-equivalent monocyte co-cultures revealed significant proinflammatory cytokine responses (such as interferon gamma) in co-cultures treated with OKT3 when compared to Tysabri and negative controls. Consequently, this study provides proof of concept that through a combination of bioprinting and Alvetex scaffold-based culture systems, scalable human skin equivalents can be manufactured for high-throughput identification of adverse immune reactions during preclinical stages of the drug development process.
- Stebbings R, Findlay L, Edwards C, et al. “Cytokine storm” in the phase I trial of monoclonal antibody TGN1412: better understanding the causes to improve preclinical testing of immunotherapeutics. J Immunol. 2007;179(5):3325-3331. doi: 10.4049/jimmunol.179.5.3325
- Hay M, Thomas D, Craighead J, Economides C, Rosenthal J. Clinical development success rates for investigational drugs. Nat Biotechnol. 2014;32:40-51. doi: 10.1038/nbt.2786
- Pound P, Ritskes-Hoitinga M. Is it possible to overcome issues of external validity in preclinical animal research? Why most animal models are bound to fail. J Transl Med. 2018;16:304. doi: 10.1186/s12967-018-1678-1
- Panoskaltsis N, McCarthy NE, Knight SC. Myelopoiesis of acute inflammation: lessons from TGN1412-induced cytokine storm. Cancer Immunol Immunother. 2021;70:1155-1160. doi: 10.1007/s00262-020-02702-9
- DiMasi JA, Grabowski HG, Hansen RW. Innovation in the pharmaceutical industry: new estimates of R&D costs. J Health Econ. 2016;47:20-33. doi: 10.1016/j.jhealeco.2016.01.012
- Mahlich J, Bartol A, Dheban S. Can adaptive clinical trials help to solve the productivity crisis of the pharmaceutical industry? - a scenario analysis. Health Econ Rev. 2021;11:4. doi: 10.1186/s13561-021-00302-6
- Hughes JP, Rees S, Kalindjian SB, Philpott KL. Principles of early drug discovery. Br J Pharmacol. 2011;162(6):1239-1249. doi: 10.1111/j.1476-5381.2010.01127.x
- Ahmed SS, Whritenour J, Ahmed MM, et al. Evaluation of a human in vitro skin test for predicting drug hypersensitivity reactions. Toxicol Appl Pharmacol. 2019;369:39-48. doi: 10.1016/j.taap.2019.02.005
- Lawrence E, Sims J, Gander A, et al. The barriers and motivators to using human tissues for research: the views of UK-based biomedical researchers. Biopreserv Biobank. 2020;18(4):266-273. doi: 10.1089/bio.2019.0138
- Harley WS, Li CC, Toombs J, et al. Advances in biofabrication techniques towards functional bioprinted heterogeneous engineered tissues: a comprehensive review. Bioprinting. 2021;23:e00147. doi: 10.1016/j.bprint.2021.e00147
- di Marzio N, Eglin D, Serra T, Moroni L. Bio-Fabrication: convergence of 3D bioprinting and nano-biomaterials in tissue engineering and regenerative medicine. Front Bioeng Biotechnol. 2020;8. doi: 10.3389/fbioe.2020.00326
- Groll J, Boland T, Blunk T, et al. Biofabrication: reappraising the definition of an evolving field. Biofabrication. 2016;8(1):013001. doi: 10.1088/1758-5090/8/1/013001
- Koch L, Deiwick A, Franke A, et al. Laser bioprinting of human induced pluripotent stem cells-the effect of printing and biomaterials on cell survival, pluripotency, and differentiation. Biofabrication. 2018;10(3):035005. doi: 10.1088/1758-5090/aab981
- Cubo N, Garcia M, Del Cañizo JF, Velasco D, Jorcano JL. 3D bioprinting of functional human skin: production and in vivo analysis. Biofabrication. 2016;9(1):015006. doi: 10.1088/1758-5090/9/1/015006
- Murphy S, Atala A. 3D bioprinting of tissues and organs. Nat Biotechnol. 2014;32:773-785. doi: 10.1038/nbt.2958
- Solis LH, Ayala Y, Portillo S, Varela-Ramirez A, Aguilera R, Boland T. Thermal inkjet bioprinting triggers the activation of the VEGF pathway in human microvascular endothelial cells in vitro. Biofabrication. 2019;11(4):045005. doi: 10.1088/1758-5090/ab25f9
- Dudman JPR, Ferreira AM, Gentile P, et al. Reliable inkjet printing of chondrocytes and MSCs using reservoir agitation. Biofabrication. 2020;12(4):045024. doi: 10.1088/1758-5090/aba2f8
