Potential of bioprinted intestine-on-chip models in advancing understanding of human coronavirus infections and drug screening
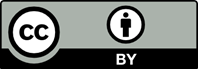
Human coronaviruses, including severe acute respiratory syndrome coronavirus 2 (SARS-CoV-2), contribute to both respiratory and gastrointestinal symptoms, necessitating a comprehensive approach to studying viral pathogenesis. In this context, bioprinted intestine-on-chip models offer a cutting-edge technology for closely replicating the tissue architecture and microenvironment of the human intestine, providing valuable insights into viral dynamics and host responses. Integration of intestinal organoids with organoid-on-chip technology enhances the accuracy of modeling SARS-CoV-2 infection by means of improving cellular differentiation and virus-binding receptor expression. Furthermore, bioprinting technology allows for automated fabrication, enabling high-throughput drug screening on the intestine-on-chip platform. These advancements in bioprinted intestine-on-chip models hold immense promise for advancing our understanding of coronavirus infection in the gut and accelerating drug development, ultimately contributing to improved patient outcomes and public health measures.
- Han H, Jang J. Recent advances in biofabricated gut models to understand the gut-brain axis in neurological diseases. Front Med Technol. 2022;4:931411. doi: 10.3389/fmedt.2022.931411
- Prashantha K, Krishnappa A, Muthappa M. 3D bioprinting of gastrointestinal cancer models: a comprehensive review on processing, properties, and therapeutic implications. Biointerphases. 2023;18(2):020801. doi: 10.1116/6.0002372
- Zimmerling A, Chen X. Bioprinting for combating infectious diseases. Bioprinting. 2020;20:e00104. doi: 10.1016/j.bprint.2020.e00104
- Yi H-G, Kim H, Kwon J, Choi Y-J, Jang J, Cho D-W. Application of 3D bioprinting in the prevention and the therapy for human diseases. Signal Transduct Target Ther. 2021;6(1):177. doi: 10.1038/s41392-021-00566-8
- V’kovski P, Kratzel A, Steiner S, Stalder H, Thiel V. Coronavirus biology and replication: implications for SARS-CoV-2. Nat Rev Microbiol. 2021;19(3):155-170. doi: 10.1038/s41579-020-00468-6
- Luo X, Zhou G-Z, Zhang Y, Peng L-H, Zou L-P, Yang Y-S. Coronaviruses and gastrointestinal diseases. Mil Med Res. 2020;7(1):49. doi: 10.1186/s40779-020-00279-z
- Vabret A, Dina J, Gouarin S, et al. Human (non-severe acute respiratory syndrome) coronavirus infections in hospitalised children in France. J Paediatr Child Health. 2008;44(4): 176-181. doi: 10.1111/j.1440-1754.2007.01246.x
- Assiri A, Al-Tawfiq JA, Al-Rabeeah AA, et al. Epidemiological, demographic, and clinical characteristics of 47 cases of Middle East respiratory syndrome coronavirus disease from Saudi Arabia: a descriptive study. Lancet Infect Dis. 2013;13(9):752-761. doi: 10.1016/S1473-3099(13)70204-4
- Guo M, Tao W, Flavell RA, Zhu S. Potential intestinal infection and faecal–oral transmission of SARS-CoV-2. Nat Rev Gastroenterol Hepatol. 2021;18(4):269-283. doi: 10.1038/s41575-021-00416-6
- Chen Y, Chen L, Deng Q, et al. The presence of SARS-CoV-2 RNA in the feces of COVID-19 patients. J Med Virol. 2020;92(7):833-840. doi: 10.1002/jmv.25825
- Zhang F, Lau RI, Liu Q, Su Q, Chan FKL, Ng SC. Gut microbiota in COVID-19: key microbial changes, potential mechanisms and clinical applications. Nat Rev Gastroenterol Hepatol. 2023;20(5):323-337. doi: 10.1038/s41575-022-00698-4