- Li X, Liu B, Pei B, et al. Inkjet bioprinting of biomaterials. Chem Rev. 2020;120(19):10793-10833. doi: 10.1021/acs.chemrev.0c00008
- Faulkner-Jones A, Fyfe C, Cornelissen DJ, et al. Bioprinting of human pluripotent stem cells and their directed differentiation into hepatocyte-like cells for the generation of mini-livers in 3D. Biofabrication. 2015;7(4):044102. doi: 10.1088/1758-5090/7/4/044102
- Dudman J, Ferreira AM, Gentile P, Wang X, Dalgarno K. Microvalve bioprinting of MSC-chondrocyte co-cultures. Cells. 2021;10(12):3329. doi: 10.3390/cells10123329
- Ng WL, Qi JTZ, Yeong WY, Naing MW. Proof-of-concept: 3D bioprinting of pigmented human skin constructs. Biofabrication. 2018;10(2):025005. doi: 10.1088/1758-5090/aa9e1e
- Yang X, Lu Z, Wu H, Li W, Zheng L, Zhao J. Collagen-alginate as bioink for three-dimensional (3D) cell printing based cartilage tissue engineering. Mater Sci Eng C. 2018;83:195-201. doi: 10.1016/j.msec.2017.09.002
- Fedorovich NE, Kuipers E, Gawlitta D, Dhert WJA, Alblas J. Scaffold porosity and oxygenation of printed hydrogel constructs affect functionality of embedded osteogenic progenitors. Tissue Eng Part A. 2011;17(19-20):2473-2486. doi: 10.1089/ten.TEA.2011.0001
- Alonzo M, AnilKumar S, Roman B, Tasnim N, Joddar B. 3D bioprinting of cardiac tissue and cardiac stem cell therapy. Transl Res. 2019;211:64-83. doi: 10.1016/j.trsl.2019.04.004
- Albanna M, Binder KW, Murphy SV, et al. In situ bioprinting of autologous skin cells accelerates wound healing of extensive excisional full-thickness wounds. Sci Rep. 2019;9(1):1856. doi: 10.1038/s41598-018-38366-w
- Zhou Y, Qin R, Chen T, Zhang K, Gui J. 3D bioprinting modified autologous matrix-induced chondrogenesis (AMIC) technique for repair of cartilage defects. Mater Des. 2021;203. doi: 10.1016/j.matdes.2021.109621
- Hill DS, Robinson ND, Caley MP, et al. A novel fully humanized 3D skin equivalent to model early melanoma invasion. Mol Cancer Ther. 2015;14(11):2665-2673. doi: 10.1158/1535-7163.MCT-15-0394
- Choudhury S, Das A. Advances in generation of three-dimensional skin equivalents: pre-clinical studies to clinical therapies. Cytotherapy. 2021;23(1):P1-9. doi: 10.1016/j.jcyt.2020.10.001
- Berger A. Th1 and Th2 responses: what are they? BMJ. 2000;321:424. doi: 10.1136/bmj.321.7258.424
- Swain SL, Weinberg AD, English M, Huston G. IL-4 directs the development of Th2-like helper effectors. J Immunol. 1990;145(11):3796-3806.
- Gaston RS, Deierhoi MH, Patterson T, et al. OKT3 first-dose reaction: association with T cell subsets and cytokine release. Kidney Int. 1991;39(1):141-148. doi: 10.1038/ki.1991.18
- Römer PS, Berr S, Avota E, et al. Preculture of PBMCs at high cell density increases sensitivity of T-cell responses, revealing cytokine release by CD28 superagonist TGN1412. Blood. 2011;118(26):6772-6782. doi: 10.1182/blood-2010-12-319780
- Yoshizaki, K, Nishimoto N, Matsumoto K, et al. Interleukin 6 and expression of its receptor on epidermal keratinocytes. Cytokine. 1990;2(5):381-387. doi: 10.1016/1043-4666(90)90069-6
- Mazzocchi A, Soker S, Skardal A. 3D bioprinting for high-throughput screening: drug screening, disease modeling, and precision medicine applications. Appl Phys Rev. 2019;6(1):011302. doi: 10.1063/1.5056188
- Mandrycky C, Wang Z, Kim K, Kim DH. 3D bioprinting for engineering complex tissues. Biotechnol Adv. 2016;34(4): 422-434. doi: 10.1016/j.biotechadv.2015.12.011
- Lee V, Singh G, Trasatti JP, et al. Design and fabrication of human skin by three-dimensional bioprinting. Tissue Eng Part C. 2014;20(6):473-484. doi: 10.1089/ten.TEC.2013.0335
- Michael S, Sorg H, Peck CT, et al. Tissue engineered skin substitutes created by laser-assisted bioprinting form skin-like structures in the dorsal skin fold chamber in mice. PLoS One. 2013;8(3):e57741. doi: 10.1371/journal.pone.0057741
- Weng T, Zhang W, Xia Y, et al. 3D bioprinting for skin tissue engineering: current status and perspectives. J Tissue Eng. 2021;12:20417314211028574. doi: 10.1177/20417314211028574