- Hashimoto T, Perlot T, Rehman A, et al. ACE2 links amino acid malnutrition to microbial ecology and intestinal inflammation. Nature. 2012;487(7408):477-481. doi: 10.1038/nature11228
- Viana SD, Nunes S, Reis F. ACE2 imbalance as a key player for the poor outcomes in COVID-19 patients with age-related comorbidities – role of gut microbiota dysbiosis. Ageing Res Rev. 2020;62:101123. doi: 10.1016/j.arr.2020.101123
- Taelman J, Diaz M, Guiu J. Human intestinal organoids: promise and challenge. Front Cell Dev Biol. 2022;10:854740. doi: 10.3389/fcell.2022.854740
- Zachos NC, Kovbasnjuk O, Foulke-Abel J, et al. Human enteroids/colonoids and intestinal organoids functionally recapitulate normal intestinal physiology and pathophysiology. J Biol Chem. 2016;291(8):3759-3766. doi: 10.1074/jbc.R114.635995
- Sato T, Vries RG, Snippert HJ, et al. Single Lgr5 stem cells build crypt-villus structures in vitro without a mesenchymal niche. Nature. 2009;459(7244):262-265. doi: 10.1038/nature07935
- Sato T, Stange DE, Ferrante M, et al. Long-term expansion of epithelial organoids from human colon, adenoma, adenocarcinoma, and Barrett’s epithelium. Gastroenterology. 2011;141(5):1762-1772. doi: 10.1053/j.gastro.2011.07.050
- Onozato D, Ogawa I, Kida Y, et al. Generation of budding-like intestinal organoids from human induced pluripotent stem cells. J Pharm Sci. 2021;110(7):2637-2650. doi: 10.1016/j.xphs.2021.03.014
- Zhao Z, Chen X, Dowbaj AM, et al. Organoids. Nat Rev Methods Primers. 2022;2(1):94. doi: 10.1038/s43586-022-00174-y
- Frum T, Spence JR. hPSC-derived organoids: models of human development and disease. J Mol Med. 2021;99(4): 463-473. doi: 10.1007/s00109-020-01969-w
- Puschhof J, Pleguezuelos-Manzano C, Martinez-Silgado A, et al. Intestinal organoid cocultures with microbes. Nat Protoc. 2021;16(10):4633-4649. doi: 10.1038/s41596-021-00589-z
- Crawford SE, Ramani S, Blutt SE, Estes MK. Organoids to dissect gastrointestinal virus–host interactions: what have we learned? Viruses. 2021;13(6):999. doi: 10.3390/v13060999
- Heo I, Dutta D, Schaefer DA, et al. Modelling cryptosporidium infection in human small intestinal and lung organoids. Nat Microbiol. 2018;3(7):814-823. doi: 10.1038/s41564-018-0177-8
- Bartfeld S, Bayram T, van de Wetering M, et al. In vitro expansion of human gastric epithelial stem cells and their responses to bacterial infection. Gastroenterology. 2015;148(1):126-136.e6. doi: 10.1053/j.gastro.2014.09.042
- Aguirre Garcia M, Hillion K, Cappelier J-M, Neunlist M, Mahe MM, Haddad N. Intestinal organoids: new tools to comprehend the virulence of bacterial foodborne pathogens. Foods. 2022;11(1):108. doi: 10.3390/foods11010108
- Aguilar C, Alves da Silva M, Saraiva M, Neyazi M, Olsson IAS, Bartfeld S. Organoids as host models for infection biology – a review of methods. Exp Mol Med. 2021;53(10): 1471-1482. doi: 10.1038/s12276-021-00629-4
- Co JY, Margalef-Català M, Monack DM, Amieva MR. Controlling the polarity of human gastrointestinal organoids to investigate epithelial biology and infectious diseases. Nat Protoc. 2021;16(11):5171-5192. doi: 10.1038/s41596-021-00607-0
- Han X, Mslati MA, Davies E, Chen Y, Allaire JM, Vallance BA. Creating a more perfect union: modeling intestinal bacteria-epithelial interactions using organoids. Cell Mol Gastroenterol Hepatol. 2021;12(2):769-782. doi: 10.1016/j.jcmgh.2021.04.010
- Holly MK, Smith JG. Adenovirus infection of human enteroids reveals interferon sensitivity and preferential infection of goblet cells. J Virol. 2018;92(9):e00250-18. doi: 10.1128/JVI.00250-18
- Lamers MM, Beumer J, van der Vaart J, et al. SARS-CoV-2 productively infects human gut enterocytes. Science. 2020;369(6499):50-54. doi: 10.1126/science.abc1669
- Zhou J, Li C, Liu X, et al. Infection of bat and human intestinal organoids by SARS-CoV-2. Nat Med. 2020;26(7):1077-1083. doi: 10.1038/s41591-020-0912-6
- Stanifer ML, Kee C, Cortese M, et al. Critical role of type III interferon in controlling SARS-CoV-2 infection in human intestinal epithelial cells. Cell Rep. 2020;32(1):107863. doi: 10.1016/j.celrep.2020.107863
- Beumer J, Geurts MH, Lamers MM, et al. A CRISPR/ Cas9 genetically engineered organoid biobank reveals essential host factors for coronaviruses. Nat Commun. 2021;12(1):5498. doi: 10.1038/s41467-021-25729-7
- Zang R, Castro MFG, McCune BT, et al. TMPRSS2 and TMPRSS4 promote SARS-CoV-2 infection of human small intestinal enterocytes. Sci Immunol. 2020;5(47):eabc3582. doi: 10.1126/sciimmunol.abc3582
- Triana S, Metz-Zumaran C, Ramirez C, et al. Single-cell analyses reveal SARS-CoV-2 interference with intrinsic immune response in the human gut. Mol Syst Biol. 2021;17(4):e10232. doi: 10.15252/msb.202110232
- Zhou J, Li C, Zhao G, et al. Human intestinal tract serves as an alternative infection route for Middle East respiratory syndrome coronavirus. Sci Adv. 2017;3(11):eaao4966. doi: 10.1126/sciadv.aao4966
- Wang Y, Li P, Lavrijsen M, et al. Immunosuppressants exert differential effects on pan-coronavirus infection and distinct combinatory antiviral activity with molnupiravir and nirmatrelvir. United European Gastroenterol J. 2023;11(5):431-447. doi: 10.1002/ueg2.12417
- Li J, Wang Y, Solanki K, et al. Nirmatrelvir exerts distinct antiviral potency against different human coronaviruses. Antiviral Res. 2023;211:105555. doi: 10.1016/j.antiviral.2023.105555
- Li P, Wang Y, Lamers MM, et al. Recapitulating infection, thermal sensitivity and antiviral treatment of seasonal coronaviruses in human airway organoids. eBioMedicine. 2022;81:104132. doi: 10.1016/j.ebiom.2022.104132
- Hashimoto R, Tamura T, Watanabe Y, et al. Evaluation of broad anti-coronavirus activity of autophagy-related compounds using human airway organoids. Mol Pharmaceutics. 2023;20(4):2276-2287. doi: 10.1021/acs.molpharmaceut.3c00114
- Calistri A, Luganini A, Mognetti B, et al. The new generation hDHODH inhibitor MEDS433 hinders the in vitro replication of SARS-CoV-2 and other human coronaviruses. Microorganisms. 2021;9(8):1731. doi: 10.3390/microorganisms9081731
- Kim M-H, van Noort D, Sung JH, Park S. Organ-on-a-chip for studying gut-brain interaction mediated by extracellular vesicles in the gut microenvironment. Int J Mol Sci. 2021;22(24):13513. doi: 10.3390/ijms222413513
- Leung CM, de Haan P, Ronaldson-Bouchard K, et al. A guide to the organ-on-a-chip. Nat Rev Methods Primers. 2022;2(1):33. doi: 10.1038/s43586-022-00118-6
- Xian C, Zhang J, Zhao S, Li X-G. Gut-on-a-chip for disease models. J Tissue Eng. 2023;14:20417314221149882. doi: 10.1177/20417314221149882
- Fois CAM, Le TYL, Schindeler A, et al. Models of the gut for analyzing the impact of food and drugs. Adv Healthc Mater. 2019;8:1900968. doi: 10.1002/adhm.201900968
- Ensari A, Marsh MN. Exploring the villus. Gastroenterol Hepatol Bed Bench. 2018;11:181-190.
- Parker A, Maclaren OJ, Fletcher AG, et al. Cell proliferation within small intestinal crypts is the principal driving force for cell migration on villi. FASEB J. 2017;31:636-649. doi: 10.1096/fj.201601002
- Sommer F, Bäckhed F. Know your neighbor: microbiota and host epithelial cells interact locally to control intestinal function and physiology. BioEssays. 2016;38:455-464. doi: 10.1002/bies.201500151
- Kim SH, Chi M, Yi B, et al. Three-dimensional intestinal villi epithelium enhances protection of human intestinal cells from bacterial infection by inducing mucin expression. Integr Biol. 2014;6:1122-1131. doi: 10.1039/C4IB00157E
- Fois CAM, Schindeler A, Valtchev P, Dehghani F. Dynamic flow and shear stress as key parameters for intestinal cells morphology and polarization in an organ-on-a-chip model. Biomed Microdevices. 2021;23:55. doi: 10.1007/s10544-021-00591-y
- Delon LC, Guo Z, Oszmiana A, et al. A systematic investigation of the effect of the fluid shear stress on Caco- 2 cells towards the optimization of epithelial organ-on-chip models. Biomaterials. 2019;225:119521. doi: 10.1016/j.biomaterials.2019.119521
- Chi M, Yi B, Oh S, Park D-J, Sung JH, Park S. A microfluidic cell culture device (μFCCD) to culture epithelial cells with physiological and morphological properties that mimic those of the human intestine. Biomed Microdevices. 2015;17:58. doi: 10.1007/s10544-015-9966-5
- Gayer CP, Basson MD. The effects of mechanical forces on intestinal physiology and pathology. Cell Signal. 2009;21:1237-1244. doi: 10.1016/j.cellsig.2009.02.011
- Feaugas T, Sauvonnet N. Organ-on-chip to investigate host-pathogens interactions. Cell Microbiol. 2021;23:e13336. doi: 10.1111/cmi.13336
- Grassart A, Malardé V, Gobaa S, et al. Bioengineered human organ-on-chip reveals intestinal microenvironment and mechanical forces impacting shigella infection. Cell Host Microbe. 2019;26:435-444.e4. doi: 10.1016/j.chom.2019.08.007
- Kim HJ, Huh D, Hamilton G, Ingber DE. Human gut-on-a-chip inhabited by microbial flora that experiences intestinal peristalsis-like motions and flow. Lab Chip. 2012;12(12):2165-2174. doi: 10.1039/C2LC40074J
- Tovaglieri A, Sontheimer-Phelps A, Geirnaert A, et al. Species-specific enhancement of enterohemorrhagic E. coli pathogenesis mediated by microbiome metabolites. Microbiome. 2019;7(1):43. doi: 10.1186/s40168-019-0650-5
- Wang Y, Wang P, Qin J. Human organoids and organs-on-chips for addressing COVID-19 challenges. Adv Sci. 2022;9(10):2105187. doi: 10.1002/advs.202105187
- Tang H, Abouleila Y, Si L, et al. Human organs-on-chips for virology. Trends Microbiol. 2020;28(11):934-946. doi: 10.1016/j.tim.2020.06.005
- Ortega-Prieto AM, Skelton JK, Wai SN, et al. 3D microfluidic liver cultures as a physiological preclinical tool for hepatitis B virus infection. Nat Commun. 2018;9(1):682. doi: 10.1038/s41467-018-02969-8
- Wang J, Wang C, Xu N, Liu Z-F, Pang D-W, Zhang Z-L. A virus-induced kidney disease model based on organ-on-a-chip: pathogenesis exploration of virus-related renal dysfunctions. Biomaterials. 2019;219:119367. doi: 10.1016/j.biomaterials.2019.119367
- Han Y, Yang L, Lacko LA, Chen S. Human organoid models to study SARS-CoV-2 infection. Nat Methods. 2022;19(4):418-428. doi: 10.1038/s41592-022-01453-y
- Zhang M, Wang P, Luo R, et al. Biomimetic human disease model of SARS-CoV-2-induced lung injury and immune responses on organ chip system. Adv Sci. 2021;8(3):2002928. doi: 10.1002/advs.202002928
- Deguchi S, Kosugi K, Hashimoto R, et al. Elucidation of the liver pathophysiology of COVID-19 patients using liver-on-a-chips. PNAS Nexus. 2023;2(3):pgad029. doi: 10.1093/pnasnexus/pgad029
- Lu RXZ, Lai BFL, Rafatian N, et al. Vasculature-on-a-chip platform with innate immunity enables identification of angiopoietin-1 derived peptide as a therapeutic for SARS-CoV-2 induced inflammation. Lab Chip. 2022;22(6):1171-1186. doi: 10.1039/D1LC00817J
- Villenave R, Wales SQ, Hamkins-Indik T, et al. Human gut-on-a-chip supports polarized infection of Coxsackie B1 virus in vitro. PLOS ONE. 2017;12(2):e0169412. doi: 10.1371/journal.pone.0169412
- Bein A, Kim S, Goyal G, et al. Enteric coronavirus infection and treatment modeled with an immunocompetent human intestine-on-a-chip. Front Pharmacol. 2021;12:718484. doi: 10.3389/fphar.2021.718484
- Hikmet F, Méar L, Edvinsson Å, Micke P, Uhlén M, Lindskog C. The protein expression profile of ACE2 in human tissues. Mol Syst Biol. 2020;16(7):e9610. doi: 10.15252/msb.20209610
- Guo Y, Luo R, Wang Y, et al. SARS-CoV-2 induced intestinal responses with a biomimetic human gut-on-chip. Sci Bull. 2021;66(8):783-793. doi: 10.1016/j.scib.2020.11.015
- Yeager CL, Ashmun RA, Williams RK, et al. Human aminopeptidase N is a receptor for human coronavirus 229E. Nature. 1992;357(6377):420-422. doi: 10.1038/357420a0
- Tobey N, Heizer W, Yeh R, Huang T-I, Hoffner C. Human intestinal brush border peptidases. Gastroenterology. 1985;88(4):913-926. doi: 10.1016/S0016-5085(85)80008-1
- Shim K-Y, Lee D, Han J, Nguyen N-T, Park S, Sung JH. Microfluidic gut-on-a-chip with three-dimensional villi structure. Biomed Microdevices. 2017;19(2):37. doi: 10.1007/s10544-017-0179-y
- Tan H-Y, Trier S, Rahbek UL, Dufva M, Kutter JP, Andresen TL. A multi-chamber microfluidic intestinal barrier model using Caco-2 cells for drug transport studies. PLOS ONE. 2018;13(5):e0197101. doi: 10.1371/journal.pone.0197101
- Park SE, Georgescu A, Huh D. Organoids-on-a-chip. Science. 2019;364(6444):960-965. doi: 10.1126/science.aaw7894
- Hofer M, Lutolf MP. Engineering organoids. Nat Rev Mater. 2021;6(5):402-420. doi: 10.1038/s41578-021-00279-y
- Workman MJ, Gleeson JP, Troisi EJ, et al. Enhanced utilization of induced pluripotent stem cell–derived human intestinal organoids using microengineered chips. Cell Mol Gastroenterol Hepatol. 2018;5(4):669-677.e2. doi: 10.1016/j.jcmgh.2017.12.008
- Kasendra M, Tovaglieri A, Sontheimer-Phelps A, et al. Development of a primary human small intestine-on-a-chip using biopsy-derived organoids. Sci Rep. 2018; 8(1):2871. doi: 10.1038/s41598-018-21201-7
- Kasendra M, Luc R, Yin J, et al. Duodenum intestine-chip for preclinical drug assessment in a human relevant model. eLife. 2020;9:e50135. doi: 10.7554/eLife.50135
- Shin W, Hinojosa CD, Ingber DE, Kim HJ. Human intestinal morphogenesis controlled by transepithelial morphogen gradient and flow-dependent physical cues in a microengineered gut-on-a-chip. iScience. 2019;15: 391-406. doi: 10.1016/j.isci.2019.04.037
- Jalili-Firoozinezhad S, Gazzaniga FS, Calamari EL, et al. A complex human gut microbiome cultured in an anaerobic intestine-on-a-chip. Nat Biomed Eng. 2019;3(7):520-531. doi: 10.1038/s41551-019-0397-0
- Madden LR, Nguyen TV, Garcia-Mojica S, et al. Bioprinted 3D primary human intestinal tissues model aspects of native physiology and ADME/Tox functions. iScience. 2018;2: 156-167. doi: 10.1016/j.isci.2018.03.015
- Torras N, Zabalo J, Abril E, Carré A, García-Díaz M, Martínez E. A bioprinted 3D gut model with crypt-villus structures to mimic the intestinal epithelial-stromal microenvironment. Biomater Adv. 2023;153:213534. doi: 10.1016/j.bioadv.2023.213534
- Han H, Park Y, Choi Y-M, et al. A bioprinted tubular intestine model using a colon-specific extracellular matrix bioink. Adv Healthc Mater. 2022;11(2):2101768. doi: 10.1002/adhm.202101768
- Lampart FL, Iber D, Doumpas N. Organoids in high-throughput and high-content screenings. Front Chem Eng. 2023;5:1120348. doi: 10.3389/fceng.2023.1120348
- Szymański P, Markowicz M, Mikiciuk-Olasik E. Adaptation of high-throughput screening in drug discovery—toxicological screening tests. Int J Mol Sci. 2012;13(1):427-452. doi: 10.3390/ijms13010427
- Bajorath J. Integration of virtual and high-throughput screening. Nat Rev Drug Discov. 2002;1(11):882-894. doi: 10.1038/nrd941
- Probst C, Schneider S, Loskill P. High-throughput organ-on-a-chip systems: current status and remaining challenges. Curr Opin Biomed Eng. 2018;6:33-41. doi: 10.1016/j.cobme.2018.02.004
- Beaurivage C, Naumovska E, Chang YX, et al. Development of a gut-on-a-chip model for high throughput disease modeling and drug discovery. Int J Mol Sci. 2019;20(22):5661. doi: 10.3390/ijms20225661
- Azizgolshani H, Coppeta JR, Vedula EM, et al. High-throughput organ-on-chip platform with integrated programmable fluid flow and real-time sensing for complex tissue models in drug development workflows. Lab Chip. 2021;21(8):1454-1474. doi: 10.1039/D1LC00067E
- Mazrouei R, Velasco V, Esfandyarpour R. 3D-bioprinted all-inclusive bioanalytical platforms for cell studies. Sci Rep. 2020;10(1):14669. doi: 10.1038/s41598-020-71452-6
- Lind JU, Busbee TA, Valentine AD, et al. Instrumented cardiac microphysiological devices via multimaterial three-dimensional printing. Nat Mater. 2017;16(3):303-308. doi: 10.1038/nmat4782
- Trampe E, Koren K, Akkineni AR, et al. Functionalized bioink with optical sensor nanoparticles for O2 imaging in 3D-bioprinted constructs. Adv Funct Mater. 2018;28(45):1804411. doi: 10.1002/adfm.201804411
- Vancamelbeke M, Vermeire S. The intestinal barrier: a fundamental role in health and disease. Expert Rev Gastroenterol Hepatol. 2017;11(9):821-834. doi: 10.1080/17474124.2017.1343143
- Lee Y, Kim M-H, Alves DR, et al. Gut–kidney axis on chip for studying effects of antibiotics on risk of hemolytic uremic syndrome by shiga toxin-producing escherichia coli. Toxins. 2021;13(11):775. doi: 10.3390/toxins13110775
- Skardal A, Murphy SV, Devarasetty M, et al. Multi-tissue interactions in an integrated three-tissue organ-on-a-chip platform. Sci Rep. 2017;7(1):8837. doi: 10.1038/s41598-017-08879-x
- Goldstein Y, Spitz S, Turjeman K, et al. Breaking the third wall: implementing 3D-printing techniques to expand the complexity and abilities of multi-organ-on-a-chip devices. Micromachines. 2021;12(6):627. doi: 10.3390/mi12060627
- Díaz Lantada A, Pfleging W, Besser H, et al. Research on the methods for the mass production of multi-scale organs-on-chips. Polymers. 2018;10(11):1238. doi: 10.3390/polym10111238
- Picollet-D’hahan N, Zuchowska A, Lemeunier I, Le Gac S. Multiorgan-on-a-chip: a systemic approach to model and decipher inter-organ communication. Trends Biotechnol. 2021;39:788-810. doi: 10.1016/j.tibtech.2020.11.014
- Lee H, Cho D-W. One-step fabrication of an organ-on-a-chip with spatial heterogeneity using a 3D bioprinting technology. Lab Chip. 2016;16(14):2618-2625. doi: 10.1039/C6LC00450D
- Zandrini T, Florczak S, Levato R, Ovsianikov A. Breaking the resolution limits of 3D bioprinting: future opportunities and present challenges. Trends Biotechnol. 2023;41(5): 604-614. doi: 10.1016/j.tibtech.2022.10.009
- Tian C-m, Yang M-f, Xu H-m, et al. Stem cell-derived intestinal organoids: a novel modality for IBD. Cell Death Discov. 2023;9(1):255. doi: 10.1038/s41420-023-01556-1
- van Berlo D, Nguyen VVT, Gkouzioti V, Leineweber K, Verhaar MC, van Balkom BWM. Stem cells, organoids, and organ-on-a-chip models for personalized in vitro drug testing. Curr Opin Toxicol. 2021;28:7-14. doi: 10.1016/j.cotox.2021.08.006
- Kim J, Koo B-K, Knoblich JA. Human organoids: model systems for human biology and medicine. Nat Rev Mol Cell Biol. 2020;21(10):571-584. doi: 10.1038/s41580-020-0259-3
- Piergiovanni M, Leite SB, Corvi R, Whelana M. Standardisation needs for organ on chip devices. Lab Chip. 2021;21(15):2857-2868. doi: 10.1039/D1LC00241D
- Kesti M, Fisch P, Pensalfini M, Mazza E, Zenobi-Wong M. Guidelines for standardization of bioprinting: a systematic study of process parameters and their effect on bioprinted structures. BioNanoMaterials. 2016;17(3-4):193-204. doi: 10.1515/bnm-2016-0004
- Ren Y, Yang X, Ma Z, et al. Developments and opportunities for 3D bioprinted organoids. Int J Bioprint. 2021; 7(3):364. doi: 10.18063/ijb.v7i3.364
- Ashammakhi N, Ahadian S, Xu C, et al. Bioinks and bioprinting technologies to make heterogeneous and biomimetic tissue constructs. Mater Today Bio. 2019;1:100008. doi: 10.1016/j.mtbio.2019.100008
- Mazzocchi A, Soker S, Skardal A. 3D bioprinting for high-throughput screening: drug screening, disease modeling, and precision medicine applications. Appl Phys Rev. 2019;6(1):011302. doi: 10.1063/1.5056188
- Kim W, Kim G. Intestinal villi model with blood capillaries fabricated using collagen-based bioink and dual-cell-printing process. ACS Appl Mater Interfaces. 2018;10(48): 41185-41196. doi: 10.1021/acsami.8b17410